Molecular Biology of the Cell Chapter 12 Intracellular Organization and Protein Sorting (Part 2) PDF
Document Details
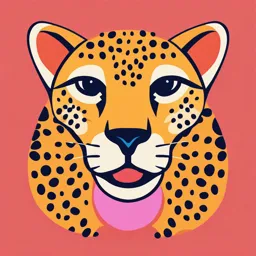
Uploaded by CalmFairy
جامعة البترا-الأردن & كلية الطب-جامعة الأزهر-مصر
Alberts, Bray, Hopkin, Johnson, Lewis, Raff, Roberts, and Walter
Tags
Related
- Protein Sorting at the Cytoplasm Lecture PDF
- Protein Sorting - BCCB2004 Lecture Notes (PDF)
- Protein Sorting (Part II) BCCB2004 PDF
- Molecular Cell Biology Week 4 Protein Sorting Mechanisms PDF
- Cell Biology - Intracellular Transport PDF
- Molecular Biology of the Cell Chapter 12 - Intracellular Compartments and Protein Sorting PDF
Summary
This is a chapter from the textbook "Molecular Biology of the Cell" seventh edition, focusing on the intracellular organization and protein sorting process, particularly within peroxisomes and mitochondria. The chapter details the mechanisms involved in protein transport within these organelles.
Full Transcript
Chapter 12 Intracellular Organization and Protein Sorting Part-2 Copyright © 2022 W. W. Norton & Company, Inc. PEROXISOMES Peroxisomes are major sites of oxygen utilization and are found in virtually all eukaryotic cells. They contain oxidative enzymes, such as catalase and urat...
Chapter 12 Intracellular Organization and Protein Sorting Part-2 Copyright © 2022 W. W. Norton & Company, Inc. PEROXISOMES Peroxisomes are major sites of oxygen utilization and are found in virtually all eukaryotic cells. They contain oxidative enzymes, such as catalase and urate oxidase, at such high concentrations. PEROXISOMES Peroxisomes use molecular oxygen and hydrogen peroxide to perform oxidation reactions. Peroxisomes contain one or more enzymes that use molecular oxygen to remove hydrogen atoms from specific organic substrates in an oxidation reaction that produces hydrogen peroxide (H2O2). Catalase uses the H2O2 generated by other enzymes in the organelle to oxidize a variety of substrates. Peroxisomes breakdown the fatty acid molecules. The process, called β oxidation, shortens the alkyl chains of fatty acids sequentially in blocks of two carbon atoms at a time, thereby converting the fatty acids to acetyl CoA. The peroxisomes then export the acetyl CoA to the cytosol for use in biosynthetic reactions. PEROXISOMES The proteins that compose peroxisomes are delivered by two different routes: 1- Integral membrane proteins of the peroxisomal membrane are first inserted into the ER.: These peroxisome-destined proteins are then packaged into specialized peroxisomal precursor vesicles. New precursor vesicles then fuse to form a new peroxisome or fuse with an existing peroxisome to facilitate its growth. 2- Peroxisomal proteins can be imported into preexisting peroxisomes directly from the cytosol: A specific sequence of three amino acids (Ser-Lys-Leu) located at the C-terminus of many peroxisomal proteins functions as an import signal PEROXISOMES Peroxisomal protein import is driven by ATP hydrolysis and utilizes a collection of proteins, called peroxins, that catalyze the import cycle. C-terminal peroxisomal sorting signals are recognized by the peroxin Pex5 in the cytosol. N-terminal peroxisomal signal sequences are recognized by the peroxin Pex7. The protein translocator in the peroxisomal membrane is composed of at least six different peroxins. Unlike protein translocators in the ER, the peroxisomal translocator can transport fully folded and even oligomeric proteins across the membrane. To allow the passage of large and variably sized cargo molecules, the transporter is thought to dynamically adapt in size to the particular cargo molecules to be transported. It is not known how such a large pore can be utilized for transport without leakage of contents between the cytosol and peroxisome. THE TRANSPORT OF PROTEINS INTO MITOCHONDRIA - Mitochondria is a double membrane–enclosed organelles. - It is a specialize in ATP synthesis, using energy derived from electron transport and oxidative phosphorylation - Although it contains its own DNA, ribosomes, and other components required for protein synthesis, almost all of their proteins are encoded in the cell nucleus and imported from the cytosol. - Each imported protein must reach the particular organelle sub-compartment in which it functions. The sub-compartments of mitochondria The different sub-compartments in mitochondria are formed by the two concentric mitochondrial membranes: - The inner mitochondrial membrane, which encloses the matrix space and forms extensive invaginations called cristae. - The outer mitochondrial membrane, which is in contact with the cytosol. - The intermembrane space which is the space between the inner and outer membranes, is subdivided into the crista space and intermembrane space, with protein complexes at the junctions where the cristae invaginate. Each of the sub-compartments in mitochondria contains a distinct set of proteins. New mitochondria is produced by the growth of preexisting organelles, followed by fission. THE TRANSPORT OF PROTEINS INTO MITOCHONDRIA Translocation into mitochondria depends on signal sequences and protein translocators. One or more signal sequences direct all mitochondrial precursor proteins to their appropriate mitochondrial sub-compartment. Many proteins entering the matrix space contain a signal sequence at their N-terminus that a signal peptidase rapidly removes after import. Other imported proteins, including all outer membrane and many inner membrane and inter-membrane space proteins, have internal signal sequences that are not removed. The signal sequences are both necessary and sufficient for the import and correct localization of the proteins The protein translocators in the mitochondrial membranes. Multi-subunit protein complexes that function as protein translocators mediate protein movement across or into mitochondrial membranes. Protein translocator complexes are located in both the inner and outer mitochondrial membranes. They have the capacity to recognize particular types of signals. These translocators direct ∼1500 different precursor proteins from the cytosol to the appropriate sub-compartment of mitochondria. The protein translocators in the mitochondrial membranes. The organization of signals in a precursor protein ultimately controls which translocator(s) the precursor protein engages and the order in which the signals are used to reach the protein’s final destination inside mitochondria. The protein translocators in the mitochondrial membranes. SAM 5 sorting and assembly machinery; insertion and deletion OXA 5 cytochrome oxidase activity; TIM 5 translocator of the inner mitochondrial membrane; TOM 5 translocator of the outer membrane; MIM 5 mitochondrial import machinery. The TOM complex is required for the import of nearly all nucleus-encoded mitochondrial proteins. It initially recognizes their signal sequences and transports them from the cytosol into the intermembrane space. Then each mitochondrial proteins follow different itineraries depending on sequence features encoded in the protein. The protein translocators in the mitochondrial membranes. SAM 5 sorting and assembly machinery; OXA 5 cytochrome oxidase activity; TIM 5 translocator of the inner mitochondrial membrane; TOM 5 translocator of the outer membrane; MIM 5 mitochondrial import machinery. Some proteins are synthesized by mitochondrial ribosomes and inserted into the inner membrane by the OXA complex. Mitochondrially encoded membrane proteins are assembled with nuclear encoded membrane proteins imported from the cytosol to form functional protein complexes such as the respiratory chain complexes used for energy production. Protein import by mitochondria. The protein translocators in the mitochondrial outer membrane do not bind to ribosomes, and most mitochondrial proteins are imported by a post-translational mechanism. Mitochondrial precursor proteins do not immediately fold into their native structures after they are synthesized; instead, some interact with chaperones of the hsp70 family, whereas others are dedicated to mitochondrial precursor proteins and bind directly to their signal sequences. All the interacting proteins help to prevent the precursor proteins from aggregating or folding up spontaneously before they engage with the TOM complex in the outer mitochondrial membrane. Protein import by mitochondria. Once the translocating protein protrudes into the intermembrane space, sequences within the polypeptide chain determine what happens next. For example, proteins destined for the matrix or inner membrane engage one of the TIM complexes and are either translocated across or inserted into the inner membrane. Although the TOM and TIM complexes usually work together to translocate precursor proteins across both membranes at the same time, they are capable of operating independently. The role of energy in protein import into mitochondria Mitochondrial protein import utilizes three different sources of energy at four discrete sites: 1- ATP (used at two of these sites: outside the mitochondria and inside the matrix). 2- Membrane potential across the inner mitochondrial membrane. 3- The redox potential of the electron transport chain. Not all mitochondrial precursor proteins need each of these energy sources to arrive at their final destination. The initial use of energy is needed to maintain the polypeptide in an unfolded state prior to import (Chaperons interactions with the polypeptides). The role of energy in protein import into mitochondria : Energy from membrane potential Once the signal sequence has passed through the TOM complex and is bound to a TIM complex, further translocation through the TIM translocation channel requires the membrane potential (the electrical component of the electrochemical H+ gradient across the inner membrane). Pumping of H+ from the matrix space to the intermembrane space, driven by electron transport processes in the inner membrane maintains the electrochemical gradient. The energy in the electrochemical H+ gradient across the inner membrane drives the translocation of the positively charged signal sequences through the TIM complexes by electrophoresis. The same H+ gradient also powers most of the cell’s ATP synthesis by ATP synthase complexes in the inner mitochondrial membrane. The role of energy in protein import into mitochondria: Energy from ATP hydrolysis Mitochondrial hsp70 has a high affinity for unfolded polypeptide chains, and it binds tightly to an imported protein chain as soon as the chain emerges from the TIM translocator in the matrix space. The hsp70 then undergoes an ATP-dependent conformational change that exerts a pulling force on the protein being imported before releasing it. The energy driven cycle of binding, pulling, and release continues until the protein has completed import through the TIM23 complex. Many imported matrix proteins are passed on to another chaperone protein, mitochondrial hsp60, to assist their folding through cycles of ATP hydrolysis The role of energy in protein import into mitochondria: Energy from redox potential). Certain inter-membrane space proteins that contain cysteine motifs use the difference in redox potential between the cytosol and mitochondria as a source of energy. When a portion of these proteins initially emerges into the intermembrane space, they form a transient covalent disulfide bond to the Mia40 protein. This interaction prevents the backsliding of the protein through the TOM complex into the cytosol. The imported proteins are eventually released from Mia40 in an oxidized form containing intrachain disulfide bonds, resulting in a folded protein that is now trapped in the intermembrane space. Mia40 becomes reduced in the process and is then reoxidized by passing electrons to the electron transport chain in the inner mitochondrial membrane. In this way, the energy stored in the redox potential in the mitochondrial electron transport chain is tapped to drive protein import.