Molecular Cell Biology Week 4 Protein Sorting Mechanisms PDF
Document Details
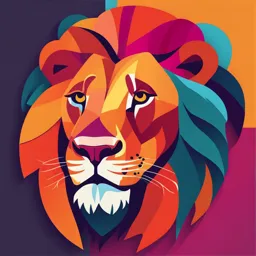
Uploaded by ExceptionalPrimrose
University of Wollongong
Jody Gorman
Tags
Related
- Protein Trafficking 2024 PDF
- BCCB2004 Foundations of Cell Biology Lecture Notes PDF
- BIOL340 Molecular Cell Biology Week 4 Protein Sorting Mechanisms PDF
- Molecular Biology of the Cell Chapter 12 Intracellular Organization and Protein Sorting (Part 2) PDF
- Cell and Molecular Biology Lecture 13 PDF
- Molecular Biology of the Cell Chapter 12 - Intracellular Compartments and Protein Sorting PDF
Summary
This document is lecture notes on protein sorting mechanisms. It discusses protein synthesis, folding, and targeting to various cellular compartments (nucleus, mitochondria, chloroplasts, peroxisomes). It describes the different mechanisms of protein import and export, and presents diagrams to illustrate the processes.
Full Transcript
BIOL340 Molecular Cell Biology Week 4 Protein Sorting Mechanisms Dr Jody Gorman [email protected] Becker’s World of the Cell. 9th Edition. Chpts 12, 16 and 19 Molecular Biology of the Cell, 7th ed. Various chapters 1 Overview Protein synthesis and folding Protein road map Sorting signals Protei...
BIOL340 Molecular Cell Biology Week 4 Protein Sorting Mechanisms Dr Jody Gorman [email protected] Becker’s World of the Cell. 9th Edition. Chpts 12, 16 and 19 Molecular Biology of the Cell, 7th ed. Various chapters 1 Overview Protein synthesis and folding Protein road map Sorting signals Protein trafficking to the nucleus, mitochondria, chloroplasts and peroxisomes Summary ROAD TRIP!!! But which way do we go? 2 Review of protein synthesis 3 Review of protein folding A protein must be converted from a linear chain of amino acids to a specific 3D shape (conformation) to gain its function. Unfolded Folded Cellulose " Function Endoglucanase el lul ose C 4 Proteins required in various cellular compartments Intracellular processes must be separated ¢semipermeable membranes But if most proteins begin their synthesis in the cytosol… How are they accurately targeted to their appropriate compartment? Figure 12.1. Molecular Biology of the Cell 5 So many possible destinations… Will remain in cytosol or be transported to nucleus, mitochondria, chloroplasts or peroxisome Will enter the ER/ER membrane or be transported out of cell or into endomembrane system organelles 6 Lodish et al. (2000) Protein Road Map Three distinct routes of protein importation into organelles ¢ Through nuclear pores ¢Across membranes ¢Via vesicles 7 Protein Road Map Importation can occur →co-translationally OR →post-translationally 8 Figure 12.24. Molecular Biology of the Cell Protein Road Map cytoplasm 1 1 Proteins released into cytosol from free ribosomes Proteins synthesised on ribosomes attached to ER (RER) nucleus mitochondria Vesicular transport plastid endoplasmic reticulum Gated transport Transmembrane transport peroxisome lysosome golgi secretory vesicles endosome cell surface 9 How does a protein know where to go? Its fate depends on sorting signals or “postcode” Info stored in the amino acid sequence Sorting signals can be built into a protein in two ways Signal peptide ¢ER Signal patch ¢Vesicular transport (e.g. Golgi to lysosome) 10 Sorting Signals Sorting Signals Signal sequences were first discovered in proteins imported into the rough ER Two reactions – one with rough microsomes from the ER → size difference = short N-terminal sequence Figure 12.18. Molecular Biology of the Cell 12 Sorting Signals Some typical signal sequences (aka signal peptides) Figure 12.13. Molecular Biology of the Cell 13 Protein Road Map cytoplasm nucleus peroxisome mitochondria plastid endoplasmic reticulum lysosome golgi secretory vesicles endosome cell surface 14 Transportation into the nucleus Figure 16.27. Becker’s World of the Cell 15 Transport of proteins between the nucleus and cytosol Continuous bidirectional transport across channels in the nuclear envelope - selective → Histones, DNA and RNA polymerases, gene regulatory proteins, and RNA processing proteins all require import from the cytosol where they are made → tRNA’s and mRNA’s synthesized n the nucleus are transported into the cytosol where they participate in translation 16 Transport of proteins between the nucleus and cytosol The lumen of the nuclear envelope is continuous with the ER lumen Bidirectional traffic occurs through the nuclear pore complexes Mr = 125,000,000 Da ~ 50 different proteins (nucleoporins) 17 Figure 12.54A. Molecular Biology of the Cell The Nuclear Pore Complex (NPC) Nuclear pore complexes perforate the nuclear envelope Figure 12.55. Molecular Biology of the Cell Freely permeable to small water soluble molecules (< 5000 Da) Molecules > 60,000 Da cannot pass Proteins with a nuclear localization signal (NLS) are recognised by nuclear import receptors RNA and new ribosomal subunits have nuclear export signals recognised by nuclear export receptors 18 Nuclear Localisation Signals (NLS) Nuclear localisation signals direct proteins to the nucleus Figure 12.56. Molecular Biology of the Cell 19 Nuclear Import Receptors Most nuclear localisation signals are specifically recognised by nuclear import receptors These are soluble cytosolic proteins that bind to both the NLS on the protein being transported and to NPC proteins Figure 12.54A. Molecular Biology of the Cell 20 Transport through the Nuclear Pore Complex Interaction between FG repeats on fibrils/filaments and binding sites on nuclear import receptors enables transport across the nuclear pore complex 21 Figure 12.59. Molecular Biology of the Cell Nuclear transport The Ran GTPase Imposes Directionality on Nuclear Import Through NPCs The critical difference between Ran-mediated nuclear import and nuclear export is the nature of cargo binding by the cargo receptor. In nuclear import, cargo binding is mutually exclusive of Ran-GTP; in nuclear export, cargo binding requires RanGTP. 22 Figure 12.61. Molecular Biology of the Cell Protein Road Map cytoplasm nucleus peroxisome mitochondria plastid endoplasmic reticulum lysosome golgi secretory vesicles endosome cell surface 23 Mitochondria Membrane bound organelles convert energy to forms that drive the cell (ATP) Matrix - hundreds of enzymes (TCA cycle) Inner membrane - ATP synthase etc. Outer membrane - porin Intermembrane space - several kinases Figure 12.47A. Molecular Biology of the Cell 24 Importing proteins into mitochondria and chloroplasts Translocation Into Mitochondria Depends on Signal Sequences and Protein Translocators Mitochondrial proteins are imported post-translationally as unfolded polypeptide chains Protein import is powered by ATP hydrolysis, a membrane potential, and redox potential Transport into the inner mitochondrial membrane occurs via several routes 25 Importing proteins into mitochondria Multi-subunit protein complexes that function as protein translocators mediate movement across mitochondrial membranes Figure 12.48A. Molecular Biology of the Cell Importing proteins into mitochondria TOM = Translocator, Outer Membrane TIM = Translocator, Inner Membrane ATP hydrolysis drives dissociation of protein from cytosolic Hsp70 and matrix mtHsp70 27 Figure 12.49. Molecular Biology of the Cell Importing proteins into the inner mitochondrial membrane Combination of translocator protein complexes, signal sequences and sometimes chaperones mediates movement into the mitochondrial membrane 28 Figure 12.51. Molecular Biology of the Cell 29 Chloroplasts Specialized plastid (photosynthesis) 6 compartments inner and outer envelope membrane intervening membrane space stroma thylakoid membrane lumen Figure 12.47B. Molecular Biology of the Cell 29 Importing proteins into chloroplasts Translocation into chloroplasts similar to mitochondrial transport → Occurs post-translationally → Uses separate translocator complexes in each membrane (TOC and TIC) → requires energy → Uses N-terminal signal sequences that are ckeaved after use TOC = Translocator, Outer Membrane of Chloroplasts TIC = Translocator, Inner Membrane of Chloroplasts 30 Chloroplast protein import mechanisms are similar to those of mitochondria Two signal sequences required for transport to the thylakoid lumen 31 Figure 12.53A. Molecular Biology of the Cell Chloroplast protein import mechanisms are similar to those of mitochondria There are several pathways that transport proteins from the stroma to the thylakoid space Hydrolysis of ATP and GTP drives import 32 Figure 12.53B. Molecular Biology of the Cell Protein Road Map cytoplasm nucleus peroxisome mitochondria plastid endoplasmic reticulum lysosome golgi secretory vesicles endosome cell surface 33 34 Peroxisomes electron micrograph of three peroxisomes in a rat liver cell Single membrane organelle Oxidizes organic molecules Use O2 to remove H2 Produces and degrades H2O2 Breakdown of fatty acids ¢ (Acetyl coA -> TCA cycle) Ancient metabolic organelle? Figure 12.43. Molecular Biology of the Cell 34 Importing proteins into peroxisomes Surrounded by only a single membrane Acquire most proteins by selective import from the cytosol but some enter the via the ER Short signal sequences direct the import of proteins into peroxisomes Soluble Peroxisomal Targeting Signal (PTS) receptors and docking receptors on the cytosolic surface help Driven by ATP hydrolysis 35 Import into Peroxisomes Ma, Changle & Agrawal, Gaurav & Subramani, Suresh. (2011). Peroxisome assembly: Matrix and membrane protein biogenesis. The Journal of cell biology. 193. 7-16. Mechanisms not well understood >23 proteins involved in the import process - peroxins A complex of at least 6 different proteins forms a translocator Imported in native confirmation aided by at least one soluble import receptor, peroxin 5, Pex5 Pex5 carries its cargo all the way into the peroxisome before being recycled 36 Summary Although most proteins are synthesised in the cytoplasm, they are directed to their final compartmental location by specific sorting signals Proteins synthesized in the cytosol may traffic to either the nucleus, mitochondria, chloroplast or peroxisome Nucleus: bidirectional transport through nuclear pore complex (NLS + importins/exportins, Ran-GTP + pore complex) Mitochondria: signal sequence + chaperones + TOM/TIM complex + ATP Chloroplast: similar to mitochondria (signal sequences, chaperones, TOC/TIC, ATP/GTP) Peroxisomes: PTS/mPTS + PTS receptors 37 Example Exam Questions Describe the experiments that could be carried out to confirm that a sorting signal is directing a protein to the nucleus (3 marks) Describe, with the aid of a diagram, how cytosolic proteins are trafficked to the mitochondrial matrix (10 marks) Identify and briefly describe the two ways sorting signals can be built into a protein (3 marks) Describe, with the aid of a diagram, how cytosolic proteins are trafficked to the nucleus (10 marks) 38 BIOL340 Molecular Cell Biology Week 4 Macromolecular Traffic Dr Jody Gorman [email protected] Becker’s World of the Cell. 9th Edition. Chpts 12, 16 and 19 Molecular Biology of the Cell, 7th ed. Various chapters` 1 Overview The Endoplasmic Reticulum – SER versus RER Polysomes and protein translation Co-translational import of proteins Vesicular transport of proteins in cells Summary 2 Protein Road Map cytoplasm nucleus 1 Proteins synthesised on ribosomes attached to ER (RER) peroxisome mitochondria plastid endoplasmic reticulum Transmembrane transport lysosome Vesicular transport endosome golgi secretory vesicles cell surface 3 The Endoplasmic Reticulum An extensive network of membrane surrounded sacs and tubules rough Central role is lipid and protein ER biosynthesis Internal space (lumen) separate from the cytosol 2 types: ¢smooth ER ¢rough ER Fig. 12.15A. Molecular Biology of the Cell. smooth ER VERY DYNAMIC STRUCTURE Fig. 12.15B. Molecular Biology of the Cell. 4 Review - SER versus RER RER bound ribosomes on cytosolic face synthesis of proteins destined for endomembrane system or export addition of simple oligosaccharides to proteins SER no bound ribosomes lipid metabolism synthesis of cholesterol and steroid hormones detoxification of foreign substances 5 The Endoplasmic Reticulum and Lipid Production ER membrane is the site of production of most lipid classes membrane lipids for new cell PMs establishes polarity of membranes (later) https://www.youtube.com/watch?v=UcQE_YOrTjA Fig 12.14. Molecular Biology of the Cell. 6 The Endoplasmic Reticulum and Protein Production: Polysomes Polysomes or polyribosomes occur when multiple ribosomes (80 – 100 nucleotides apart) are translating protein simultaneously on a single mRNA molecule Fig. 6.77. Molecular Biology of the Cell. 7 Found free in the cytosol OR attached to the ER Fig. 12.21. Molecular Biology of the Cell. 8 Protein Road Map Two distinct import mechanisms: ¢Posttranslational import ¢Cotranslational import Three distinct routes of protein importation into organelles 9 Protein Transport Importation can occur →co-translationally OR →post-translationally 10 Figure 12.24. Molecular Biology of the Cell Transport of Soluble Proteins in the ER Fig. 12.25B. Molecular Biology of the Cell. Hsp70 chaperones bound to the polypeptide detach as its passed through the membrane Requires Sec62 and Sec63 complex attached to the Sec61 translocator and positions Bip molecules to bind the polypeptide in the ER lumen Bip chaperones assist pulling the polypeptide into the ER lumen using a ratchet like mechanism Requires energy 11 Co-translational import of proteins in the ER The ribosome is brought to the membrane by the SRP and SRP receptor and then engages with the Sec61 translocator. The growing polypeptide chain is threaded across the membrane as it is made. No additional energy is needed, as the only path available to the growing chain is to cross the membrane. Fig. 12.25A. Molecular Biology of the Cell. 12 Import of proteins into the ER occurs co-translationally One end of protein is translocated into the ER lumen as the rest of the polypeptide is being synthesized The proteins are never released into the cytosol There is no danger of the protein folding before reaching the membrane-associated translocator No need for cytosolic chaperones to keep the protein unfolded A different mechanism to the post-translational import of proteins into mitochondria/nuclei/peroxisomes Involves a signal recognition particle 13 A signal-recognition particle (SRP) directs ER signal peptides to a specific receptor in the RER membrane As a signal sequence emerges from the ribosome and binds to the SRP, a conformational change in the SRP exposes a binding site for the SRP receptor Fig. 12.19. Molecular Biology of the Cell. 14 Co-translational import of proteins into the ER The SRP receptor in the ER membrane, binds the SRP–ribosome complex and directs it to the translocator Fig. 12.20. Molecular Biology of the Cell. The SRP (in complex with SRP receptor) then moves away from its binding site on the ribosome, which is then occupied by the translocator in the ER membrane. SRP then releases the signal sequence, which inserts into the translocator to initiate polypeptide chain transfer across the lipid bilayer. The SRP and SRP receptor dissociate from each other and are recycled for the next round of protein targeting. 15 Transport of Single-pass Transmembrane Proteins in the ER An N-terminal signal sequence initiates translocation, same as for soluble protein, but an additional hydrophobic segment acts as a stop-transfer signal to anchor the protein in the membrane Fig. 12.27. Molecular Biology of the Cell. https://www.youtube.com/watch?v=gq77U09HZrI 16 Single-pass Transmembrane Proteins Internal start-transfer sequences can bind the translocator in either of two orientations (C-term or N-term on luminal side of ER) Fig. 12.26. Molecular Biology of the Cell. Transmembrane proteins contain hydrophobic segments that are recognized like signal sequences and remain in the lipid bilayer as a membrane-spanning a-helix Transport of multipass integral membrane proteins in the ER Combinations of start- and stop- transfer signals determine the topology of multipass integral membrane proteins Each successive transmembrane segment is similarly inserted into the membrane via the lateral gate in an orientation opposite to that of the transmembrane segment that immediately preceded it. Fig. 12.28. Molecular Biology of the Cell. 18 Folding and assembly of translocated proteins is assisted by ER resident proteins ER retention signal (-Lys-Asp-Glu-Leu-COO-) at C-terminus is responsible for retaining the protein in the ER ER resident chaperones assist proper protein folding e.g. BiP →pulls proteins translationally into the ER →recognizes misfolded proteins as well as protein sub-units that have not assembled into their oligomeric form Protein disulfide isomerase (PDI) catalyses the oxidation of free sulfhydryl (SH) groups on cysteines to form disulphide bonds (S-S) --SH + SH---S S-All cysteines in protein domains exposed to (normally oxidising) extracellular spaces are disulphide linked (-S-S-); this is not the case in the normally reducing cytosol (-SH) 19 Glycosylation (addition of sugars) to proteins in RER Most proteins synthesised in the rough ER become glycoproteins including those destined for the golgi, lysosomes, plasma membrane or extracellular space Largely achieved through addition of a common N-linked oligosaccharides Simple oligosaccharides added by large family of glycosyltransferase Key functions of carbohydrate groups on glycoproteins: ¢Can be important in binding interactions with other macromolecules ¢Aid correct folding ¢Can be essential for secretion 20 Protein glycosylation in the rough ER A single N-acetylglucosamine group is added to a serine and threonine residue of the protein – this is a simple sugar characteristic of cytosolic proteins Catalyzed by membrane bound oligosaccharyl transferase enzyme associated with translocon Figure 12.32B. Molecular Biology of the Cell 21 Protein Road Map cytoplasm nucleus peroxisome mitochondria plastid endoplasmic reticulum lysosome golgi secretory vesicles endosome cell surface 22 Membrane asymmetry is initially established in the ER Most membrane lipid bilayers are assembled in the ER Transmembrane proteins inserted in predictable orientation determined by aa sequence ER is also site of most membrane lipid biosynthesis: ¢Lipids inserted into cytosolic face of bilayer are bidirectionally moved to reach asymmetry by scramblases ¢Flipases moves specific lipids into other half of bilayer to produce an asymmetric distribution Membranes grow as newly synthesised proteins and lipids are inserted into the existing ER membrane 23 Maintenance of membrane asymmetry: vesicular transport The golgi and other cell compartments receive their lipids from ER vesicles They have asymmetric phospholipid composition maintained by flipases Phospholipid synthesis occurs at the cytosolic half of the ER membrane but equilibrate across the bilayer in minutes by scramblase Non specific Bidirectional energy independent Specific Unidirectional Requires ATP Figure 12.39. Molecular Biology of the Cell 24 Vesicular Transport Transport vesicles bud off from one compartment and fuse with another They carry material as cargo from the lumen and membrane of the donor compartment to the lumen and membrane of the target compartment Figure 13.2. Molecular Biology of the Cell 25 Trafficking through the Endomembrane System Most proteins are transported in vesicles from the ER to the Golgi Here they are sorted and transported to their destination in vesicles e.g. Proteins destined for lysosomes recognised by their phosphorylated Nlinked carbohydrates (receptor) Figure 13.3B. Molecular Biology of the Cell 26 Transport of proteins from the ER to the Golgi Apparatus Figure 13.23. Molecular Biology of the Cell Proteins Leave the ER in COPIIcoated Transport Vesicles Transmembrane proteins (approx. 200 per vesicle) are packaged into budding transport vesicles through interactions of exit signals on their cytosolic tails with adaptor proteins of the inner COPII coat. Some of these transmembrane proteins function as cargo receptors, binding soluble proteins in the ER lumen and helping to package them into vesicles. Chaperones ensure proteins are properly folded before they leave the ER 27 Trafficking through the Endomembrane System Step 1. Ribosomes of the RER synthesise proteins; proteins enter ER lumen; initial glycosylation Step 2. Transition vesicles carry proteins and lipids to cis-Golgi network (CGN). Step 3. Lipids and proteins move through the cisternae of the Golgi. Step 4. At the trans-Golgi network (i) some vesicles form secretory vesicles that release contents by exocytosis, (ii) others form endosomes and then lysosomes. https://www.youtube.com/watch?v=rvfvRgk0MfA 28 Summary ER is the site of synthesis of proteins and lipids N-terminal ER signal sequence on a polypeptide means it will be bound for the RER (co-translational translocation) - These proteins will be either retained in the ER lumen, secreted, or become integral membrane proteins of the endomembrane system Most polypeptides are modified in the ER lumen Further processing occurs as they move along the vesicle transport pathway through the Golgi After the Golgi, proteins are packaged into secretory vesicles or lysosomes 29 Example Exam Questions Describe the specific steps required for cotranslational import of a polypeptide into the endoplasmic reticulum lumen (10 marks) Briefly explain the two signals that are involved in the formation of a transmembrane protein (3 marks) Name 3 of the final destinations for proteins in the vesicular transport system (3 marks) Explain how proteins in the ER lumen can be secreted from the cell or travel to lysosomes, providing details on both pathways (10 marks) 30 BIOL340 Molecular Cell Biology Week 7 The Cytoskeleton Dr Jody Gorman [email protected] Molecular Biology of the Cell, 7th ed. Chapter 16 Becker’s World of the Cell. 9th Edition. Chapter 13 1 The Cell https://www.yourgenome.org/facts/what-is-a-cell/ Fig. 1.21. Molecular Biology of the Cell. Have you ever thought about… How nerve cells maintain their shape and get materials made up in the body to the other end? How DNA chromatids are separated during cell division? How epithelial cells lining the respiratory tract sweep mucus carrying debris up and out of our lungs? The Cytoskeleton The interior of a cell is highly structured The cytoskeleton is a network of interconnected filaments and tubules extending through the cytosol It provides strength and shape It plays roles in cell movement and division It drives and guides intracellular organelle traffic It is dynamic and changeable A remarkable and dynamic system of filaments giving shape, structure and strength to cells as well as driving and guiding intracellular organelle traffic Overview Cytoskeleton components and function overview Microtubules Composition, Organisation, Assembly and Function Dynamic Instability Microfilaments Composition, Organisation, Assembly and Function Treadmilling Intermediate filaments (brief) MT and MF binding proteins – they don’t work alone! Using drugs/poisons to study the cytoskeleton Disease examples Types of Cytoskeletal Elements 3 basic types of cytoskeletal elements: - 25 nm - 7 nm - 8-12 nm An Interconnected Network These cytoskeletal elements interact with hundreds of accessory proteins that regulate and connect the filaments to each other and other cytoskeletal components Dynamic and Adaptable A fibroblast growing and dividing is an example of the rapid reorganisation capable of the cytoskeleton Fig. 16.2. Molecular Biology of the Cell. Stable Structures and Cellular Polarity The dynamic elements of the cytoskeleton produce stable large scale structures for cellular organisation The cytoskeleton is also responsible for large-scale cellular polarity, enabling cells to tell the difference between top and bottom or front and back Fig. 16.4. Molecular Biology of the Cell. Microtubules (MTs) MTs are the largest structural elements of the cytoskeleton They are involved in a variety of functions in the cell They are rigid Typically have one end attached to a microtubuleorganising centre (MTOC) Two main types – Cytoplasmic microtubules – Axonemal microtubules Microtubules - YouTube Panel 16.1. Molecular Biology of the Cell. Cytoplasmic MTs Cytoplasmic MTs pervade the cytosol and are responsible for a variety of functions – Maintaining or altering cell shape – Placement and movement of vesicles – Formation of mitotic and meiotic spindles – Maintaining axons Fig. 16.44. Molecular Biology of the Cell. Fig. 16.1B. Molecular Biology of the Cell. 4 Axonemal MTs Include the organised and stable MTs found in structures such as: – Cilia and flagella – Basal bodies to which cilia/flagella attach Biology, 2nd ed, New York: McGraw-Hill, p 77 The axoneme, the central shaft of a cilium or flagellum, is a highly ordered bundle of MTs Microtubule Composition The basic subunit of a MT protofilament is a heterodimer of tubulin: one α-tubulin and one β-tubulin bound non-covalently to each other All the dimers are oriented the same way Because of dimer orientation, protofilaments have an inherent polarity Fig. 16.37. Molecular Biology of the Cell. Microtubule Composition MTs are a hollow cylindrical structures built from 13 parallel protofilaments of tubulin protein Biggest and most rigid of the microfilaments Can form singlets, doublets and triplets Fig. 16.37. Molecular Biology of the Cell. Microtubule Assembly - Nucleation Will self-assemble in-vitro but nucleation is the rate limiting step Nucleation creates a ring structured MT ‘seed’ for growth (SLOW) – 13 dimers More commonly seeded by gamma-tubulin ring in Microtubule Organising Centre (MTOC) to speed things up Fig. 16.41. Molecular Biology of the Cell. Microtubule Organisation MTs originate from microtubule-organising centres (MTOCs) within the cell 1. Centrosomes near the nucleus 2. Basal bodies near the cell membrane in cilia and flagella In animals, the centrosome is associated with two centrioles surrounding by pericentriolar material Fig. 16.42. Molecular Biology of the Cell. Microtubule Organisation MTOCs nucleate (form MT seed) and anchor MTs MTs grow outward from the MTOC with a fixed polarity – the minus ends are anchored in the MTOC Because of this, dynamic growth and shrinkage of MTs occurs at the plus ends, near the cell periphery No MTOC No MTOC Microtubule Assembly MTs form by the addition of tubulin dimers at their ends MTs form by the reversible polymerisation of dimers in the presence of GTP and Mg2+ Dynamic Instability Constant polymerisation and depolymerisation cycle (dynamic instability) is a unique property of microtubules Fig. 16.39. Molecular Biology of the Cell. MT Binding Proteins Modulate Filament Dynamics and Organization Fig. 16.46. Molecular Biology of the Cell. Some mediate the interaction of MT’s with other cell components Panel 16.4. Molecular Biology of the Cell. Microtubule Targeting Agents A diverse group of chemical compounds that bind to microtubules and affect their properties and function Interfere with tubulin assembly/disassembly and therefore altering the equilibrium between subunits Disruption of microtubules induces various cellular responses often leading to cell cycle arrest or cell death Microtubule Dysfunction & Disease MTs allow for nutrient transport in neurons Tau helps support these MTs Hyperphosphorylated Tau forms aggregates, dissociating from MTs Lack of nutrients starves neurons Associated with Alzheimer’s disease Microtubule Summary Structure – α- and β-tubulin heterodimers form protofilament that coils into a tube with polarity – Often organised at MTOCs Function – Maintaining axons, vesicle transport, mitotic spindle formation Dynamic instability – Allows controlled growth/decay of MTs Can be targeted with drugs/poisons – Taxol, vinblastine, nocodazole, colchicine Microfilaments (MFs) Smallest of the cytoskeletal filaments – 7nm Helical polymers of actin Flexible structures Dispersed throughout the cell but most highly concentrated in the cortex beneath the plasma membrane Organise into a variety of linear bundles, 2D networks and 3D gels Panel 16.1Fig. 16.37. Molecular Biology of the Cell. Fig. 16.18A. Molecular Biology of the Cell. Microfilaments (MFs) Best known for their role in muscle contraction Develop and maintain cell shape Involved in cell migration and cytoplasmic streaming Structural core of microvilli Fig. 16.19. Molecular Biology of the Cell. Microfilament Composition Each actin subunit is a 375-amino-acid polypeptide carrying a tightly associated molecule of ATP or ADP Actin subunits assemble head to tail to form a protofilament Two protofilaments form a helix held together by lateral contacts Like tubulin the filaments have polarity and the ends differ chemically and structurally Fig. 16.8. Molecular Biology of the Cell. Microfilament Assembly G(lobular)-actin monomers reversibly polymerize into actin microfilaments (“F-actin”) G-actin is added rapidly at the plus end and slower/lost at the minus end G-actin monomers nucleate slowly but then can assemble onto MF more rapidly Ø bound ATP is then slowly hydrolysed to ADP Growing MF ends have ATP-actin, whereas most of the MF is composed of ADP-actin Does this look familiar? Treadmilling Subunits cycle rapidly between the free and filamentous states Ability to maintain a constant length despite a net flux of subunits through the polymer Occurs at a subunit concentration where filament growth balances shrinkage Requires energy in the form of ATP hydrolysis Panel 16.2. Molecular Biology of the Cell. Fig. 16.11B. Molecular Biology of the Cell. Actin Binding Proteins Influence filament dynamics and organization Fig. 16.14. Molecular Biology of the Cell. Panel 3. Molecular Biology of the Cell. Profilin stimulates actin filament elongation Drugs can be used to perturb microfilaments Like for MT’s, the role of MF’s can be determined through chemical inhibition All of these compounds cause dramatic changes in the actin cytoskeleton and are toxic to cells, indicating that the function of actin filaments depends on a dynamic equilibrium between filaments and actin monomers Microfilament Dysfunction & Disease Mutations in the skeletal muscle α-actin gene (ACTA1) may affect its ATP binding ability and cause a range of congenital myopathies People with actin-accumulation myopathy have severe muscle weakness (myopathy) and poor muscle tone (hypotonia) throughout the body Floppy baby syndrome can be detected shortly after birth Symptoms include feeding and swallowing difficulties, a weak cry, and difficulty with controlling head movements Microfilament (MF) Summary Structure – G-actin monomers polymerised into microfilament – Assembled like MTs, have polarity and dynamic instability Function – Locomotion (via contraction) and cell shape Highly regulated by actin-binding proteins Intermediate Filaments (IFs) Most stable component of the cytoskeleton – less dynamic Likely support the entire cytoskeleton and confer strength on tissues IF’s are rope-like fibres with a 10 mm diameter Made from a large diverse family of intermediate filament proteins Panel 16.1. Molecular Biology of the Cell. Intermediate Filament Assembly Basic structural unit consists of two IF polypeptides intertwined into a coiled-coil The two dimers are aligned in parallel The parallel dimers align laterally to form a tetrameric protofilament Protofilaments overlap to build up a filamentous structure ~8 protofilaments thick Fig. 16.62. Molecular Biology of the Cell. Intermediate Filaments Unlike actin and tubulin which is highly conserved IF’s are much more diverse with distinct cell types and specific function Encoded by 70 different human genes! Keratins and Mechanical Stability A single epithelial cell can produce multiple types of keratins which co-polymerise into a single network Held together by disulfide bonds Form tough coverings for animals, as in the outer layer of skin and in hair, nails, claws, and scales Impart mechanical strength by anchoring the intermediate filaments at sites of cell–cell and cell matrix contact – desmosomes and hemidesmosomes Keratin Mutation and Disease Mutations in keratin genes cause several human diseases Eg, epidermolysis bullosa simplex Occurs when defective keratins are expressed in the basal cell layer of the epidermis Results in the skin blistering to even mild mechanical stress Fig. 16.64. Molecular Biology of the Cell. Intermediate Filament Summary Most stable component of cytoskeleton Diverse with distinct cell type and specific function Structure: – tetrameric protofilaments joined together Functions: – structural support, scaffolding, give cell shape and strength Summary Structural and motility structural Example Exam Questions Compare and contrast the 3 cytoskeletal elements within cells including their assembly, structure and function (10 marks) Which cytoskeletal components experience dynamic instability? (1 mark) Identify the two subsets of microtubules and briefly describe their function (3 marks) What regulates microfilament organisation? (1 mark) BIOL340 Molecular Cell Biology Week 7 Cell Motility Dr Jody Gorman [email protected] Molecular Biology of the Cell, 7th ed. Chapter 16 Becker’s World of the Cell. 9th Edition. Chapter 13 1 Overview Cell motility as an important function of the cytoskeleton Motor proteins – Kinesins and dyneins (MTs) – Myosins (MFs) Cell motility examples – Moving cargo – Cell protrusions and locomotion Motility Systems Motility occurs at the tissue, cellular, and subcellular levels Cells move; for example neutrophils ‘crawl’ to sites of infection and engulf foreign invaders Intracellular components move; for example microtubules of the mitotic spindle play a role in the separation of chromosomes during cell division To generate movement, MTs and MFs provide a scaffold for motor proteins that produce motion at the molecular level Phagocytosis of MRSA by a human neutrophil - YouTube Two Motility Systems 1. Microtubule-based motility – – – – transport of organelles and vesicles Formation of mitotic and meiotic spindles fast axonal transport in neurons sliding of MTs in cilia and flagella 2. Microfilament-based motility – – – muscle contraction cell shape changes and migration cytoplasmic streaming Motility Systems Are driven by motor proteins – They couple ATP hydrolysis to changes in shape and attachment of the motor protein i.e. they convert chemical energy into mechanical work – They undergo cycles of ATP hydrolysis, ADP release, and acquisition of new ATP – They have common structural features – They can move along a cytoskeletal filament for significant distances – They move unidirectionally along their cytoskeletal track in a stepwise manner – dynamic instability regulates this signalling Motor Protein Classes and Functional Domains Three categories of molecular motors: Kinesin and dynein move along MT tracks Myosins move along MF tracks Functional domains include: Motor to power motion Lever transduces conformational change Binding domain to attach cargo Microtubule-based Motility Kinesins & Dyneins MTs provide a rigid set of tracks for transport of a variety of organelles and vesicles (cargo) Traffic toward the negative ends of MTs is considered ‘inbound’; toward the positive end is ‘outbound’ – Remember: minus end generally seeded at MTOC https://step1.medbullets.com/biochemistry/102076/microtubules Motor Proteins - Axonal Transport Proteins produced in the cell body are transported to the nerve ending in a process called fast axonal transport This involves packaging of these proteins into vesicles for transport Organelles can also be observed moving along filaments through axoplasm (cytoplasm of axons) at rates of about 2 µm/sec Two proteins are responsible for fast axonal transport – Kinesin I Involved in ATP-dependent transport toward the positive end (outbound) Called anterograde axonal transport – Cytoplasmic dynein Moves particles in the opposite direction so to the negative end (inbound) Called retrograde axonal transport Motor Proteins - Axonal Transport http://humanphysiology.academy Kinesins Move toward plus end of MTs Consist of two dimerised heavy chains and two light chains The heavy chains contain globular domains that attach to MTs, a coiled-coil stalk, a leverlike neck that connects the two, and a tail Fig. 16.52. Molecular Biology of the Cell. Kinesin Mediated Transport Movement looks like walking, the head domains take turns as the front foot ADP bound head binds to tubulin and ADP is displaced for ATP which results in the rear head flipping forward affects the confirmation flipping the linker forward and the lagging head with it to next tubulin to which it binds and then the cycle is repeated It can move long distances before detaching from it by releasing bound ADP and acquiring a new ATP, so that the cycle repeats Fig. 16.52. Molecular Biology of the Cell. Kinesins and Intracellular Transport Dyneins Family of minus end–directed microtubule motors structurally unrelated to the kinesins Largest of the motor proteins Divided into two categories 1. Cytoplasmic dynein – associated with a protein complex called dynactin, which helps link dynein to cargo 2. Axonemal dyneins – specialized for the rapid and efficient microtubule sliding movements that drive the beating of cilia and flagella Fig. 16.56. Molecular Biology of the Cell. Dynein-Mediated Transport Although structurally unrelated to myosins and kinesins, dyneins follow the general rule of coupling ATP hydrolysis and conformational change to microtubule binding and unbinding resulting in a power-stroke forward Fig. 16.55. Molecular Biology of the Cell. Dynein Mediated Transport Dynein Mediated Transport Can also “Jog” in place causing the whole microtubule to roll along beneath them – often occurs in conjunction with depolymerisation of the MT How chromatids are separated and dividing cells are separated during mitosis Dynein complex bound to membrane but motor can move along MT’s so can pull it in a certain direction MT Motors & the Endomembrane System Membrane extensions of the ER can be moved along MTs The vesicles to and from the Golgi complex are carried by MT motors on MTs Movement based on polarity and MT’s Kinesin – – Golgi èER – Golgi èCell membrane (exocytosis) Dynein – – ER è Golgi – Cell membrane è Golgi (endocytosis) Summary – MT Associated Motor Proteins Function largely to transport cargo around the cell Utilise ATP for mechanical energy Unidirectional MT proteins – Kinesin Plus end-directed MT motor – Cytoplasmic dyneins Minus end-directed MT motor Microfilament-based Movement Myosins ATP-dependent motors, the large superfamily called myosins, interact with and exert force on actin microfilaments All have at least one heavy chain, with a globular head group attached to a tail of varying length Myosin II was the first motor protein to be identified and is the best understood Myosin Contraction Cycle Myosin uses a cycle of structural changes to ‘walk’ along an actin filament Attached: myosin locked tightly to actin in a rigor confirmation Released: binding ATP causes confirmational change and reduced affinity for actin Cocked: the change also causes the lever arm to swing and ATP is hydrolysed Re-binding: the myosin head binds weakly to a new site 5 nm along the actin filament Power stroke: Pi is released and myosin becomes tightly bound Force generating: myosin again locked tightly to actin in a rigor confirmation at the new position Fig. 16.24. Molecular Biology of the Cell. Myosin vs Kinesin Both are efficient motor proteins that exert similar forces Both have globular domains that walk along a protein filament, and both use ATP hydrolysis to change their shape However Kinesins operate alone or in small numbers to transport vesicles over large distances Myosin II molecules move short distances but operate in large arrays, in some cases billions of motors working together to mediate muscle contraction Myosin II and Muscle Contraction The basic function of myosin II is to pull arrays of actin filaments together, resulting in rapid and efficient contraction of muscle cells Skeletal muscle fibres are huge single cells produced from fusion of many cells, the cytoplasm of which is primarily filled with myofibrils Myofibrils are repeating units of contractile units called sarcomeres A SKELETAL MUSCLE CELL CONTRACTION Fig. 16.26 and 16.27. Molecular Biology of the Cell. Non-muscle Cell Motility Non-muscle cells lack cilia/flagella They can instead ‘crawl’ using lamellipodia and/or filopodia Crawling involves: Protrusion produced by polymerisation of the branched actin network at the leading edge Attachment to substratum proteins via focal adhesions Tension to detach the trailing edge which is then drawn forward by contraction of the cell body Microfilament-based Motility Summary Coordinated by myosin – Myosin II similar to kinesin, but present in large numbers to facilitate muscle contraction Operates in a contraction cycle, producing a ‘power-stroke’ Non-muscle cells move via polymerisation of actin at leading edge, attachment to substratum, actin-myosin contraction, and retraction of rear by depolymerisation Summary – Cell Motility MTs and MFs can interact with motor proteins Cell motility and intracellular movements are driven by motor proteins – Couple ATP hydrolysis to movements along MTs (kinesins and dyeinins) or MFs (myosins) – ATP hydrolysis is coupled to conformational changes in the motor allowing it to move along a cytoskeletal element Cell locomotion involves cycles of protrusion, attachment, translocation, and detachment Sci-Show – Motor proteins: Tiny pirates in your cells https://www.youtube.com/watch?v=SgR4ojtPw5Q Sample Exam Questions Describe how cargo is transported between intracellular cell compartments (7 marks) What are the three types of motor proteins? (1 1/2 marks) What are the two cell motility systems? (1 mark) Describe the steps involved in non-muscle cell locomotion (5 marks) BIOL340/981 Cell and Molecular Biology Cancer Week 8: Lecture 1 Dr Debbie Watson [email protected] Building 32.309 Consultation: Email for appointment School of Chemistry and Molecular Bioscience 1 Overview Lecture 1: Cancer Definition of cancer and nomenclature Causes of cancer Cancer initiation and progression Phenotypic (cellular) features of benign and malignant cancers Chapter 20 Cancer Molecular Biology of the Cell Textbook Lecture 2: Cancer Regulators of cancer progression Oncogenes Tumour suppressor genes Epigenetic changes in gene expression Multistep model of cancer progression Gene signatures Cancer therapeutics 2 Learning Objectives To understand the hallmarks of cancer To distinguish between benign and malignant cancer To describe different types of cancers To understand factors that can initiate cancer To understand cancer progression and metastases 3 What is cancer? A group of diseases ( > 277 types) characterised by a loss of normal cell growth control Normal cells Proliferate when required Undergo apoptosis when Damaged irreversibly After a finite number of cell divisions Cancer cells Do not respond to control mechanisms 4 Common hallmarks of cancer growth Altered homeostasis that results in cells growing and dividing at a faster rate than they die Bypass of normal limits to cell proliferation Evasion of cell-death signals Altered cellular metabolism Manipulation of the tissue environment to support cell survival and to evade a deleterious immune response Escape of cells from their home tissues and proliferation in foreign sites (metastasis) Ch 20 pg 1171 Molecular Biology of the Cell 5 Cancer cells have uncontrolled proliferation and metastasis Cancer cells share two fundamental properties oAbnormal cell growth and division (uncontrolled proliferation) oDefects in the normal restraints that keep cells from spreading and colonising other parts of the body (metastasis) In normal cells these processes are tightly controlled by genes (time and place) In cancer cells these genes are mutated or expressed inappropriately Cancer cells display altered control of growth and homeostasis Figure 20-14 Molecular Biology of the Cell 7 Cancer cells have uncontrolled proliferation and metastasis Source of Image https://www.coursehero.com/sg/cell-biology/mechanisms-of-cancer/ 8 The Hallmarks of Cancer 9 The Metabolic Pathways of Cancer 10 Benign tumour (neoplasm) Abnormally growing cells which remain as a single mass Non-cancerous Restricted within capsule of connective tissue Malignant tumour Tumour cells with the ability to invade through surrounding normal tissues (invasion) and spread to anatomically distant sites (metastasis) True cancer, responsible for > 90% of cancer deaths 11 Benign vs malignant cancers Normal glands Capsule of connective tissue Diffuse invasive cells No evidence of barriers Benign Malignant Adenoma (glandular Adenocarcinoma neoplasm) See similar Figure 20-3 Molecular Biology of the Cell 12 Cancer growth affects tissue function Normal skin Squamous cell carcinoma Connective tissue Connective tissue 13 Nomenclature Dependent upon site of origin Carcinoma Epithelial cell Adenocarcinoma (from a glandular neoplasm) Leukaemia, lymphoma, myeloma Hematopoietic cells Sarcoma Connective tissue Gliomas, retinoblastomas Central nervous system (CNS) 14 Example of a Quiz Question Describe the phenotypic differences between normal and tumour cells. 15 Cancer initiation and progression Cancer is a multistep process characterised by a progression of permanent alterations in cells 16 Initiation of cancer What factors influence initiation (and progression) of cancer? 1. 2. 3. 4. Environmental factors Infectious agents Family history (germ-line mutations) Age mutagens 17 1. Environmental factors Chemicals (carcinogens) Agents that cause simple local changes in nucleotide sequence E.g. smoking tobacco (lung cancer) Ionising radiation Chromosome breaks and translocations E.g. UV radiation (skin cancer) 18 2. Infectious agents Viruses DNA and RNA tumour viruses introduce foreign genetic material E.g. human papilloma virus (cervical cancer) Bacteria Produce toxins that disrupt cell signalling Induce chronic inflammation E.g. H. pylori (gastric cancer) 19 3. Inherited mutations Germ-line mutations Present in egg or sperm Cause cancer family syndrome Mutations in normal genes related to cell cycle controls Increased risk of specific types of cancer 20 Examples of Inherited mutations oColon cancer – tumour suppressor gene (APC) oRetinoblastoma – deletion (13q) oBreast cancer – genes that produce proteins that repair damaged DNA (BRCA1 & BRCA2) (5-10%) oLynch Syndrome - genes that affect DNA mismatch repair (MLH1) 21 3. Inherited mutations Most cancers are NOT hereditary Majority of human cancers result from an accumulation of somatic mutations Over 80 yrs, 1015 body cells replicate Random errors occur over a lifetime 22 4. Age Largest risk factor 23 Example of a Quiz Question What factors can affect tumour initiation 24 Tumour progression Progression can take years Mutant cells are selectively favoured over their neighbouring, normal cells 25 Tumour progression Subsequent mutations lead to more aggressive phenotypes 26 Tumour progression With the correct phenotype, tumour cells become malignant They can invade and metastasise* *Dissemination of cancer cells to anatomically distant organ sites and subsequent adaptation to foreign tissue microenvironments http://media.pearsoncmg.com/bc/bc_becker_woc_7/vids/html/24_2_TumorGrowth.html 27 Invasion and Metastasis Local invasion Metastasis 28 Metastatic spread Different cancer types exhibit remarkable variability in their metastatic course Liver of a breast cancer patient Length of the latency period or time to spread (months to years) Different organs affected (most commonly the liver, lung, bone, and brain) 29 Metastatic spread in GBM is rare Medicinal Research Reviews 2016 DOI 10.1002/med.21408 30 Brain cancer kills more children than any other disease 31 Tumour phenotype Key phenotypic features are shared by benign and malignant tumour cells Nuclear alterations Altered morphology & disorganised growth patterns Immortal 32 Phenotype vs genotype 33 Nuclear alterations Differences in nuclear morphology Papanicolaou (Pap) test Normal Abnormal cells in tissue (dysplasia) Invasive carcinoma 34 Nuclear alterations Aneuploidy (abnormal chromosome number) due to defect in mitotic spindle checkpoint Karyotype of a breast cancer cell showing a highly abnormal chromosome complement. 35 Altered morphology & disorganised growth Cancer cell proliferation is anchorage-independent and insensitive to population density Normal cells do not grow well in culture without a solid surface to attach to Stop dividing when they become confluent Tumour cells look different (cytoskeletal rearrangement) and grow in disordered multilayered clumps in vitro Contact inhibition Loss of contact inhibition 36 Immortality o Limitless replicative potential Cell number Cancer cells are no longer committed to die Tumour cells Only those tumors containing cells that maintain their telomere length will be capable of unlimited growth o 1. Any cell appearing within tumor that expresses telomerase will have a tremendous growth advantage over cells that lack it o 2. Over time, telomerase-containing cells will flourish, while those lacking it will die off; eventually, all of the cells in the tumor will contain telomerase Normal cells 1 2 3 4 Days in culture 37 Lecture 1 Cancer Summary I Benign tumour Abnormal cell growth, non-cancerous, confined to capsule Malignant tumour Can invade or metastasise, leading to cancer Tumours initiated through various factors Tumour progression caused by successive mutations, leading to an aggressive phenotype Benign and malignant tumours share some phenotypic features, but 38 Malignant tumours Malignant tumour cells can invade and metastasise This requires additional cellular alterations, or ‘progression factors’ Progression factors include Invasive capacity Enhanced expression of tissue degrading proteases Epithelial-Mesenchymal Transition (EMT) Together, enhance motility Angiogenesis Formation of new blood vessels 39 Invasive capacity: Enhanced expression of proteases 1. Cleaves cell surface proteins 2. Allows ECM breakdown 3. Activates growth factors or cytokines 1. 2. 3. 40 Invasive capacity: Epithelial-Mesenchymal Transition (EMT) 41 Malignant tumours are motile Increased cell motility is a fundamental characteristic of cancer cells Required in order for cells to invade through the basement membrane Represents an initial step in the metastatic cascade Necessary for cells to move from their primary organ of origin to distant metastatic sites MCF-7 (Green) non-invasive HT-1080 (Red) invasive 42 Angiogenesis Angiogenesis = formation of new blood vessels Feeds the tumour and provides a mode of transport 43 Angiogenesis Will NOT grow > 1-2 mm without blood supply 44 The metastatic cascade NB: angiogenesis not shown Arrest at new site Survival of CTCs in the circulation CTCs = Circulating Tumour Cells 45 The metastatic cascade 1. Dysplasia 2. Formation of primary tumour 3. EMT 4. Intravasation 5. Circulation 6. Arrest at new site 7. Extravasation 8. MET 9. Micrometastasis 10. Macrometastasis EMT = Epithelial-Mesenchymal Transition, MET = Mesenchymal-Epithelial Transition 46 The tumour microenvironment For your information Cancer cells are part of a complex microenvironment 47 Malignant potential? For your information Not all benign tumours become malignant Moles are examples of benign tumours where cells have undergone permanent growth arrest (senescence) A benign melanocytic tumour (non-cancerous) A melanoma 48 Lecture 1 Cancer Summary II Malignant tumours are distinguished from benign tumours by further phenotypic changes Progression factors Invasive capacity (via proteases & EMT) Angiogenesis Tumour invasion and metastasis occurs in a stepwise manner 1. Dysplasia 6. Arrest at new site 2. Formation of primary 7. Extravasation tumour 8. MET 3. EMT 9. Micrometastasis 4. Intravasation 10.Macrometastasis 5. Circulation 49 Example of a Quiz Question Describe two factors that affect tumour progression? 50 Star Wars Quotes - Yoda 51 BIOL340/981 Cell and Molecular Biology Cancer Week 8: Lecture 1 Dr Debbie Watson [email protected] Building 32.309 Consultation: Email for appointment School of Chemistry and Molecular Bioscience 1 Overview Lecture 1: Cancer Definition of cancer and nomenclature Causes of cancer Cancer initiation and progression Phenotypic (cellular) features of benign and malignant cancers Chapter 20 Cancer Molecular Biology of the Cell Textbook Lecture 2: Cancer Regulators of cancer progression Oncogenes Tumour suppressor genes Epigenetic changes in gene expression Multistep model of cancer progression Gene signatures Cancer therapeutics 2 Learning Objectives To understand the hallmarks of cancer To distinguish between benign and malignant cancer To describe different types of cancers To understand factors that can initiate cancer To understand cancer progression and metastases 3 What is cancer? A group of diseases ( > 277 types) characterised by a loss of normal cell growth control Normal cells Proliferate when required Undergo apoptosis when Damaged irreversibly After a finite number of cell divisions Cancer cells Do not respond to control mechanisms 4 Common hallmarks of cancer growth Altered homeostasis that results in cells growing and dividing at a faster rate than they die Bypass of normal limits to cell proliferation Evasion of cell-death signals Altered cellular metabolism Manipulation of the tissue environment to support cell survival and to evade a deleterious immune response Escape of cells from their home tissues and proliferation in foreign sites (metastasis) Ch 20 pg 1171 Molecular Biology of the Cell 5 Cancer cells have uncontrolled proliferation and metastasis Cancer cells share two fundamental properties oAbnormal cell growth and division (uncontrolled proliferation) oDefects in the normal restraints that keep cells from spreading and colonising other parts of the body (metastasis) In normal cells these processes are tightly controlled by genes (time and place) In cancer cells these genes are mutated or expressed inappropriately Cancer cells display altered control of growth and homeostasis Figure 20-14 Molecular Biology of the Cell 7 Cancer cells have uncontrolled proliferation and metastasis Source of Image https://www.coursehero.com/sg/cell-biology/mechanisms-of-cancer/ 8 The Hallmarks of Cancer 9 The Metabolic Pathways of Cancer 10 Benign tumour (neoplasm) Abnormally growing cells which remain as a single mass Non-cancerous Restricted within capsule of connective tissue Malignant tumour Tumour cells with the ability to invade through surrounding normal tissues (invasion) and spread to anatomically distant sites (metastasis) True cancer, responsible for > 90% of cancer deaths 11 Benign vs malignant cancers Normal glands Capsule of connective tissue Diffuse invasive cells No evidence of barriers Benign Malignant Adenoma (glandular Adenocarcinoma neoplasm) See similar Figure 20-3 Molecular Biology of the Cell 12 Cancer growth affects tissue function Normal skin Squamous cell carcinoma Connective tissue Connective tissue 13 Nomenclature Dependent upon site of origin Carcinoma Epithelial cell Adenocarcinoma (from a glandular neoplasm) Leukaemia, lymphoma, myeloma Hematopoietic cells Sarcoma Connective tissue Gliomas, retinoblastomas Central nervous system (CNS) 14 Example of a Quiz Question Describe the phenotypic differences between normal and tumour cells. 15 Cancer initiation and progression Cancer is a multistep process characterised by a progression of permanent alterations in cells 16 Initiation of cancer What factors influence initiation (and progression) of cancer? 1. 2. 3. 4. Environmental factors Infectious agents Family history (germ-line mutations) Age mutagens 17 1. Environmental factors Chemicals (carcinogens) Agents that cause simple local changes in nucleotide sequence E.g. smoking tobacco (lung cancer) Ionising radiation Chromosome breaks and translocations E.g. UV radiation (skin cancer) 18 2. Infectious agents Viruses DNA and RNA tumour viruses introduce foreign genetic material E.g. human papilloma virus (cervical cancer) Bacteria Produce toxins that disrupt cell signalling Induce chronic inflammation E.g. H. pylori (gastric cancer) 19 3. Inherited mutations Germ-line mutations Present in egg or sperm Cause cancer family syndrome Mutations in normal genes related to cell cycle controls Increased risk of specific types of cancer 20 Examples of Inherited mutations oColon cancer – tumour suppressor gene (APC) oRetinoblastoma – deletion (13q) oBreast cancer – genes that produce proteins that repair damaged DNA (BRCA1 & BRCA2) (5-10%) oLynch Syndrome - genes that affect DNA mismatch repair (MLH1) 21 3. Inherited mutations Most cancers are NOT hereditary Majority of human cancers result from an accumulation of somatic mutations Over 80 yrs, 1015 body cells replicate Random errors occur over a lifetime 22 4. Age Largest risk factor 23 Example of a Quiz Question What factors can affect tumour initiation 24 Tumour progression Progression can take years Mutant cells are selectively favoured over their neighbouring, normal cells 25 Tumour progression Subsequent mutations lead to more aggressive phenotypes 26 Tumour progression With the correct phenotype, tumour cells become malignant They can invade and metastasise* *Dissemination of cancer cells to anatomically distant organ sites and subsequent adaptation to foreign tissue microenvironments http://media.pearsoncmg.com/bc/bc_becker_woc_7/vids/html/24_2_TumorGrowth.html 27 Invasion and Metastasis Local invasion Metastasis 28 Metastatic spread Different cancer types exhibit remarkable variability in their metastatic course Liver of a breast cancer patient Length of the latency period or time to spread (months to years) Different organs affected (most commonly the liver, lung, bone, and brain) 29 Metastatic spread in GBM is rare Medicinal Research Reviews 2016 DOI 10.1002/med.21408 30 Brain cancer kills more children than any other disease 31 Tumour phenotype Key phenotypic features are shared by benign and malignant tumour cells Nuclear alterations Altered morphology & disorganised growth patterns Immortal 32 Phenotype vs genotype 33 Nuclear alterations Differences in nuclear morphology Papanicolaou (Pap) test Normal Abnormal cells in tissue (dysplasia) Invasive carcinoma 34 Nuclear alterations Aneuploidy (abnormal chromosome number) due to defect in mitotic spindle checkpoint Karyotype of a breast cancer cell showing a highly abnormal chromosome complement. 35 Altered morphology & disorganised growth Cancer cell proliferation is anchorage-independent and insensitive to population density Normal cells do not grow well in culture without a solid surface to attach to Stop dividing when they become confluent Tumour cells look different (cytoskeletal rearrangement) and grow in disordered multilayered clumps in vitro Contact inhibition Loss of contact inhibition 36 Immortality o Limitless replicative potential Cell number Cancer cells are no longer committed to die Tumour cells Only those tumors containing cells that maintain their telomere length will be capable of unlimited growth o 1. Any cell appearing within tumor that expresses telomerase will have a tremendous growth advantage over cells that lack it o 2. Over time, telomerase-containing cells will flourish, while those lacking it will die off; eventually, all of the cells in the tumor will contain telomerase Normal cells 1 2 3 4 Days in culture 37 Lecture 1 Cancer Summary I Benign tumour Abnormal cell growth, non-cancerous, confined to capsule Malignant tumour Can invade or metastasise, leading to cancer Tumours initiated through various factors Tumour progression caused by successive mutations, leading to an aggressive phenotype Benign and malignant tumours share some phenotypic features, but 38 Malignant tumours Malignant tumour cells can invade and metastasise This requires additional cellular alterations, or ‘progression factors’ Progression factors include Invasive capacity Enhanced expression of tissue degrading proteases Epithelial-Mesenchymal Transition (EMT) Together, enhance motility Angiogenesis Formation of new blood vessels 39 Invasive capacity: Enhanced expression of proteases 1. Cleaves cell surface proteins 2. Allows ECM breakdown 3. Activates growth factors or cytokines 1. 2. 3. 40 Invasive capacity: Epithelial-Mesenchymal Transition (EMT) 41 Malignant tumours are motile Increased cell motility is a fundamental characteristic of cancer cells Required in order for cells to invade through the basement membrane Represents an initial step in the metastatic cascade Necessary for cells to move from their primary organ of origin to distant metastatic sites MCF-7 (Green) non-invasive HT-1080 (Red) invasive 42 Angiogenesis Angiogenesis = formation of new blood vessels Feeds the tumour and provides a mode of transport 43 Angiogenesis Will NOT grow > 1-2 mm without blood supply 44 The metastatic cascade NB: angiogenesis not shown Arrest at new site Survival of CTCs in the circulation CTCs = Circulating Tumour Cells 45 The metastatic cascade 1. Dysplasia 2. Formation of primary tumour 3. EMT 4. Intravasation 5. Circulation 6. Arrest at new site 7. Extravasation 8. MET 9. Micrometastasis 10. Macrometastasis EMT = Epithelial-Mesenchymal Transition, MET = Mesenchymal-Epithelial Transition 46 The tumour microenvironment For your information Cancer cells are part of a complex microenvironment 47 Malignant potential? For your information Not all benign tumours become malignant Moles are examples of benign tumours where cells have undergone permanent growth arrest (senescence) A benign melanocytic tumour (non-cancerous) A melanoma 48 Lecture 1 Cancer Summary II Malignant tumours are distinguished from benign tumours by further phenotypic changes Progression factors Invasive capacity (via proteases & EMT) Angiogenesis Tumour invasion and metastasis occurs in a stepwise manner 1. Dysplasia 6. Arrest at new site 2. Formation of primary 7. Extravasation tumour 8. MET 3. EMT 9. Micrometastasis 4. Intravasation 10.Macrometastasis 5. Circulation 49 Example of a Quiz Question Describe two factors that affect tumour progression? 50 Star Wars Quotes - Yoda 51