Transcription and Translation 2024 PDF
Document Details
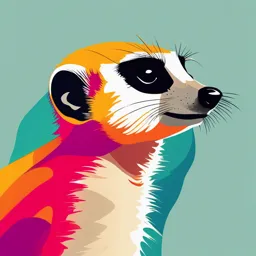
Uploaded by ToughestAntagonist
University of Sunderland
2024
Dr Mark Carlile
Tags
Summary
These lecture notes focus on the molecular biology of DNA transcription in prokaryotes. They provide an overview of the basic mechanisms used for gene transcription in such organisms.
Full Transcript
Reading the DNA Sequence: Transcription Dr Mark Carlile Dale 106 [email protected] Dr Mark Carlile : Transcription & Translation 1 Overview of my lectures on PHA111 All of my lectures are broadly focused on molecular biology: Wk 27: Nucleic acid biochemistry Wk 28: DNA replication Wk 28:...
Reading the DNA Sequence: Transcription Dr Mark Carlile Dale 106 [email protected] Dr Mark Carlile : Transcription & Translation 1 Overview of my lectures on PHA111 All of my lectures are broadly focused on molecular biology: Wk 27: Nucleic acid biochemistry Wk 28: DNA replication Wk 28: Transcription Wk 28: Translation (protein synthesis) Dr Mark Carlile | Nucleic acid biochemistry 2 Recommended textbook: Dr Mark Carlile : DNA Replication 3 What have we covered so far …. The biochemistry of nucleic acids DNA replication Now we will briefly cover ….. Transcription : reading the DNA sequence and making RNA from the information Translation : making proteins from genetic information Dr Mark Carlile : Transcription & Translation 4 The Central Dogma of Molecular Biology Cellular function Lecture 1 Lecture 2 Lecture 3 Look at how the genetic material is controllably replicated prior to cell division Look at the functional genome and its generation (RNA) Look at how the genome is translated into working parts Dr Mark Carlile : Transcription & Translation 5 The Central Dogma of Molecular Biology Transcription is the enzymatic synthesis of RNA from a DNA template and forms the first step in gene expression Generation of a messenger RNA (mRNA) Translation is the enzymatic synthesis of protein from an transcribed gene sequence into a functional RNA molecule mRNA Dr Mark Carlile : Transcription & Translation 6 Transcription 186 Section K – Transcription in prokaryotes Transcription is catalysed by an RNA polymerase enzyme complex which requires: mRNA synthesis a dsDNA template Ribonucleotides (ATP, GTP, CTP and UTP) late Temp A DN d stran RNA synthesis is in the 5’ to 3’ direction Both strands of the DNA can be used: Sense strand – carries the gene sequence that will be copied into an RNA molecule that will be later translated into protein Antisense strand – used as a template to generate a copy of the sense strand through complementary base airing. Also carries the gene sequence for RNA molecules that are usually non-coding and function as structural or regulatory molecules mRNA Fig. 1. Formation of the phosphodiester bond in transcription. may also be called the template strand since it is used as the template to which ribonucleotides base-pair for the synthesis of the RNA. Initiation Dr Mark Carlile : Transcription & Initiation of transcription involves the binding of an RNA polymerase to the dsDNA. RNA polymerases are usually multisubunit enzymes. They bind to the dsDNA and initiate transcription at sites called promoters (Fig. 2). Promoters are sequences of DNA at the start of genes, that is to the 5!-side (upstream) of the coding region. Sequence elements of promoters are often conserved between Translation different genes. Differences between the promoters of different genes give rise to differing efficiencies of transcription initiation and are involved in their regu- 7 RNA polymerases In prokaryotes there is a single RNA polymerase In eukaryotes, there are 3 RNA polymerase enzymes – I, II and III Each polymerase is responsible for transcribing different RNAs Polymerase I transcribes most rRNA (ribosomal RNAs) Polymerase II is responsible for the transcription of all protein-coding genes Polymerase III transcribes tRNAs (transfer RNAs) Dr Mark Carlile : Transcription & Translation 8 Transcription Initiation: Initiation of transcription involves the binding of RNA polymerase complex to the dsDNA The polymerase binds to a specific regulatory sequence(s) on the DNA that is upstream of the gene to be transcribed: Promoter sequences Elements of promoters are conserved between species Promoters can be switched on weakly or strongly based on the sequence elements present and if they are stimulated by bound proteins Gene K1 – Basic principles of transcription Promoter Gene Coding sequence 187 Fig. 2. Structure of a typical transcription unit showing promoter and terminator sequences, and the RNA product. Dr Mark Carlile : Transcription & Translation 9 Transcription Initiation: The dsDNA needs to be locally unwound for the RNA polymerase to access the promoter sequence The polymerase starts to synthesize the messenger RNA (mRNA) at specific nucleotide called the start site or initiation site (position +1 of the gene sequence) Gene K1 – Basic principles of transcription Promoter Gene Coding sequence 187 initiation site Fig. 2. Structure of a typical promoter and terminator sequences, RNA polymerase binding site transcription unit showingTranscription and the RNA product. Elongation The RNA polymerase covalently adds ribonucleotides to the 3!-end of the growing RNA chain (Fig. 1). The polymerase therefore extends the growing Dr Mark Carlile : Transcription & Translation RNA chain in a 5!→3! direction. This occurs while the enzyme itself moves in a 3!→5! direction along the antisense DNA strand (template). As the enzyme 10 Transcription Elongation: The RNA polymerase covalently adds ribonucleotides to the 3’-end of the growing mRNA molecule in the 5’ to 3’ direction The polymerase is moving in the 3’ to 5’ direction along the antisense/template DNA strand The polymerase locally unwinds the DNA duplex ahead of its RNA synthesis activity. The helix is reformed behind the polymerase enzyme. In E.coli the enzyme synthesizes around 40 bases per second at 37OC Gene K1 – Basic principles of transcription Promoter Gene Coding sequence 187 Direction of RNA synthesis Fig. 2. Structure of a typical transcription unit showing promoter and terminator sequences, and the RNA product. Dr Mark Carlile : Transcription & Translation 11 E.coli RNA polymerase 1268 Chapter 31. Transcription (which is on the DNA’s nontemplate/sense strand). The E.coli polymerasemoter consists of at least five “known” Consequently, conformational tension builds up as the RNA template/antisense strand is pulled through the RNAP’s subunits active site, a process called scrunching because the resulting increased size of the transcription bubble in the downstream direction must somehow be accommodated within the RNAP. In abortive initiation, the RNAP fails to escape Two α-subunits: required for holoenzyme assembly. the promoter and instead relieves the conformational tenOne β-subunit: Catalytic centre of the and keyRNA for initiation sion by releasing theenzyme newly synthesized fragment, thereby letting the transcription bubble relax to its normal and elongation size. The RNAP then reinitiates transcription from the !1 2+ ions One β’-subunit: binds position. two ZnIn to initiation, help catalyse joining successful the strainthe eventually pro-of vides sufficient energy to strip the promoter from the ribonucleotides RNAP, which then commences the processive (continuous) One ω-subunit: stabilises the assembled holoenzyme transcription of the template. This process requires the dissociation of the " factor from the core–DNA–RNA One -subunit: responsible for promoter recognition complex to form the elongation complex, although recent experiments indicate that this process often occurs stochastically (randomly) over several nucleotide additions. The " factor can then join with another core to form a new initiation complex as was demonstrated by a burst of RNA synthesis on addition of core enzyme to a transcribing reaction mixture that initially contained only holoenzyme. In the complete holoenzyme there are: DNA σ Figure 31-11 X-ray structure of Tth core RNAP in complex with a 23-nt template DNA, a 14-nt nontemplate DNA, and a 16-nt RNA. The protein is drawn in ribbon form with its two $ subunits yellow and green, its # subunit cyan, its #¿ subunit pink, and its & subunit gray. The bound Mg2! and Zn2! ions are represented by red and orange spheres, respectively. The DNA and RNA are shown in ladder form with template DNA green, nontemplate DNA blue, and RNA red. Note that residues 208 to 390 of the #¿ subunit, which extend from the tip of its pincer, are disordered and hence not visible, as are the 86-residue C-terminal domains of both $ subunits. [Based on an X-ray structure by Dmitry Vassylyev, University of Alabama at Birmingham. PDBid 5O5I.] α ββ’ωσ a. Bacterial RNAP Has a Highly So the complete holoenzyme is: Complex Structure 2 The X-ray structure of E. coli RNAP has not been determined. However, Seth Darst and Dmitry Vassylyev independently determined the X-ray structures of the closely similar Thermus aquaticus (Taq) and Thermus thermophilus (Tth) RNAP core enzymes and holoenzymes. The structure of the Tth core enzyme in complex with DNA and RNA, in agreement with EM studies of E. coli RNAP, has the overall shape of a crab claw whose two “pincers” formed by the # and #¿ Dr Markare Carlile : Transcription & Translation subunits (Fig. 31-11). The protein is !150 Å long (parallel to The enzyme that travels along the DNA is called the core enzyme and contains: α2ββ’ω 12 E.coli σ70 factor Section 31-2. RNA Polymerase The E.coli polymerase binds to sequences within the promoter region upstream of the initiation site 1267 Different promoters are Operon recognised by different factors/subunits – the most commonInitiation σ factor in E.coli is the σ70 !35σregion !10 region (Pribnow box) site (+1) factor C C A GG C T T T A C A C T T T A T G C T T C C GG C T C G T A T G T T G T G T GG A A T T G T G A G C GG lac Since the transcription start siteACisCC CAdenoted by position +1 the promoter sequence is denoted by a negative number T C G A A T GG C G C A A A A C C T T T C G C GG T A T GG C A T G A T A G C G C C C GG A A G A G A G T C lacI since they are upstream galP2 (before)Athe site T T Tinitiation A T T CCA TG T C A C A C T T T T C G C A T C T T T G T T A T G C T A T GG T T A T T T C A T A C C A T The araBAD G G A T C C T A C C T G A C G C T T T T T A T C G C A A C T C T C T A C T G T T T C T C C A T A C C C G T T T T T is between and araC 40 G C C G60 T Gbase A T T Apairs T A G Along C A C T T T T G T T A C G C G T T T T T G T C A T GG C T T T GG T C C C G C T T T G trp A A A T GAGC T G T T GA C A A T T A A T C A T CGA A C T AG T T A A C T AG T A CGC A AG T T C A CG T A bioAhas been T T C Cshown A A A A CG T T T the T T TG T T Gpolymerase T T A A T T C GG T G T AGA C T T G T A A A C C T A A A T C T T T T from -55 to +20 toT Gbind RNA holoenzyme bioB C A T A A T C G A C T T G T A A A C C A A A T T G A A A A G A T T T A GG T T T A C A A G T C T A C A C C G A A T t RNA C Astrongly A CG T A A C A C T T T A C Awith G C G Gthe C G Choloenzyme G T C A T T T GA T A T GA T GCGC C C CGC T T C C CGA T A from -20 to +20 isTyrvery associated rrnD1 C A A A A A A A T A C T T G T G C A A A A A A T T GGG A T C C C T A T A A T G C G C C T C C G T T G A G A C G A A Tefficient T T T T C T Atranscription T T G C GG C C T G C GG A G A A C T C C C T A T A A T G C G C C T C C A T C G A C A C GG up to -40 are rrnE1 requiredC Afor rrnA1 A A A A T A A A T G C T T G A C T C T G T A G C GGG A A GG C G T A T T A T G C A C A C C C C G C G C C G C T G σ70 promoter The region The region Sequences Other important sequences: !35 region Consensus sequence: T T G A C A 69 79 61 56 54 54... 16–19 bp... !10 region T A T A A T 77 76 60 61 56 82... 5–8 bp... Initiation site A C 55 51 G T 48 42 Not Figure 31-10 The sense (nontemplate) sequences of Not the consensus sequence of 298 E. coli promoters with the -35 strand region Pribnow box selected E. coli promoters. A 6-bp region centered around the number below each base indicating its percentage occurrence. Conserved Conserved TTGACA TATAAT !10 position (red shading) and a 6-bp sequence around the !35 The downstream portions of the rrn genes’ UP elements can be Highly conserved Highly conserved region (blue shading) are both conserved. The transcription seen. [After Rosenberg, M. and Court, D., Annu. Rev. Genet. 13, initiation sites ('1), which inRecognition most promoters sequence occur at a single 321–323 (1979). Consensus sequence DNA Unwinding site from Lisser, S. and purine nucleotide, are shaded in green. The bottom row shows Margalit, H., Nucleic Acids Res. 21, 1512 (1993).] Dr Mark Carlile : Transcription & Translation 13 RNA polymerase: Schematic Dr Mark Carlile : Transcription & Translation 14 Overview: E.coli transcription Step 1: Promoter Binding The core polymerase enzyme (i.e. no σ factor) has a non-specific loose affinity for DNA. Association of the σ factor forms the holoenzyme which binds to specific promoter sequences with a 100-fold increase in affinity It is thought that the holoenzyme is always attached to DNA and slides along searching for the promoter sequence -35 and -10 sites This closed complex then sit awaiting stimulation at certain highly expressed or important genes Step 2: DNA unwinding To gain access to the antisense strand the DNA needs to be unwound by the polymerase The topology of the DNA can play a big part in this mechanism Negative supercoiling is used at very active gene promoters to help with the melting of the duplex The topology of the -35 and -10 sites relative to each other may facilitate changes in DNA conformation/topology The initial unwinding of the DNA duplex forms an open complex with the holoenzyme Dr Mark Carlile : Transcription & Translation 15 Overview: E.coli transcription Step 3: Transcription initiation RNA synthesis can initiate without a primer (in contrast to DNA synthesis) Almost all RNA has a purine at its 5’-most end : G is more common than A The polymerase takes in the first two ribonucleotides and forms a phosphodiester bond. The chain is started with a GTP or a ATP The first 9 bases are joined without the enzyme moving – transcription can be aborted during the formation of any one of these bonds In E.coli a fast growth rate sometimes means that the next polymerase holoenzyme is queued awaiting the next initiation so this decision of to transcribe or not to transcribe needs to be taken fast (usually 1-2 seconds) Dr Mark Carlile : Transcription & Translation 16 Overview: E.coli transcription Step 4: mRNA Elongation Once the decision to transcribe is taken the σ-factor forms a ternary (3-components) complex with: Polymerase DNA Nacent RNA This causes the polymerase to move forward and clear the promoter and allows re-initiation by a new holoenzyme if needs-be As the polymerase moves along the DNA a constant area of 17-bp is unwound at any given time at 40 bp per sec A 12 bp hybrid between the template strand and the mRNA is also kept pretty constant – one turn of a RNA-DNA helix The σ-factor is released once the polymerase is moving and is now free to form a new holoenzyme Dr Mark Carlile : Transcription & Translation 17 Overview: E.coli transcription Step 5: Termination Once the polymerase reaches a termination sequence (stop signal) is dissociates from the DNA The most common stop signal is formation of an RNA hairpin generated by self-complementary sequence areas in the mRNA This structure is often GC-rich and so is very stable causing the polymerase to stall Has 4 or more U-residues after the GC-loop which are only weakly associated with the A residues on the template strand The effect of stalling the polymerase and the now weak association between the RNA and DNA template cause a dissociation of the core enzyme Dr Mark Carlile : Transcription & Translation 18 Which genes to transcribe? For most genes transcriptional controls are paramount Transcription of a particular gene is controlled by a regulatory region of DNA near the site of transcription – common in Prokrayotes Some regulatory regions are like simple switches, while others are like a microprocessor and respond to a variety of signal inputs – common in Eukaryotes Gene Regulatory Proteins N1 – Eukaryotic transcription factors Very specific proteins bind to the regulatory region of the DNA to ‘turn on’(and off) the gene The proteins bind to the outside of the DNA by recognising specific sequences Fits into the DNA major groove Recall that the bases are mostly on the inside of the DNA helix, however, the edges of the base pairs are exposed at the surface The ‘exposed edges’ provide opportunities for interactions via hydrogen bonding (H-bond donors and H-bond acceptors) and hydrophobic interactions in both the minor and major grooves It is in the major groove where the patterns for each of the 4 base-pairs is unique – most regulatory proteins bind atSection the Nmajor groove 230 – Regulation of transcription in eukaryotes 60 aa Helix-turn-helix protein motif The zinc finger domain This domain exists in two forms. The C H zinc finger has a loop of 12 (Homeodomain) acids anchored by two cysteine and two histidine residues that tetrahe Fig. 1. The helix–turn–helix core structure. 2 Fits into the DNA major groove 2 co-ordinate a zinc ion (Fig. 2a). This motif folds into a compact stru comprising two !-strands and one "-helix, the latter binding in the major g of DNA (Fig. 2b) The "-helical region contains conserved basic amino which are responsible for interacting with the DNA. This structure is rep nine times in TFIIIA, the RNA Pol III transcription factor (see Topic M3 also present in transcription factor SP1 (three copies). Usually, three or C2H2 zinc fingers are required for DNA binding. A related motif, in whi zinc ion is co-ordinated by four cysteine residues, occurs in over 100 s hormone receptor transcription factors (see Topic N2). These factors con homo- or hetero-dimers, in which each monomer contains two C4 ‘zinc fi motifs (Fig. 2c). The two motifs are now known to fold together into a complex conformation stabilized by zinc, which binds to DNA by the ins of one "-helix from each monomer into successive major grooves, in a m reminiscent of the helix–turn–helix proteins. Zinc-finger motif Gene Regulatory Proteins These regulatory regions of DNA typically contain less than 20 nucleotide base pairs, yet are absolutely fundamental components of genetic switches To date, hundreds of recognition sequences and regulatory proteins have been identified One group of DNA-binding proteins utilises one or more Zn2+ ions – the Zn2+ ions acting to maintain the correct protein folding Slide 20 of 40 MPharm MPH117 DNA – Transcription and Translation An Example The lac operon In E.coli lactose can be used as a carbon source. The enzymes for lactose metabolism are only generated when lactose is available and are controllably expressed from a central control expression cassette called an operon. Three enzymes are expressed when lactose is available: Galactoside permease (lacY) allows lactose uptake from media β-galactosidase (lacZ) converts lactose to: Glucose + Galactose (main reaction) and Allolactose (side reaction) Thiogalactoside transketolase (lacA) – Physiological function in lactose metabolism unknown Expression is of a polycistronic mRNA The lac operon is structured as follows: lacZYA transcription cassette Polycistronic mRNA PlacI lacI Plac Olac lacZ lacY lacA Repressor protein Always ON Always OFF in absence of lactose An Example The lac operon lacZYA transcription cassette Polycistronic mRNA PlacI lacI Plac Olac lacZ lacY lacA lacZ : β-galactrosidase lacY : galactoside permease lacA : thiogalactoside transacetylase Wild-type molecular switch lac repressor (Homotetramer) lac Operator Plac Wild-type molecular switch Binging of lacI to the operator is cooperative Binding of lacI to Olac promotes the binding of RNA polymerase Low level expression of lacZYA -5 14 bp 14 bp + RNA Poly’ase binding Plac lacA lacY lacZ +28 In the absence of lactose the lacI protein is bound to the operator and only a small amount of lacA, lacY and lacZ are generated. LacI also promotes the binding of RNA polymerase When lactose is present it is converted to allolatose which binds to the lacI protein causing it to dissociated from the operator site DNA Once the operator sequence is free a strong level of transcription is induced. + RNA Poly’ase binding Plac Un-induced lacA lacY lacZ + β-galactosidase RNA Poly’ase Plac Induced lacA lacY lacZ RNA Poly’ase Plac Induced RNA Poly’ase lacA mRNA lacY Transcription lacZ Overview: Today we have covered: The basics of DNA transcription in prokaryotes The basic mechanisms used to control gene transcription in prokaryotes Dr Mark Carlile : Transcription & Translation 25