Topic 2 Bacteria Students PDF
Document Details
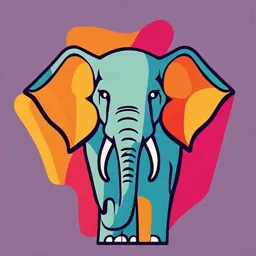
Uploaded by GratifyingHyperbolic3802
University of Waterloo
Tags
Related
- Archaea Cell Biology PDF
- Microbiology - Week 5 - Bacterial Cell Biology PDF
- L14 Basic Biology of Bacteria Maulik 2024 PDF
- Microbiology Chapter 5: Cell Biology of Bacteria and Eukaryotes PDF
- Microbiology L2 Basic Structure of Bacteria PDF
- Lectures 2,3 Bacterial Cell Structure (Medical Microbiology) PDF
Summary
This document presents an overview of bacterial morphology, covering various shapes, sizes, and internal structures of bacterial cells. It discusses the components of the bacterial cell, including the cytoplasm, cytoskeleton, cell envelope, and cell surface, providing details about their functions and structures. The document also touches upon bacterial cell sizes and describes the advantages to being a small cell.
Full Transcript
Topic 2 | Bacteria Topic Overview Morphology Cell sizes Cytoplasm Cytoskeleton Cell envelope Cell surface Taxonomy Morphology of Bacterial Cells Bacteria can take many different shapes spherical (s. coccus, pl. cocci) Figure 2.1 A Morp...
Topic 2 | Bacteria Topic Overview Morphology Cell sizes Cytoplasm Cytoskeleton Cell envelope Cell surface Taxonomy Morphology of Bacterial Cells Bacteria can take many different shapes spherical (s. coccus, pl. cocci) Figure 2.1 A Morphology of bacterial cells rod-shaped Figure 2.1 B (s. bacillus, pl. bacilli) comma-shaped (s. vibrio, pl. vibrios) Figure 2.1 C Morphology of bacterial cells Figure 2.1 D spiral (s. spirillum, pl. spirilla) pleiomorphic (varied shapes) Figure 2.2 Morphology of Bacterial Cells Generally not a good predictor of physiology, ecology, or phylogeny Morphology may be determined by selective forces nutrient uptake efficiency (surface-to-volume ratio) spirals allow efficient swimming in viscous or turbulent fluids (i.e., near surfaces) gliding motility (filaments) Morphology of Bacterial Cells Some bacteria can also assume multicellular organizations hyphae (branching filaments of cells) mycelia (tufts of hyphae) trichomes (smooth unbranched chains of cells) Figure 2.3 Cell Sizes Prokaryotes are 0.2 μm to > 700 μm in length/diameter most rod-shaped bacteria between 0.5 μm-4.0 μm wide and 1-15 μm long very few “large” prokaryotes Eukaryotic cells range from 10 μm to >200 μm Minimum size simply due to minimum space requirements for genome, proteins, ribosomes Figure 2.4 Cell Sizes There are exceptions to the general size of bacterial cells Thiomargarita namibiensis: up to 700 μm in diameter! Epulopiscium fishelsoni: Figure 2.5 200‒700 μm x 80 μm! Epulopiscium fishelsoni (“guest at a banquet of a fish”) From gut of surgeon fish Uncultured identified by 16S rRNA sequence related to Clostridium 700 μm long compared to eukaryotic Paramecium: 75 μm Figure 3.2 Figure 3.2 Thiomargarita namibiensis Heide Schulz-Vogt, 700-μm diameter Advantages to Being Small Higher surface-to- volume ratio greater rate of nutrient/waste exchange per unit volume supports higher metabolic rate supports faster growth rate, faster evolution Why be big? Figure 3.3 (Madigan 13th) Lower Size Limits Size reduction constrained by the minimum complement of cellular structures Diameters < 0.15 μm unlikely “Very small” cells common in open marine environments 0.2 μm to 0.4 μm common Scale bars = 100 nm Cells are ~ 200 nm (or 0.2 μM) across, at the limit of cell size! What is in the cytoplasm of bacteria? Figure 2.6 largest area is the nucleoid region houses the chromosome(s) and DNA replication machinery How does DNA compress within the nucleoid of bacteria? several mechanisms to reduce space – use of cations (Mg2+, K+, Na+) to shield negative charges on sugar-phosphate (PO4-) backbone – small, positively charged proteins bind to the chromosome to maintain condensed structure – topoisomerases modify structure of DNA to enable “supercoiling” No membrane surrounds the nucleoid No histone proteins (like those found in Archaea and Eukaryotes) remainder of the cytoplasm is a “stew” of macromolecules (e.g., tRNA, rRNA, mRNA, proteins, ribosomes) inclusion bodies and microcompartments may also be present sulfur globules: sulfur storage for energy polyhydroxybutyrate granules: carbon storage gas vesicles: buoyancy control carboxysomes: location of carbon fixation reactions (RUBISCO) magnetosomes: organelle associated with direction finding remainder of the cytoplasm is a “stew” of macromolecules (e.g., tRNA, rRNA, mRNA, proteins, ribosomes) inclusion bodies and microcompartments may also be present sulfur globules: sulfur storage for energy polyhydroxybutyrate granules: carbon storage gas vesicles: buoyancy control carboxysomes: location of carbon fixation reactions (RUBISCO) magnetosomes: organelle associated with direction finding carboxysomes Figure 2.7 gas vesicles Figure B2.1 Bacterial cytoskeleton a series of internal proteins assist in keeping everything in the right place Some cytoskeleton proteins are involved in cell wall synthesis during cell division MreB (homolog of actin – microfilaments) FtsZ (homolog of tubulin - microtubules) Figure 2.8 Other cytoskeletal proteins are involved in moving internal items e.g., plasmids Figure 2.8 C CELL ENVELOPE All layers surrounding the cytoplasm of cells, which includes the cell membrane (plasma membrane), cell wall, and outer membrane (if present). Cytoplasmic membrane all cells have a plasma membrane separates the interior of the cell from the external environment Figure 2.9 Roles of the plasma membrane Capturing energy electron transport chains create proton motive force (PMF) can be used for respiration/photosynthesis can be used to derive energy for motion (flagella) Holding sensory systems embedded proteins can detect environment changes, alter gene expression in response Permeability barrier (but not structural) usually composed of a phospholipid bilayer with embedded proteins hydrophobic core hydrophilic surfaces interact with either the external environment or the cytoplasm Figure 2.9 B chemically variable due to changes in fatty acid groups attached to a glycerol backbone connected by ester linkages Ester Figure 2.1 A May have sterol-like molecules called “hopanoids” in it to help with stability across temperature ranges. Figure 2.10 A How do items cross the plasma membrane? O2 and CO2 are small and can diffuse across readily H2O is helped across by aquaporin protein channels (osmosis) Facilitated diffusion and co-transport protein channel moves particles by leveraging a concentration gradient no ATP energy required Co-transport is a Figure 2.11 form of “active transport” - energy Active transport example #2 – ABC transporters ATP-Binding Cassette protein transporter moves particles AGAINST a concentration gradient requires energy in form of ATP Figure 2.1 A Protein secretion shipping proteins outside the cell uses ATP energy e.g., toxins, siderophores, enzymes involved in breaking down substrates Figure 2.13 Roles of the Plasma Membrane Capturing energy electron transport chains create proton motive force (PMF) can be used for respiration/photosynthesis can be used to derive energy for motion (flagella) Holding sensory systems embedded proteins can detect environment changes, alter gene expression in response Permeability barrier (but not structural) Cell wall gives cells their shape protects from osmotic lysis/mechanical forces a matrix of crosslinked strands of peptidoglycan subunits Figure 2.14 A Peptidoglycan subunits N-acetylmuramic acid (NAM) small peptide chain N-acetylglucosamine (NAG) Figure 2.14 B Amine Acetyl Peptides and peptide crosslinks vary by species Gram-negative Gram-positive Figure 2.15 Wikipedia Commons Several of the amino acids found associated with NAM in peptidoglycan are unusual D forms d forms are stereoisomers (mirror images) of the l forms normally found in biological proteins Figure 2.16 Transglycosylation Transpeptidation (FtsI) Divisome associated with FtsZ Figure 2.17 Cell Wall Formation The cell wall can be degraded lysozyme and lysostaphin secretions Figure 2.18 Without the cell wall the cell cannot resist osmotic pressure changes Figure 2.18 C Osmosis is the flow of water across the plasma membrane toward the side with a higher solute (particle) concentration. Osmosis can cause a cell to swell with water or shrivel as water leaves, but a strong cell wall can help keep a bacterial cell alive during these hardships. b-lactam I tra nhibit nsp s F ept tsI antibiotics ida tion prevent peptidoglycan crosslinking Figure 2.19 Antibiotic resistance Some bacteria can produce an enzyme to destroy the critical b-lactam ring structure second drug must be added to inhibit the enzyme Figure 2.20 Gram-positive cells thick outer layer of peptidoglycan narrow periplasmic space negatively charged teichoic acids in the peptidoglycan Figure 2.21 Gram-negative cells very thin layer of peptidoglycan periplasmic space of varying width outer membrane composed of lipopolysaccharide (LPS) Figure 2.22 LPS from Gram-negative cells can be harmful Lipid A O (outer) side chain of polysaccharides can vary dramatically changed by the microbe to evade host immune responses Figure 2.23 Not all cell wall structures are the same stain method developed by Hans Christian Gram in 1884 separates cells into 2 classes: Gram-positive and Gram-negative The Gram-Stain Alcohol decolourization shrinks large pores in Gram-positive cells helps lock the crystal violet stain in Alcohol removes outer membrane lipids in Gram-negative cells more likely to lose the initial crystal violet stain Positive (Purple) Negative (red) How do nutrients get through the cell wall? Gram-positive peptidoglycan layer has large pores throughout its matrix Gram-negative cells have porins and TonB proteins in the outer membrane – transfer molecules into the periplasmic space – TonB uses proton motive Figure 2.24 force across cytoplasmic membrane Figure 2.25 How can molecules get out of a Gram-negative cell’s periplasmic space? some move from the periplasm to outside directly (these are known as autotransporters and are rare) some use single-step (never entering the periplasm) transport systems The Bacterial Cell Surface Flagella spiral, hollow, rigid filaments extending from the cell surface locations and number vary across species Figure 2.26 Flagella spiral, hollow, rigid filaments extending from the cell surface locations and number vary across species Figure 2.26 Composed of three basic pieces 1. filament of multiple flagellin proteins 5‒10 μm long 2. hook protein portion connects filament to basal body 3. basal body disk-like structure that turns filament like a propeller Figure 2.26 Energy to spin flagella derived from proton motive force (PMF) complex structures spinning creates “runs” and “tumbles” expensive: ~1000 H+ translocated per rotation! Figure 2.27 Chemotaxis Chemoreceptor proteins temporally sense changes in concentrations of attractants or repellents Figure 2.28 Cells can have internal flagella Some spirochetes have flagella in the periplasm as they spin, they rotate the entire cell body like a corkscrew Figure 2.29 Nonflagellar motility Gliding motility smooth sliding over a surface, not well understood e.g., Myxobacteria, Cyanobacteria Twitching motility slow, jerky process using pili that extend, attach to, and pull along a surface e.g., Pseudomonas aeruginosa Polymerization of actin in host cells for propulsion of bacteria into adjacent cells e.g., Shigella dysenteriae, Listeria monocytogenes Figure 2.31 Adherence molecules allow cells to stick to surfaces pili (s. pilus), fibers of pilin protein, possess other proteins on their tips for sticking Sex pilus A sex pilus is a different Figure 2.32 structure used for conjugation (sending a plasmid from one cell to another). Adhesive pili (“fimbriae”) Stalk Some microbes will use an extension of the cell envelope, tipped by a “holdfast” of polysaccharides Provide extra surface area for nutrient absorption e.g., Caulobacter, Figure 2.33 Hyphomonas Capsules Thick layer of polysaccharides surrounding some cells (both G+’ve and G-’ve) provide adhesion, defense against host immunity, protection against desiccation Figure 2.34 Capsules can help bacteria form biofilms Provide protection and enhanced survivability in harsh environments Figure 2.35 Surface arrays (S-layers) crystalline array of interlocking proteins can protect a cell against predation or infection with bacteriophages found in both Gram-positive and Gram-negative cells (and Archaea!) Figure 2.36 Bacterial taxonomy most microbes still can’t be cultured! what can be grown are named according to the standard binomial system: Species: group of strains sharing common features, while differing considerably from other strains Genus: group of closely related species Above the genus level, family, order, class, phylum, and domain are used Prince Charles Orders Fishy Green Soup Classification depends on many features: DNA sequence data size/shape Gram type colony morphology presence of structures such as capsules or endospores (SEE LEARN ANNOUNCEMENT) physiological/metabolic traits once classified, microbes are deposited in at least two culture collections the World Federation for Culture Collections maintains a database of more than 500 collections from over 60 countries pure, maintained cultures made available to scientists for research purposes a “Type strain” is a referenced specimen deposited in a culture repository. new info Only ~1% cultured Coming later: “Bacterial MVPs” (need a little metabolism first)