Nitrogenous Wastes and Temperature Regulation in Vertebrates PDF
Document Details
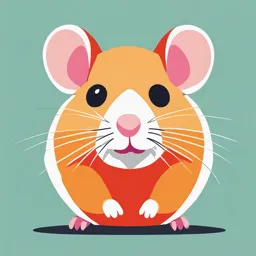
Uploaded by ValiantEmerald
Tags
Summary
This document discusses how different vertebrate species excrete nitrogenous wastes like ammonia, urea, and uric acid, and how these excretion methods relate to water availability. It explains how temperature affects the rates of biological processes. It uses examples from different types of animals and relates to their ability to maintain a temperature difference from their environment, such as water.
Full Transcript
Nitrogenous Wastes Differences in how nitrogenous wastes are excreted are partly a matter of the availability of water and partly the result of differences among phylogenetic lineages. Most vertebrates eliminate nitrogen as ammonia, as urea, or as uric acid. Excreting nitrogenous wastes primarily as a...
Nitrogenous Wastes Differences in how nitrogenous wastes are excreted are partly a matter of the availability of water and partly the result of differences among phylogenetic lineages. Most vertebrates eliminate nitrogen as ammonia, as urea, or as uric acid. Excreting nitrogenous wastes primarily as ammonia is called ammonotelism, excretion primarily as urea is ureotelism, and excretion primarily as uric acid is uricotelism. Most vertebrates excrete a mixture of nitrogenous waste products, with different proportions of the three compounds. Ammonotely Bony fishes are primarily ammonotelic and excrete ammonia through the skin and gills as well as in urine. Because ammonia is produced by deamination of proteins, no metabolic energy is needed to produce it. Ureotely Mammals are primarily ureotelic, although they excrete some nitrogenous wastes as ammonia and uric acid. Normal values for humans, for example, are 82 percent urea and 2 percent each ammonia and uric acid. The remaining 14 percent of nitrogenous waste is composed of other nitrogen-containing compounds, primarily amino acids and creatine. Urea is synthesized from ammonia in a cellular enzymatic process called the urea cycle. Urea synthesis requires more energy than does ammonia production, but urea is less toxic than ammonia. Because urea is not very toxic, it can be concentrated in urine, thus conserving water. Uricotely Reptiles, including birds, are primarily urico- telic, but here again all three of the major nitrogenous compounds are present. The pathway for synthesis of uric acid is complex and requires more energy than synthesis of urea. The advantage of uric acid lies in its low solubility: it precipitates from the urine and is excreted as a semisolid paste. The water that was released when the uric acid precipitated is reabsorbed, so uricotely is an excellent method of excreting nitrogenous wastes while conserving water. Some species of reptiles change the proportions of the three compounds depending on the water balance of the animal, excreting more ammonia and urea when water is plentiful and shifting toward uric acid when it is necessary to conserve water. 4.5 Responses to Temperature Vertebrates occupy habitats that extend from cold polar latitudes to hot deserts. To appreciate their adaptability, we must consider how temperature affects an aquatic vertebrate such as a fish or amphibian that has little capacity to maintain a difference 90 CHAPTER 4 Living in Water between its body temperature and the temperature of the water around it. Organisms have been called bags of chemicals catalyzed by enzymes. This description emphasizes that living systems are subject to the laws of physics and chemistry just as nonliving systems are. Because temperature influences the rates at which chemical reactions proceed, temperature vitally affects the life processes of organisms. The rates of most chemical reactions increase or decrease when the temperature changes. The ratio of the rate at one temperature and the rate at a temperature 10°C higher is called Q10. Because Q10 is the ratio of the rates, a Q10 of 1.0 means that the rate stays the same, a Q10 greater than 1 means the rate increases, and a Q10 less than 1.0 indicates the rate decreases (Figure 4–13). Q10 can be used to describe the effect of temperature on biological processes at all levels of biological organization from whole animals down to molecules. The standard metabolic rate (SMR) of an organism is the minimum rate of oxygen consumption needed to sustain life. That is, the SMR includes the costs of ventilating the lungs or gills, of pumping blood through the circulatory system, of transporting ions across membranes, and of all the other activities necessary to maintain the integrity of an organism. The SMR does not include the costs of activities like locomotion or growth. The SMR is temperature sensitive, and that means the energy cost of living is affected by changes in body temperature. If the SMR of a fish is 2 joules per minute at 10°C and the Q10 is 2, the fish will use 4 joules per minute at 20°C and 8 joules per minute at 30°C. That increase in energy use translates to a corresponding increase in the amount of food the fish must eat. Controlling Body Temperature Because the rates of many biological processes are affected by temperature, it would be advantageous for any animal to be able to control its body temperature. However, the high heat capacity and heat conductivity of water make it difficult for most fishes or aquatic amphibians to maintain a temperature difference between their bodies and the surrounding water. Air has both a lower heat capacity and a lower heat conductivity than water, however, and the body temperatures of most terrestrial vertebrates are at least partly independent of the air temperature. Many terrestrial vertebrates and some aquatic vertebrates do have body temperatures substantially above the temperature of the air or water around them. Maintaining those temperature differences requires thermoregulatory mechanisms, and these are well developed among vertebrates. Q10 = 1.0 (a) Rate 100 90 80 70 60 50 Q10 = 2.0 Q10 = 0.5 5 10 15 20 25 30 35 Temperature (°C) 40 45 Swimming speed (cm/sec) (b) 60 50 40 30 20 10 15 20 25 30 Temperature (°C) 35 40 (c) 0.90 Activity index 0.80 0.70 monly described as poikilotherms (Greek poikilo = variable and therm = heat) and homeotherms (Greek homeo = the same) through the middle of the twentieth century. Poikilotherms were animals with variable body temperatures, and homeotherms were animals with stable body temperatures. Fishes, amphibians, and reptiles were called poikilotherms, and birds and mammals were homeotherms. This terminology has become less appropriate as our knowledge of the temperature-regulating capacities of animals has become more sophisticated. Poikilothermy and homeothermy describe the variability of body temperature, and these terms cannot readily be applied to groups of animals. For example, some mammals allow their body temperatures to drop 20°C or more from their normal levels at night and in the winter, whereas many fishes live in water that changes temperature less than 2°C in an entire year. That example presents the contradictory situation of a homeotherm that experiences 10 times as much variation in body temperature as a poikilotherm. 0.60 Ectothermy and Endothermy Complications like these 0.50 make it very hard to use the words homeotherm and poikilotherm rigorously. Most biologists concerned with temperature regulation prefer the terms ectotherm and endotherm. These terms are not synonymous with the earlier words because, instead of referring to the variability of body temperature, they refer to the sources of energy used in thermoregulation. Ectotherms (Greek ecto = outside) gain their heat largely from external sources—by basking in the sun, for example, or by resting on a warm rock. Endotherms (Greek endo = inside) largely depend on metabolic production of heat to raise their body temperatures. The source of heat used to maintain body temperature is the major difference between ectotherms and endotherms because their body temperatures are quite similar. Terrestrial ectotherms (like lizards and turtles) and endotherms (like birds and mammals) have activity temperatures between 30°C and 40°C. Endothermy and ectothermy are not mutually exclusive mechanisms of temperature regulation, and many animals use them in combination. In general, birds and mammals are primarily endothermal, but some species make extensive use of external sources of heat. For example, roadrunners are predatory birds living in the deserts of the southwestern United States and adjacent Mexico. On cold nights, roadrunners allow their body temperatures to fall from the normal level of 38°C or 39°C down to 35°C or lower. In the mornings they 0.40 10 15 20 25 Temperature (°C) 30 35 (d) 0.70 Reaction rate Poikilothermy and Homeothermy Vertebrates were com- 0.60 0.50 0.40 0.30 0.20 25 30 35 40 45 Temperature (°C) 50 Figure 4–13 Examples of the effect of temperature on organisms. (a) A hypothetical reaction for which the rate initially increases and then falls as temperature rises. Between 10°C and 20°C the rate doubles from 50 to 100, which is a Q10 of 2.0. The rate does not change between 20°C and 30°C, so the Q10 for this temperature range is 1.0. The rate drops from 100 units at 30°C to 50 units at 40°C, so the Q10 is 0.5. (b) The maximum swimming speed of a goldfish increases up to about 30°C and then falls. (c) Spontaneous activity by a goldfish peaks at around 20°C and falls as the temperature increases. (d) Activity of the enzyme lactic dehydrogenase from a lungfish increases slowly from 25°C to 35°C (Q10 about 1.2), more steeply between 35°C and its maximum at 40°C (Q10 about 1.4), and declines rapidly between 40°C and 50°C (Q10 about 0.4). Responses to Temperature 91 bask in the sun, raising the feathers on their backs to expose an area of black skin. Calculations indicate that a roadrunner can save 132 joules per hour by using solar energy instead of metabolism to raise its body temperature. Deviations from general patterns of temperature regulation go the other way as well. Snakes are normally ectothermal, but the females of several species of pythons coil around their eggs and produce heat by rhythmic contraction of their trunk muscles. The rate of contraction increases as air temperature falls, and a female Indian python is able to maintain her eggs close to 30°C at air temperatures as low as 23°C. This heat production entails a substantial increase in the python’s metabolic rate—at 23°C, a female python uses about 20 times as much energy when she is brooding as she does normally. Thus, generalizations about the body temperatures and thermoregulatory capacities of vertebrates must be made cautiously, and the actual mechanisms used to regulate body temperature must be studied carefully. Regional Heterothermy Regulation of body tempera- ture is not an all-or-nothing phenomenon for vertebrates. Regional heterothermy is a general term used to refer to different temperatures in different parts of an animal’s body. Dramatic examples of regional heterothermy are found in several fishes that maintain some parts of their bodies at temperatures 15°C warmer than the water in which they are swimming. That’s a remarkable accomplishment for a fish because each time the blood passes through the gills it comes into temperature equilibrium with the water. Thus, to raise its body temperature by using endothermal heat production, a fish must limit the loss of heat to the water via the gills. Warm Muscles The mechanism used to retain heat is a countercurrent system of blood flow in retia mirabilia. As cold arterial blood from the gills enters the warm part of the body, it flows through a rete and is warmed by heat from the warm venous blood that is leaving the tissue. This arrangement is found in some sharks, especially species in the family Lamnidae (including the mako, great white shark, and porbeagle), which have retia mirabilia in the trunk. These retia retain the heat produced by activity of the swimming muscles, with the result that those muscles are kept 5°C to 10°C warmer than water temperature. Scombroid fishes, a group of teleosts that includes the mackerels, tunas, and billfishes (i.e., the swordfish and spearfish as well as the sailfishes and marlins), have also evolved endothermal heat production. Tunas have an arrangement of retia that retains the heat produced 92 CHAPTER 4 Living in Water by myoglobin-rich swimming muscles located close to the vertebral column (Figure 4–14). The temperature of these muscles is held near 30°C at water temperatures from 7°C to 23°C. Additional heat exchangers are found in the brains and eyes of tunas and sharks, and these organs are warmer than water temperature but somewhat cooler than the swimming muscles. Hot Eyes The billfishes have a somewhat different arrangement in which only the brain and eyes are warmed, and the source of heat is a muscle that has changed its function from contraction to heat production. The superior rectus eye muscle of these billfishes has been extensively modified. Mitochondria occupy more than 60 percent of the cell volume, and changes in cell structure and biochemistry result in the release of heat by the calcium-cycling mechanism that is usually associated with contraction of muscles. A related scombroid, the butterfly mackerel, has a thermogenic organ with the same structural and biochemical characteristics found in billfishes, but in the mackerel it is the lateral rectus eye muscle that has been modified. An analysis of the phylogenetic relationships of scombroid fishes by Barbara Block and her colleagues suggests that endothermal heat production has arisen independently three times in the lineage—once in the common ancestor of the living billfishes (by modification of the superior rectus eye muscle), once in the butterfly mackerel lineage (by modification of the lateral rectus eye muscle), and a third time in the common ancestor of tunas and bonitos (by development of countercurrent heat exchangers in muscle, viscera, and brain, and development of red muscle along the horizontal septum of the body). The ability of these fishes to keep parts of the body warm may allow them to venture into cold water that would otherwise interfere with body functions. Block has pointed out that modification of the eye muscles and the capacity for heat production among scombroids is related to the temperature of the water in which they swim and capture prey. The metabolic capacity of the heater cells of the butterfly mackerel, which is the species that occurs in the coldest water, is the highest of all vertebrates. Swordfishes, which dive to great depths and spend several hours in water temperatures of 10°C or lower, have better-developed heater organs than do marlins, sailfishes, and spearfishes, which spend less time in cold water. Marine Mammals The temperature equilibration of blood and water that occurs in the gills is the primary obstacle to whole-body endothermy for fish. Countercurrent systems allow them to keep critical parts of 21.3° 23.3° 25.3° 27.3° 29.3° 31.4° Rete region 27.3° 25.3° Water 19.3° (a) 23.3° 21.3° (b) 35 Muscle temperature (°C) Tmuscle 30 er Tm = 25.3 + 0.24 Tw 25 = at Tw 20 5 (c) 10 15 20 25 30 Water temperature (°C) Figure 4–14 Details of body temperature regulation by the bluefin tuna. (a) The red muscle and retia are located adjacent to the vertebral column. (b) Cross-sectional views showing the temperature gradient between the core (at 31.4°C) and water temperature (19.3°C). (c) Core muscle temperatures of bluefins compared to water temperature. their bodies warm, but other parts are at water temperature. Air-breathing aquatic tetrapods avoid that problem because they have lungs instead of gills. Mammals are endotherms, and their high metabolic rates combined with the muscular metabolism that accompanies activity release heat that warms the body. For terrestrial mammals, the furry body covering (the pelage) traps metabolic heat in the dead air spaces between hairs and reduces the movement of heat out of the body, just as the air trapped between strands of fiberglass insulations reduces the movement of heat through the wall of a building. A furry body covering Responses to Temperature 93 works well in air but not as well in water. If water displaces the air between the hairs, the coat loses its insulative properties. Many semiaquatic mammals, such as otters and beavers, have water-repellent coats, but they must emerge onto land frequently and groom their coats to keep them water-repellent. The most specialized marine mammals, cetaceans (whales and porpoises) and pinnipeds (seals, sea lions, and walruses), use blubber (a layer of fat beneath the skin) rather than fur for insulation. Blubber is an extremely effective insulator, so good that some seals risk death by overheating if they undertake prolonged strenuous activity on land. Even in water, strenuous activity can lead to overheating. Aquatic mammals have countercurrent exchange systems in their flippers that allow them to retain heat in the body or release it to the ocean. The venous blood returning from the flipper is cold because it has passed through a flat structure with a large surface area that is in contact with the ocean water. Those are ideal conditions for heat exchange, and the blood that leaves the flippers is very close to water temperature. When a marine mammal needs to retain heat in the body, blood returning from the flippers flows through veins that are closely associated with the arteries that carry blood from the body to the flippers. Cold venous blood is heated by warm arterial blood flowing out from the core of the body. By the time the venous blood reaches the body, it is nearly back to body temperature. When a bout of rapid swimming produces enough heat to increase body temperature above normal, the animal changes the route that venous blood takes as it returns from the flipper. Instead of flowing through veins that are pressed closely against the walls of the arteries, the returning blood is shunted through vessels distant from the arteries. The arterial blood is still hot when it reaches the flipper, and that heat is dissipated into the water, cooling the animal. 4.6 Body Size and Surface/ Volume Ratio Body size is an extremely important element in the exchange occurring between an organism and its environment. For objects of the same shape, volume increases as the cube of linear dimensions, whereas surface area increases only as the square of linear dimensions. Consider a cube that is 1 centimeter (cm) on each side. Each face of the cube is 1 cm2 (that is, 1 cm. 1 cm), and a cube has six faces (numbered 1 through 6 if the cube comes from a set of dice), so the 94 CHAPTER 4 Living in Water total surface area is 6 cm2. The volume of that cube is one cubic centimeter (1 cm. 1 cm. 1 cm), and the ratio of surface to volume is 6 cm2/1 cm3 (Figure 4–15a). If we increase the linear dimensions of the cube so that a side becomes 5 centimeters long, each face has a surface area of 25 cm2 (that is, 5 cm. 5 cm), and the total surface area is 150 cm2 (6. 25 cm2). The volume of this larger cube is 125 cm3 (that is, 5 cm. 5 cm. 5 cm), and the ratio of surface to volume is 150 cm2/125 cm3, which reduces to 1.2 cm2/1 cm3. Thus the larger cube has only one-fifth as much surface area per unit volume as the smaller cube. A cube 10 centimeters on a side has a surface/volume ratio of 600 cm2/1000 cm3, which is 0.6 cm2/1 cm3—only one-tenth the surface/ volume ratio of the smallest cube. The pattern emerging from these calculations is shown in Figure 4–15b: as an object gets larger, it has progressively less surface area in relation to its volume. Exchange between an animal and its environment occurs through its body surface, and large animals have proportionally less area for exchange in relation to the volume (or mass) of their bodies than small animals do. The biological significance of that relationship lies in the conclusion that bigger species exchange energy with the environment less rapidly than smaller species, merely because of the difference in surface/ volume ratios. Gigantothermy Simply being big gives an animal some independence of external temperature because heat cannot flow rapidly into or out of a large body through its relatively small surface. If an animal is large enough, its body temperature will be stable simply because it’s so big; this is a form of thermoregulation called gigantothermy. The enormous dinosaurs that lived in the Jurassic and Cretaceous periods would have had very stable body temperatures just because they were so large. It would take many days for a huge dinosaur to warm or cool as its environment changed temperature. Even elephants (which are only one-twentieth the size of the largest dinosaurs) are big enough to feel the consequences of surface/volume ratio in body-temperature regulation. Elephants can easily overheat when they are active. When that happens, they dump heat by sending large volumes of blood flowing through their ears and waving them to promote cooling. Being big makes temperature regulation in water easier, as leatherback sea turtles dramatically illustrate. Leatherbacks (Dermochelys coriacea) are the largest living turtles, reaching adult body masses of 850 kilograms or more. They are also the most specialized sea turtles, having lost the bony shell that covers most Area of side = 1 cm2 Total surface area = 6 cm2 Volume = 1 cm3 Surface/volume ratio = 6 cm2/1 cm3 1 cm 1 cm 1 cm 5 cm Area of side = 25 cm2 Total surface area = 150 cm2 Volume = 125 cm3 Surface/volume ratio = 6 cm2/1 cm3 5 cm 5 cm 10 cm Area of side = 100 cm2 Total surface area = 600 cm2 10 cm Volume = 1000 cm3 Surface/volume ratio = 0.6 cm2/1 cm3 10 cm (a) Figure 4–15 Linear dimensions, surface area, and volume. A cube illustrates how 6 Surface area Volume Surface area/volume 800 5 4 600 3 400 2 200 1 0 0 0 1 2 3 4 5 6 7 Length of side of cube (cm) (b) 8 9 10 Surface/volume ratio Area (cm2) or volume (cm3) 1000 the surface/volume ratio changes with size. (a) As the length of the side of the cube is increased from 1 cm to 10 cm, the total surface area of the cube increases as the square of that length, whereas the volume of the cube increases as the cube of the length of a side. (b) Because volume increases more rapidly than surface area, the surface/volume ratio of the cube decreases as the size of the cube increases. Functionally this means that as an object becomes larger, it has less surface area relative to its volume. Thus the rate of exchange with the environment decreases. For example, if you take two cubes—one that is 1 cm on a side and the other 10 cm on a side—and then heat them to the same temperature and put them side by side on a table, the small cube will cool to room temperature faster than the large cube. Body Size and Surface/Volume Ratio 95