Microbiology PDF
Document Details
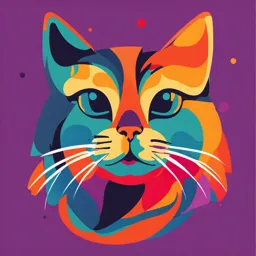
Uploaded by TopRapture
University of Liberia
Alan N Korlison
Tags
Summary
This document, prepared by Mr. Alan N Korlison, provides a comprehensive overview of microbiology including discussions of microbiology, the history, bacteria cell morphology and its components, the impact of bacteria on a variety of areas.
Full Transcript
DEPARTMENT OF BIOLOGY TJR FAULKNER COLLEGE OF SCIENCE AND TECHNOLOGY UNIVERSITY OF LIBERIA General Microbiology (301) Section (10) Prepared by: Instructor, Mr. Alan N Korlison MSc. Microbiology ...
DEPARTMENT OF BIOLOGY TJR FAULKNER COLLEGE OF SCIENCE AND TECHNOLOGY UNIVERSITY OF LIBERIA General Microbiology (301) Section (10) Prepared by: Instructor, Mr. Alan N Korlison MSc. Microbiology Introduction Microbiology is the study of living organisms of microscopic size. It is an interdisciplinary scientific study of microorganism encompassing their taxonomy, physiology, genetics, ecology and biochemistry. It examines the complex interaction between microbes and their environment including their role in health disease and biotechnological applications. Medical microbiology is the subdivision concerned with the causative agents of infectious disease of man, the response ofthe host to infection and various methods of diagnosis, treatment and prevention. The term microbe was first used by Sedillot in 1878, but now is commonly replaced by microorganism. Infection and contagion Ancient Belief Among ancient peoples, epidemic and even endemic diseases were believed to be supernatural in origin, sent by the gods as punishment for the sins of human kind. Sacrifices and lustrations to appease the anger of the gods were sought for the treatment and, more importantly, the prevention of disease. There was never any difficulty in finding particular sets of sins to justify a specific epidemic since humans are wilful and wanton by nature. Concept of Contagion existed long before microbes had been seen, observations on communicable diseases had given rise to the concept of contagion: The spread of disease by contact, direct or indirect. This idea was implicit in the laws enacted in early biblical times to prevent the spread of leprosy. Discovery of microorganisms Invisible Living Creatures Produced Disease, Varro in the second century BC later recorded the principle of contagion by invisible creatures. Roger Bacon, in the thirteenth century more than a millennium later, postulated that invisible living creatures produced disease. Fracastorius (1546), a physician of Verona concluded that communicable diseases were caused by living agents (germs) ‘seminaria’ or ‘seeds’. Kircher (1659) reported finding minute worms in the blood of plague victims, but with the equipment available to him, it is more likely that what he observed were only blood cells. Von Plenciz (1762) suggested that each disease was caused by a separate agents. Even before microorganisms were seen, some investigators suspected their existence and responsibility for disease. Among others, the Roman philosopher Lucretius (about 98-55 BC) and the physician Girolamo Fracastoro (1478-1553) suggested that disease was caused by invisible living creatures. Direct observation of microorganisms had to await the development of the microscope. Antoni van Leeuwenhoek (1632-1723) the credit for having first observed and reported bacteria belongs to Antony van Leeuwenhoek. Antoni van Leeuwenhoek, the Dutchman, was a draper and haberdasher in Delft, Holland. He had little education, but great patience and curiosity. His hobby was grinding lenses and observing diverse materials through them. He was the amateur microscopist and was the first person to observe microorganisms (1673) using a simple microscope. In 1683 he made accurate descriptions of various types of bacteria and communicated them to the Royal Society of London. Their importance in medicine and in other areas of biology came to be recognized two centuries later. Conflict over spontaneous generation Spontaneous Generation (Abiogenesis) from earliest times, people had believed in spontaneous generation that living organisms could develop from nonliving matter. Even great Aristotle (384- 322 BC) thought animal could originate from the soil. This view was finally challenged by the Italian physician Francesco Redi (1626-1697) and proved that gauze placed over jar containing meat prevented maggots forming in the meat. Similar experiments by others helped discredit the theory for larger organisms. Some proposed that microorganisms arose by spontaneous generation though larger organisms did not. John Needham (1713-1781 the English priest) in 1745, published experiments purporting the spontaneous generation (abiogenesis) of microorganisms in putrescible fluids. Felix Pouchet (1859), the French naturalist, claimed to have carried out experiments conclusively proving that microbial growth could occur without air contamination. This claim provoked Louis Pasteur (1822-1895) to settle the matter once and for all. Lazzaro Spallanzani (1729-1799), an Italian priest and naturalist also opposed spontaneous generation he boiled beef broth for an hour, sealed the flasks, and observed no formation of microbes. Franz Schulze (11815-1873), Theodore Schwann (1810- 1882), Georg Friedrich Schroder and Theodor von Dutch attempted to counter such arguments. Louis Pasteur (1822- 1895) settled the matter once and for all. In a series of classic experiments, Pasteur proved conclusively that all forms of life, even microbes, arose only from their like and not de novo. Pasteur was able to filter microorganisms from the air and concluded that this was the source of contamination and finally, in 1859, in public controversy with Pouchet, prepared boiled broth in flasks with long narrow gooseneck tubes that were open to the air. Air could pass but microorganisms settled in the gooseneck, and no growth developed in any of the flasks. If the necks were broken, growth commenced immediately. Pasteur had not only resolved the controversy by 1861 but also had shown how to keep solution sterile. Contributions of Antoni von Leeuwenhoek He constructed the first microscope: Consisted of a single biconvex lens that magnified about x200. The first person to observe microorganisms: Microorganisms were first seen by Antoni van Leeuwenhoek (1673) and he found many microorganisms in materials such as water, mud, saliva and the intestinal contents of healthy subjects, and he recognized them as living creatures (animalcules) and to Leeuwenhoek the world of “little animalcules” represented only a curiosity of nature. Accurate description of bacteria: He first accurately described the different shapes of bacteria as cocci (spheres), bacilli (rods) and spirochetes (spiral filaments) and communicated them to the Royal Society of London in 1683 Contributions of Louis Pasteur in Microbiology 1. Coined the term microbiology. 2. Proposed germ theory of disease. 3. Disapproved theory of spontaneous generation. 4. Developed sterilization techniques. 5. Developed methods and techniques for cultivation of microorganisms. 6. Studies on pebrine (silk worm disease), anthrax, chicken cholera and hydrophobia. 7. Pasteurization. 8. Coined the term vaccine. 9. Discovery of attenuation and chicken cholera vaccine. 10. Developed live attenuated anthrax vaccine. 11. Developed rabies vaccine—The crowning Robert Koch Robert Koch (1843-1910) was a German physician, the first direct demonstration of the role of bacteria in carrying disease came by the study of anthrax by Koch. Winner of the Nobel Prize in 1905, Robert Koch is known as “Father of bacteriology” Contributions of Robert Koch: 1. Staining techniques: He described methods for the easy microscopic examination of bacteria in dried, fixed films stained with aniline dyes (1877). 2. Hanging drop method: He was the first to use hanging drop method by studying bacterial motility 3. Methods for isolating pure cultures of bacteria: He devised a simple method for isolating pure cultures of bacteria by plating out mixed material on a solid culture medium and to isolate pure cultures of pathogens. 4. Discoveries of the causal agents of anthrax (1876), tuberculosis (1882), and cholera (1883). 5. Koch’s phenomenon: Koch (1890) observed that a guinea pig already infected with the bacillus responded with an exaggerated response when injected with the tubercle bacillus or its protein. This hypersensitivity reaction is known as Koch’s phenomenon Koch’s postulates: It was necessary to introduce criteria for proving the claims that a microorganism isolated from a disease was indeed the causative agent related to it. Robert Koch proved that microorganisms cause disease. Koch used the criteria proposed by his former teacher, Jacob Henle (1809-1885), to establish the relationship between Bacillus anthracis and anthrax and published his findings in 1876. His criteria for proving the causal relationship between a microorganism and a specific disease are known as Koch’s postulates (1876), which are used today to prove that a particular microorganism causes a particular disease Koch’s postulate` It states that in order for a microorganism to be considered causative agent of a disease, the identified organism must (1) Be present in all cases of the disease absent from healthy persons. (2) Be isolated from diseased patients; (3) Cause disease when reintroduced to a healthy susceptible animal model (4) Then be isolated again from the new host. Limitations of Koch’s postulates: Even today Koch’s postulates are considered whenever a new infectious disease arises. However these criteria have proved invaluable in some instances in identifying pathogens, because they cannot always be met, for example, some organisms (including all viruses) cannot be grown on artificial media, and some are pathogenic only for men. Mycobacterium leprae, a causative agent of leprosy, has not been cultured on artificial medium so far and not fulfilling Koch’s postulates. Paul Ehrlich Paul Ehrlich, an outstanding German Scientist and genius of extraordinary activity also known as “Father of chemotherapy”. Contributions of Paul Ehrlich 1. Stains to cells and tissues: He applied stains to cells and tissues for the purpose of revealing their function. 2. Acid-fastness of tubercle bacillus: He reported the acid-fastness of tubercle bacillus. 3. Methods of standardizing toxin and antitoxin: He introduced methods of standardizing toxin and antitoxin and coined the term minimum lethal dose. 4. Side chain theory of antibody production: He proposed side chain theory of antibody production, including antibody reaction leading to better understanding of the immune system. 5. Salvarsan introduction: He introduced salvarsan, an arsenical compound, sometimes called the ‘magic bullet’. It was capable of destroying the spirochete of syphilis with only moderate toxic effects. He continued his experimentation until 1912 when he announced the discovery of neosalvarsan. Role of microorganisms in disease A firm basis for the casual nature of infectious disease was established only in the latter half of the nineteenth century. Fungi, being larger than bacteria, were the first agents to be recognized.Agostino Bassi (1773-1856) demonstrated in 1835 that a silkworm disease called muscardine was due to a fungal infection. MJ Berkeley (1845) proved that the great potato blight of Ireland was caused by a fungus. Following his success with the study of fermentation, Pasteur was asked by French government to investigate the pebrine disease of silkworm that was disrupting the silk industry. He showed that the disease was due to a protozoan parasite after several years of work. Empirical Observations and the etiologic role of bacteria was first established with anthrax. Pollender (1849) and Davaine (1850) observed anthrax bacilli in the blood of animals dying of the disease. Type of microorganisms The classification of microorganisms into groups such as bacteria archaea,fungi,protozoa,algae and viruses has evolved over time, primilarly through the work of scientists like carl woese who introduced the three domain system,(bacteria archaea and eukarya) Robert Whittaker five kingdom classification system ( Animalia ,Plantae ,fungi Protista monera )including many other scientists who works paved the way for the better understanding of microorganisms. Microscope Microscope is an optical instrument used to magnify (enlarge) small objects or microorganisms which cannot be seen by naked eye. The study of the morphology of bacteria requires the use of microscopes. In general, microscopy is used in microbiology for two basic purposes: the initial detection of microbes and the definitive identification of them. 1. Light microscopy refers to the use of any kind of microscope that uses visible light to observe specimens. Here we examine several types of light microscopy. a. Bright-field microscope/Compound light microscope Bright field microscopy, also known as a compound light microscopy, uses light to illuminate a sample and create an image. The sample is placed on a glass slide and illuminated by a light source, typically a halogen lamp or a light-emitting diode (LED) light. The light passes through the sample, and an objective magnifies the image and projects it onto an eyepiece or a camera. The sample appears dark against a bright background, hence the name “bright field.” b. Dark-field microscopy__ Dark-field microscopy is frequently performed on the same microscope on which bright-field microscopy is performed. Instead of the normal condenser, a dark-field microscope uses a dark-field condenser that contains an opaque disk. The disk blocks light that would enter the objective lens directly. Only light that is reflected off (turned away from) the specimen enters the objective lens. Because there is no direct background light, the specimen appears light against a dark background. This creates a “dark-field” that contrasts against the highlighted edge of the specimens and results when the oblique rays are reflected from the edge of the specimen upward into the objective of the microscope. Using this technique is particularly important for observing organisms such as Treponema pallidum, a spirochete which is less than 0.2 mm in diameter and therefore cannot be observed with direct light. The disadvantage is that internal structure of organisms cannot be studied because light passes around rather than through organisms. c. Phase-contrast microscopy. The principle of phase-contrast microscopy is based on the wave nature of light rays, and the fact that light rays can be in phase (their peaks and valleys match) or out of phase. In a phase-contrast microscope, one set of light rays comes directly from the light source. The other set comes from light that is reflected or diffracted from a particular structure in the specimen. (Diffraction is the scattering of light rays as they “touch” a specimen’s edge. The diffracted rays are bent away from the parallel light rays that pass farther from the specimen). When the two sets of light rays-direct rays and reflected or diffracted rays are brought together, they form an image of the specimen on the ocular lens, containing areas that are relatively light (in-phase), through shades of gray, to black (out of phase). Through the use of annular rings in the condenser and the objective lens, the differences in phase are amplified so that in-phase light appears brighter than out-of-phase light. The special phase condenser consists of annular diaphragms on a rotating disk fitted to the bottom of the condenser. Advantage Phase-contrast microscopy improves the contrast, the internal structures of a cell become more sharply defined and makes evident the structures within cells that differ in thickness or refractive index. Uses 1. 1.To study unstained living cell 2. Detailed examination of internal structures in living microorganisms. 3. To study flagellar movements and motility of bacteria and protozoans 4. To study intestinal and other live protozoa such as amoebae and Trichomonas 5. To examine fungi grown in culture. d. Fluorescent microscopy Fluorescence microscopy takes advantage of fluorescence, the ability of substances to absorb short wavelengths of light (ultraviolet) and give off light at a longer wavelength (visible). If tissues, cells or bacteria are stained with a fluorescent dye and are examined under the microscope with ultraviolet light instead of ordinary visible light, they become luminous and are seen as bright objects against a dark background. The color that the cells will appear depends on the type of dye and the light filters. The fluorescence microscope is used to observe cells or other material that are either naturally fluorescent or have been stained or tagged with fluorescent dyes e. Confocal Microscopy In confocal microscopy, lenses focus a laser beam to illuminate a given point on one vertical plane of a specimen. Like fluorescent microscopy, specimens are stained with fluorochromes so they will emit, or return light. Mirrors then scan the laser beam across the specimen, illuminating successive regions and planes until the entire specimen has been scanned. Each plane corresponds to an image of one fine slice of the specimen. A computer then assembles the data and constructs a three-dimensional image, which is displayed on a screen. In effect, this microscope is a miniature CAT scan for cells. Frequently, the specimens are first stained or tagged with a fluorescent dye. By using certain fluorescent tags that bind specifically to a given protein or other compound, the precise cellular location of that compound can be determined. In some cases, multiple different tags that bind to specific molecules are used, each having a distinct color. Uses I. To obtain three-dimensional images of entire cells and cellular components ii. To evaluate cellular physiology—by monitoring the distributions and concentrations of substances such as ATP and calcium ions 2. Electron microscopy __ Electron microscopy is in some ways comparable to light microscopy. Rather than using glass lenses, visible light, and the eye to observe the specimen, the electron microscope uses electromagnetic lenses, electrons, and a fluorescent screen to produce the magnified image (figure). That image can be captured on photographic film to create an electron photomicrograph. The superior resolution of the electron microscope is due to the fact that electrons have a much shorter wavelength than the photons of white light. The electron beam is focused by circular electromagnets, which are analogous to the lenses in the light microscope. The object which is held in the path of the beam scatters the electrons and produces an image which is focused on a fluorescent viewing screen A.Transmission electron microscope (TEM).In a transmission electron microscope, electrons pass through the specimen and are scattered. Magnetic lenses focus the image onto a fluorescent screen or photographic plate; B. scanning electron microscope (SEM). In a scanning electron microscope, primary Electrons sweep across the specimen and knock electrons from its surface. These secondary electrons are picked up by a collector, amplified, and transmitted onto a viewing screen or photographic plate There are two types of electron microscopes in general use: Transmission Electron Microscope (TEM) and Scanning electron microscope (SEM) Magnification. In microscopy magnification is the process of enlarging the appearance of an object or specimen. We can calculate the total magnification of a specimen by multiplying the objective lens magnification (power) by the ocular lens magnification (power). Three different objective lenses are commonly used: low power × 10); high dry × 40); and oil immersion × 100). Most oculars magnify specimens by a factor of 10. Multiplying the magnification of a specific objective lens with that of the ocular, we see that the total magnifications would be 100 × for low power, 400 × for high power, and 1000 × for oil immersion. Resolution The limitation of bright-field microscopy is the resolution (also called resolving power) of the image (i.e. the ability to distinguish that two objects are separate and not one). The resolving power of a microscope is determined by the wavelength of light used to illuminate the subject and the angle of light entering the objective lens (referred to as the numerical aperture). A general principle of microscopy is that the shorter the wavelength of light used in the instrument, the greater the resolution. Immersion Oil The white light used in a compound light microscope has a relatively long wavelength and cannot resolve structures smaller than about 0.2 µm. To achieve high magnification (100 ×) with good resolution, the objective lens must be small. Immersion oil is placed between the glass slide and the oil immersion objective lens. The immersion oil has the same refractive index as glass, so the oil becomes part of the optics of the glass of the microscope. The oil enhances the resolution by preventing light rays from dispersing and changing wavelength after passing through the specimens. A specific objective lens, the oil immersion lens, is designed for use with oil; this lens provides 100 × magnification on most light microscopes. Comparison b/w prokaryotic and eukaryotic cells All living organisms on earth are composed of one or the other of two types of cells: prokaryotic cells and eukaryotic cells based on differences in cellular organization and biochemistry. Prokaryotes: Bacteria and Archaea. Eukaryotes: Eucarya. 1. Prokaryotes: Prokaryotic cells (pro or primitive nucleus) do not have a membrane-bound nucleus. All bacteria and blue-green algae are prokaryotes. Bacteria are prokaryotic microorganisms and don’t contain chlorophyll but in contrast, blue green algae possess chlorophyll. They are unicellular and do not show true branching, except in the so called ‘higher bacteria’ (Actinomycetales). Eukaryotes: Eukaryotic cells (eu or true nucleus), have a membrane-bound nucleus. Other algae (excluding blue-green algae), fungi, slime moulds, protozoa, higher plants, and animals are eukaryotic. Three fundamental characteristics are often considered to distinguish prokaryotes from eukaryotes: small size, absence of a complex, organelle containing cytoplasm, and the absence of a nuclear membrane. Differences b/w Prokaryotic and Eukaryotic cells Prokaryotic cell Eukaryotic cell Bacteria morphology Microorganisms are generally regarded as living forms that are microscopic in size and relatively simple, usually unicellular, in structure. The bacteria are single celled organisms that reproduce by simple division, i.e. binary fission. Most are free living and contain the genetic information, energy-producing and biosynthetic systems necessary for growth and reproduction. Microorganisms are a heterogeneous group of several distinct classes. Whittaker’s system recognizes five-kingdoms of living things—Monera (bacteria), Protista, Fungi, Plantae, and Animalia.The Five kingdoms have been modified further by the development of three domains, or Super kingdoems system— the Bacteria, the Archaea (meaning ancient), and the Eucarya. Size of Bacteria Bacteria are very small in size. The unit of measurement in bacteriology is the micron or micrometer (µm). Medical importance bacteria are generally 0.2-1.5µm in diameter and about 3- 5 µm in length. To see bacteria, a light microscope must be used. The best light microscope, using the most advanced optics, are capable of magnifications of 1000 to 2000 times. The electron microscope provides superior resolving power. Many types of microscopes are used for examination of bacteria. Shape of Bacteria Depending on their shape, bacteria are classified into several varieties 1. Cocci: Cocci (from kokko meaning berry) are spherical, or nearly spherical. Ex: streptococcus pyrogenes, staphylococcus aurus. 2. Bacilli: Bacilli (from baculus meaning rod) are relatively straight, rod shaped (cylindrical) cells. In some of the bacilli, the length of the cells may be equal to width. Such bacillary forms are known coccobacilli and have to be carefully differentiated from cocci. Example of bacilli group: Bacillus anthracis, Escherichia coli, Clostridium botulinum. 3. Vibrio: Vibrios are curved or comma-shaped rods and derive the name from their characteristic vibratory motility.Ex: Vibrio cholera, vibrio vulnificus 4. Spirilla: Spirilla are rigid spiral or helical forms.Ex: Helicobacter Pylori causes stomach ulcer, and Campylobacter jejuni. 5. Spirochetes: Spirochetes (from speira meaning coil and chaite meaning hair) are flexuous spiral forms, they move in twisted and flexible manner Ex: Treponema pallidium causative agent of Syphilis 6. Mycoplasma: Mycoplasma are cell wall deficient bacteria and hence do not possess a stable morphology. They occur as round or oval bodies and interlacing filaments.Ex: Mycoplasma hominis, Mycoplasma genitalium Anatomy of bacteria cell Bacterial Cell Components can be divided into: A. The outer layer or cell envelope consists of two components: 1. Cell wall, 2. Cytoplasmic or plasma membrane beneath cell wall. B. Cellular appendages—beside these essential components, some bacteria may possess additional structures such as capsule, fimbriae, and flagella. Capsule: Some bacteria produce a protective gelatinous covering layer called a capsule outside the cell wall. If the capsule is too thin to be seen with light microsope it is called micro capsule. Loose slime: Soluble, large-molecular, amorphous, viscid colloidal material may be dispersed by the bacterium into the environment as loose slime. Flagella: Some bacteria carry external filamentous appendages protruding from the cell wall flagella, which are organs of locomotion. Fimbriae, which appear to be organs of adhesion; and pili, which are involved in the transfer of genetic material. Bacteria Cell Interior. Those structures and substances that are bounded by the cytoplasmic membrane, compose the cell interior and include cytoplasm, cytoplasmic inclusions (mesosomes, ribosomes, inclusion granules, vacuoles) and a single circular chromosome of deoxyribonucleic acid (DNA). Cell envelope (The Outer Layer or Cell) Cell Wall The cell wall is the layer that lies just outside the plasma membrane. It is 10-25 nm thick, strong and relatively rigid, though with some elasticity, and openly porous, being freely permeable to solute molecules smaller than 10 kDa in mass and 1 nm in diameter. Functions of the cell wall: To impart shape and rigidity to the cell. It supports the weak cytoplasmic membrane against the high internal osmotic pressure of the protoplasm. Maintains the characteristic shape of the bacterium. It takes part in cell division. Also functions in interactions (e.g. adhesion) with other bacteria and with mammalian cells . Provide specific protein and carbohydrate receptors for the attachment of some bacterial viruses. Chemical Structure of Bacterial Cell Wall. Chemically the cell wall is composed of mucopeptide (peptidoglycan or murein) scaffolding formed by N -acetyl glucosamine and N- acetyl muramic acid molecules alternating in chains, which are cross-linked by peptide bonds.Peptidoglycan consists of three parts 1. A backbone—composed of alternating N-acetyl glucosamine and N-acetylmuramic acid. 2. A set of identical tetra peptide side chains attached to N-acetylmuramic acid. 3. A set of identical pentapeptide cross-bridges. In all bacterial species, the backbone is the same, however, tetra peptide side chains and pentapeptide cross-bridges vary from species to species several antibiotics interfere with construction of the cell wall peptidoglycan. In gram-positive bacteria, the cell wall consists mainly of peptidoglycan and teichoic acids, whereas in gram-negative bacteria, the cell wall is more complex in both the anatomical and chemical sense and includes the thinner peptidoglycan and outer membrane. Staining Staining is to color a microorganism with a dye, it allows for morphologic examination of organisms under a microscope. Two types of staining techniques are available: simple staining and the more advanced differential staining. Many types of each technique exist, but the differential Gram's stain is the most common. Gram's stain is used to differentiate similar organisms by categorizing them as gram-positive or gram-negative. This separation assists in determining subsequent measures to be taken for eventual identification of the organism. In Gram staining, the gram positive organisms take up the crystal violet dye whereas the gram negative are stained with the counter stain safarani red. The specimen is viewed under a microscope and a series of steps are performed. A red or pink specimen at completion indicates a gram-negative organism, whereas a purple or blue specimen indicates a gram-positive organism. Difference between Cell Wall of Gram-positive and Gram-negative Bacteria The difference between gram-positive and gram-negative bacteria has been shown to reside in the cell wall. Gram positive cell wall in general, the walls of the gram-positive bacteria have simpler chemical nature than those of gram-negative bacteria. The integrity of the cell wall is essential to the viability of the bacterium. The protoplasm may swell from osmotic inflow of water and burst the weak cytoplasmic membrane if the wall is weakened or ruptured. This process of lethal disintegration and dissolution is termed lysis. The gram-positive bacterial cell wall is about 80 nm thick and is composed mostly of several layers of peptidoglycan in addition Teichoic acid is also found in the wall. Peptidoglycan make up 50-90 percent of the dry weight of the wall and are thicker and stronger (more extensively cross-linked) than those of gram-negative bacteria. Protein is also imbedded in the cell wall. Gram-negative Cell Wall The gram-negative cell wall is structurally quite different from that of gram-positive cells it consists of peptidoglycan and, lipoprotein outer membrane, and lipopolysaccharide. I. Peptidoglycan layer: Peptidoglycan layer is a single-unit thick and constitutes 5-10 percent of the dry weight of the wall of gram-negative bacteria. It is bonded to lipoproteins covalently in the outer membrane and plasma membrane and is in the periplasmic, a gel like fluid between the outer membrane and plasma membrane. The periplasmic contains a high concentration of degradative enzymes and transport proteins. The periplasmic space is approximately 20 to 40 percent of the cell volume, which is far from insignificant. II. Lipoprotein: Lipoprotein, or murein lipoproteins seemingly attach (both covalently and non-covalently to the peptidoglycan by their protein portion, and to the outer membrane by their lipid component. Function: To stabilize the outer membrane and anchor it to the peptidoglycan layer. III. Outer membrane: External to the peptidoglycan, and attached to it by lipoproteins is the outer membrane. It is a bilayer structure. Its inner leaflet is composed of phospholipid while phospholipids of the outer leaflet are replaced by lipopolysaccharide (LPS) molecules. Functions: a. A protective barrier: A most important function is to serve as a protective barrier. It prevents or slows the entry of the salts, antibiotics and other toxic substances that might kill or injure the bacterium. b. Porins or Trans membrane proteins: In addition to LPS, the outer membrane also contains several important proteins that function in the selective transport of the nutrients into the cell. Porins or trans membrane proteins, traverse the outer membrane and form trimetric channels that permit the passage of molecules such as nucleotides, disaccharides, peptides, amino acids, vitamin B12, and iron. IV. Lipopolysaccharide (LPS): a structural component that is unique to the gram- negative outer membrane is lipopolysaccharide (LPS). It is a large complex molecule that contains lipids and carbohydrates and consists of three components: a. Lipid A is the lipid portion of LPS and is embedded in the top layer of the outer membrane. When gram-negative bacteria die, they release Lipid A, which functions as an endotoxin. All the toxicity of the endotoxin is due to lipid A which is responsible for the endotoxic activities, that is, pyrogenicity, lethal effect, tissue necrosis, ant complementary activity, B-cell mitogenicity, immunoadjuvant property and anti- tumor activity. b. The core polysaccharide is attached to lipid A and a terminal series of repeat unit contains unusual sugars. Its role is to provide stability. O polysaccharide: Extends outward from the core polysaccharide and is composed of sugar molecules. O polysaccharides function as antigens and are useful for distinguishing species of gram-negative bacteria. The role is comparable to that of teichoic acids in gram-positive cells. Polysaccharide represents a major surface antigen of the bacterial cell. It is known as O antigen. Bacteria carrying LPS containing O antigen form smooth colonies in bacteriological media in contrast to those lacking the O antigen, which form rough colonies. Outer membrane of the gram-negative cell wall prevents excess of lysozyme unless disrupted by an agent such as ethylenediaminetetraacetic acid (EDTA), a chelating agent. ii. Autolysins: Bacteria themselves possess enzymes, called autolysins, able to hydrolyze their own cell wall substances. These enzymes presumably play essential functions in cell growth and division, but their activity is most apparent during the dissolution of dead cells (autolysis). Cytoplasmic (Plasma) Membrane Structure: The cytoplasmic (plasma) membrane limits the bacterial protoplast. It is thin (5-10 nm thick), elastic and can only be seen with electron microscope. It is a typical “unit membrane”, composed of phospholipids and proteins. Lipid molecules are arrayed in a double layer with their hydrophilic polar regions externally aligned and in contact with a layer of protein at each surface. Chemically, the membrane consists of lipoprotein with small amounts of carbohydrate. With the exception of Mycoplasma, bacterial cytoplasmic membrane lacks sterols. Functions of Cytoplasmic Membrane in bacteria i. Semipermeable membrane—controlling the inflow and outflow of metabolites to and from the protoplasm. ii. Housing enzymes—involved in outer membrane synthesis, cell wall synthesis, and in the assembly and secretion of extra cytoplasmic and extracellular substances. iii. Housing many sensory and chemo taxis proteins that monitor chemical and physical changes in the environment. iv. Generation of chemical energy (i.e., ATP). v. Cell motility. vi. Mediation of chromosomal segregation during replication Functions of Capsule i. Virulence factor: Capsules often act as a virulence factor by protecting the bacterium from ingestion by phagocytosis, and non-capsulate mutant of bacteria caused by repeated subcultures in vitro lead to the loss of capsule and also of virulence. ii. Protection of the cell wall: In protecting the cell wall attack by various kinds of antibacterial agents, e.g. bacteriophages, colicines, complement, lysozyme and other lytic enzymes. iii. Identification and typing of bacteria: Capsular antigen is specific for bacteria and can be used for identification and typing of bacteria. Flagella: Motile bacteria except spirochetes, possess one or more unbranched, long filaments called flagella, which are the organs of locomotion. Structure: They are long, hollow, helical filaments, usually several times the length of the cell. They are 3-20 µm long and are of uniform diameter (0.01-0.013 µm) and terminate in a square tip. It originates in the bacterial protoplasm and is extruded through the cell wall. Flagella consists of largely or entirely of a protein, flagellin, belonging to the same chemical group as myosin, the contractile protein of muscle. Though flagella of different genera of bacteria have the same chemical composition, they are antigenically different. Flagella are highly antigenic and flagellar antigens induce specific antibodies in high titers. Several of the immune responses mounted by host systems are directed against these flagellar antigen. Flagellar antibodies are not protective but are useful in serodiagnosis. Flagella can be found on both gram-positive and gram- negative bacilli. Most cocci are immotile, whereas about half of the bacilli and almost all of the spirilla possess flagella. Each flagellum consists of three parts (. i. Filament ii. Hook iii. Basal body). i. Filament: The filament is the longest and most obvious portion which extends from the cell surface to the tip. ii. Hook: The hook is a short, curved segment iii. Basal body: The basal body is embedded in the cell (cytoplasmic membrane). In the gram-negative bacteria, the basal body has four rings connected to a central rod (L, P, S and M). The outer L and P rings associated with the lipopolysaccharide and peptidoglycan layers respectively. S ring is located just above the cytoplasmic membrane and the inner M ring contacts the cytoplasmic membrane. Gram-positive bacteria have only two basal body rings, an inner ring connected to the cytoplasmic membrane and an outer one probably attached to peptidoglycan. Cell interior/ Cytoplasm, The cytoplasm of the bacterial cell is a viscous watery solution or soft gel, containing a variety of organic or inorganic solutes, and numerous ribosomes and polysomes. The cytoplasm of bacteria differs from that of the higher eukaryotic organisms in not containing an endoplasmic reticulum or membrane-bearing microsomes, mitochondria, lysomes and in showing signs of internal mobility, e.g. cytoplasmic streaming, the formation, migration and disappearance of vacuoles, and amoeboid movement. The cytoplasm may contain granules or inclusions such as starch, glycogen, poly-b-hydroxy/alkanoates, sulphur globules, magnetosomes, parasporal bodies, gas vesicles and endospores. The cytoplasm stains uniformly with basic dyes in young cultures. Intracytoplasmic Inclusions.The synthesis and accumulation of intracellular inclusions in different prokaryotes is usually dependent upon both nutritional availability and environmental growth conditions. These are not permanent or essential structures, and may be absent under certain conditions of growth. These bodies are usually for storage, and reduce osmotic pressure by tying up molecules in particulate form. They consist of volutin (polyphosphate), lipid, glycogen, starch or sulfur. Functions of Mesosomes i. Compartmenting of DNA: These are thought to be involved in mechanisms responsible for compartmenting of DNA at cell division and sporulation. ii. Sites of the respiratory enzymes: They provide increased membrane surface and are the principle site for storing respiratory enzymes. Bacterial Nucleus Structure: The genetic material of a bacterial cell is contained in a single, long molecule of double-stranded deoxyribonucleic acid (DNA) which can be extracted in the form of a closed circular thread about 1 mm (1000 µm) long, about 1000 times the length of the cell. Therefore, it occurs tightly coiled like a skein of woolen thread. The nuclear deoxyribonucleic acid (DNA) is not associated with basic protein. The bacterial chromosome is haploid and replicates by simple fission instead of by mitosis as in higher cells. Bacterial nucleus does not possess nuclear membrane (separating them from the cytoplasm), nucleolus, and deoxyribonucleoprotein. The differences between the nuclei of bacteria and higher organisms form the main basis for classifying them as prokaryotes and eukaryotes. Plasmids. Bacteria may possess extra nuclear genetic elements in the cytoplasm consisting of DNA, termed plasmids or episomes. These can exist and replicate independently of the chromosome or may be integrated with it. In either case they normally are inherited or passed on to the progeny either through conjugation or the agency of bacteriophages. Function of plasmids: Plasmids are not essential for host growth and reproduction they inhabit, but may confer on it certain properties such as drug resistance, bacitracin production, resistance to toxic metal. Bacterial Spore. A number of gram-positive bacteria, such as those of the genera Clostridium and Bacillus can form a special resistant dormant structure called an endospore or simply spores. Endospore develop when essential nutrients are depleted. In sporulation, each vegetative cell forms only one spore, and in subsequent germination, each spore gives rise to a single vegetative cell. Sporulation in bacteria therefore is not a method of reproduction but of preservation. Sporulation: Spore formation, sporogenesis normally commences when growth ceases due to lack of nutrients, depletion of the nitrogen or carbon source (or both) being the most significant factor. New antigens appear on sporulation that are not found in the vegetative cell.Stages: It is a complex process and may be divided into several stages. Germination is the process of conversion of a spore into vegetative cells under suitable conditions. It occurs in three stages: activation, initiation and outgrowth. Properties of Endospores 1. Core: The fully developed spore has the core which is the spore protoplast containing the normal cell structures but is metabolically inactive. 2. Spore wall: The innermost layer surrounding the inner spore membrane is called the spore wall. It contains normal peptidoglycan and becomes the cell wall of the germinating vegetative cell. 3. Cortex: The cortex is the thickest layer of the spore envelope. Cortex peptidoglycan is extremely sensitive to lysozyme, and its autolysins plays a role in spore germination. 4. Spore coat: Cortex, in turn, is enclosed by fairly thick spore coat. 5. Exosporium: Spores of some species have an additional, apparently rather loose covering known as the exosporium, which may have distinctive ridges and grooves. Endospore structure Resistance Bacterial spores. These constitute some of the most resistant forms of life. They may remain viable for centuries. Though some spores may resist boiling for prolonged periods, spores of all medically important species are destroyed by autoclaving at 120°C for 15 minutes. Methods of sterilization and disinfection should ensure that spores also are destroyed. Sporulation helps bacteria survive for long periods under unfavorable environments. Endospore heat resistance probably is due to several factors: calcium-dipicolinate and acid-soluble protein stabilization of DNA, protoplast dehydration, the spore coat, DNA repair, the greater stability of the cell proteins in bacteria adapted to growth at high temperatures and others. Pleomorphism and involution form during growth. Bacteria of a single strain may show considerable variation in size and shape, or form a proportion of cells that are swollen, spherical, elongated or pear shaped. This is known as Pleomorphism and occurs most readily in certain species (e.g. Streptobacillus and Yersinia pestis) in aging cultures on artificial medium and especially in the presence of antagonistic substances such as penicillin, glycine, lithium chloride, sodium chloride in high concentration, and organic acids at low pH. The abnormal cells are generally regarded as degenerate or involution forms. Many of cells may be non-viable, whereas others may grow and revert to normal form when transferred to a suitable environment. Pleomorphism and involution forms are often due to defective cell wall synthesis. Involution forms may also develop due to the activity of autolytic enzymes. Bacteria growth and Nutrition Growth may be defined as the orderly increase of all of the chemical constituents of the cell. Bacterial growth involves both an increase in the size of individuals and increase in the number of individuals. Whatever the balance between these two processes, the net effect is an increase in the total mass (biomass). Bacterial Division Bacteria divide by binary fission where individual cells enlarge and divide to yield two progeny of approximately equal size. Nuclear division precedes cell division and, therefore, in a growing population, many cells carrying two nuclear bodies can be seen. The cell division occurs by a constrictive or pinching process, or by the ingrowth of a transverse septum across the cell. The daughter cells may remain partially attached after division in some species. Binary fission Generation Time or Doubling Time The size of a population of bacteria in a favorable growth medium increases exponentially. The interval of time between two cell divisions, or the time required for a bacterium to give rise to two daughter cells under optimum conditions, is known as the generation time or doubling time. Examples: In coliform bacilli and many other medically important bacteria, it about 20 minutes, in tubercle bacilli it is about 20 hours and in leprae bacilli it is about 20 days. It is often difficult to grasp fully the scale of exponential microbial growth. As bacteria reproduce so rapidly and by geometric progression, a single growth cannot be sustained indefinitely in a closed system (batch culture) with limited available nutrients. Colonies: A clone of cells derived from a single parent cell is known as colony. Bacteria growing on solid media form colonies. It is likely that all phases of growth are represented in colonies, bacterial cell can theoretically give rise to 1021 progeny in 24 hours, with a mass of approximately 4,000 tonnes. Phases of bacteria growth Lag phase When microorganisms are introduced into fresh culture medium, usually no immediate increase in cell number occurs, and therefore this period is called the lag phase. After inoculation, there is an increase in cell size at a time when little or no cell division is occurring. During this time, however, the cells are not dormant. This initial period is the time required for adaptation to the new environment, during which the necessary enzymes and metabolic intermediates are built up in adequate quantities for multiplication to proceed. The lag phase varies considerably in length with the species, nature of the medium, size of inoculum and environmental factors such as temperature and nutrients present in the new medium. Log (logarithmic) or exponential phase Following the lag phase, the cells start dividing and their numbers increase exponentially or by geometric progression with time. If the logarithm of the viable count is plotted against time, a straight line will be obtained. The population is most uniform in terms of chemical and physiological properties during this phase. Therefore, exponential phase cultures are usually used in biochemical and physiological studies. Exponential phase is of limited duration because of exhaustion of nutrients; accumulation of toxic metabolic end products; rise in cell density, change in pH; and decrease in oxygen tension (in case of aerobic organisms). The log phase is the time when cells are most active metabolically and is preferred for industrial purposes. However, during their log phase of growth, microorganisms are particularly sensitive to adverse conditions. Radiation and many antimicrobial drugs, e.g. the antibiotic penicillin—exert their effect by interfering with some important step in the growth process and are therefore, most harmful to cells during this phase. Stationary phase. After a varying period of exponential growth, cell division stops due to depletion of nutrients and accumulation of toxic products. Eventually growth slows down, and the total bacterial cell number reaches a maximum and stabilizes. The number of progeny cells formed is just enough to replace the number of cells that die. The growth curve becomes horizontal. The viable count remains stationary as an equilibrium exists between the dying cells and the newly formed cells. Decline or death phase. The death phase is the period when the population decreases due to cell death. Eventually the rate of death exceeds the rate of reproduction, and the number of viable cells declines. Like bacterial growth, death is exponential cell death may also be caused by autolysis besides nutrient deprivation and buildup of toxic wastes. Finally, after a variable period, all the cells die and culture becomes sterile. The total count shows a phase of decline with autolytic enzymes activity. Association of Growth Curve and Cell Changes The various stages of the growth curve are associated with morphological and physiological alterations of the cells. It has been possible to define the effect of growth rate on the size and specialized growth techniques. 1. Lag phase: Bacteria have the maximum cell size towards the end of the lag phase. 2. Log phase: Cells are smaller and stain uniformly in the log phase. 3. Stationary phase: In the stationary phase, cells frequently are Gram variable and show irregular staining due to the presence of intracellular storage granules. Sporulation occurs at this stage and also many bacteria produce secondary metabolic products such as exotoxins and antibiotics. 4. Decline phase: In the phase of decline, involution forms are common. Bacteria growth curve Detection and Measurement of Bacterial Growth A. Direct cell counts 1. Direct microscopic count: In this method known as the direct microscopic count, a measured volume of a bacterial suspension is placed within a defined area on a microscope slide. Breed count method is used to count the number of bacteria in milk. A specially designed slide called a Petroff-Hausser cell counter is also used in direct microscopic counts. 2. Cell-counting instruments: Coulter counters and flow cytometers count total cells in dilute solutions. B. Viable cell counts the viable count measures the number of living cells, that is, cells capable of multiplication. C. Measuring biomass: 1. Turbidity; 2. Dry weight; 3. Chemical Constituents. D. Measuring cell products 1. Acid; 2. Gases; 3. ATP. Bacteria culture and culture media Culture medium: A nutrient material prepared for the growth of microorganisms in a laboratory is called a culture medium. Common ingredients of culture media: Some of the components of culture media are as follows: 1. Water: Tap water is often suitable for culture media, particularly if it has a low mineral content, but if the local supply is found unsuitable, glass-distilled or demineralized water must be used instead. 2. Agar: Agar (or agar-agar) is prepared from a variety of seaweeds and is now universally used for preparing solid media. The chief component of agar is a long-chain polysaccharide. It also contains a variety of impurities including inorganic salts, a small amount of protein like material and sometimes traces of long-chain fatty acids which are inhibitory to growth. The minerals present are mainly magnesium and calcium. Agar does not add to the nutritive properties of a medium and is not affected by the growth of bacteria. The melting and solidifying points of agar solutions are not the same. At the concentrations normally used, most bacteriological agars melt at about 95°C and solidify only when cooled to about 42°C. There are considerable differences in the properties of the agars manufactured in different places, and even between different batches from the same source. A concentration of 1-2 percent usually yields a suitable gel, but the manufacturer’s instructions should be followed. The jellifying property varies in different brands of agar; for example, New Zealand agar has more jellifying capacity than Japanese agar. Agar is manufactured either in long shreds or as powder. There may be variations between different batches of the same brand, 3. Peptone: Another almost universal ingredient of common media is peptone. It is a complex mixture of partially digested proteins. The important constituents are peptones, proteoses, amino acids, a variety of inorganic salts including phosphates, potassium and magnesium, and certain accessory growth factors such as nicotinic acid and riboflavin. The brands of peptone supplied by different manufacturers show appreciable differences in composition and growth-promoting properties. Moreover, variations may occur between different batches of one brand. Apart from the standard grades of bacteriological peptone, some manufacturers supply special grades of peptone recommended for particular purposes, e.g. Neopeptone, proteose peptone, mycological peptone, etc. Commercially available peptones or digest broth can be used. Meat extract is also available commercially and is known as LabLemco. 4. Yeast extract: It contains a wide range of amino acids, growth factors and inorganic salts. Yeast extract is used mainly as a comprehensive source of growth factors and may be substituted for meat extract in culture media. 5 percent of proteins and protein breakdown products, and a wide range of mineral salts and growth factors. 6. Blood and serum: These are used for enriching culture media. Either human or animal blood can be used. Usually 5-10 percent blood is used and the most usual concentration is 10 percent. Serum is used in certain media. Types and Phases of Growth Media Growth media are used in either of two phases: liquid (broth) or solid (agar). In some instances (e.g. certain blood culture methods), a biphasic medium that contains both a liquid and a solid phase is used. (Semisolid media) a. Liquid (broth) media: In broth media nutrients are dissolved in water, and bacterial growth is indicated by a change in broth’s appearance from clear to turbid, (i.e. cloudy). Numerous culture media have been devised. The earliest culture media were liquid, which made the isolation of bacteria to prepare pure cultures extremely difficult. The original media used by Louis Pasteur were liquids such as urine or meat broth. Disadvantages i. Growths usually do not exhibit special characteristic appearances in them and they are of only limited use in identifying species. ii. Also, organisms cannot be separated with certainty from mixtures by growth in liquid media. If solid media are used, these disadvantages are overcome. Uses i. For obtaining bacterial growth from blood or water when large volumes have to be tested ii. For preparing bulk cultures of antigens or vaccines. Solid (agar) media: Solid media are made by adding a solidifying agent to the nutrients and water. Agarose is the most common solidifying agent. Types of media Based on nutrition Simple media (Basal media) Simple media are those medias that contain only basic nutrients required for the growth of ordinary organisms, and used as a general purpose media, e.g. peptone water, nutrient broth and nutrient agar. These simple media are generally used as the basis to prepare enriched media; hence known as basal media. Complex media Media that contain some ingredients of unknown chemical composition are called complex media.A complex medium contains a variety of ingredients such as meat juices and digested proteins. The exact chemical composition of these ingredients can be highly variable although a specific amount of each ingredient is in the medium. One common ingredient is peptone. This is protein taken from any of a variety of sources that has been hydrolyzed to amino acids and short peptides by treatment with enzymes, acids, or alkali. Extracts, which are the water-soluble components of a substance. Classification of culture media A. Based on phases of growth C. Special media media (i) Enriched media 1. Liquid (broth) media (ii) Enrichment media (iii) Selective media 2. Solid (agar) media (iv) Indicator or differential media 3. Semisolid media (v) Transport media B. Based on nutritional factors (vi) Sugar media 1. Simple media (Basal media) D. Reducing media 2. Complex media e.g.anaerobic blood agar 3. Synthetic or defined Colonies: are clones of cells originating from a single bacterial cell. While bacteria grow diffusely in liquids, they produce discrete visible growth on solid media. If inoculated in suitable dilutions, bacteria form colonies, Bacterial cultures derived from a single colony or clone are considered “pure”. On solid media, bacteria have distinct colony morphology and exhibit many other characteristic features such as pigmentation or hemolysis, making identification easy. Inoculum: When microbes are introduced into a culture medium to initiate growth, they are called an inoculum. Culture: The microbes that grow and multiply in or on a culture medium are referred to as a culture. Cultivation is the process of growing microorganisms in culture by taking bacteria from the infection site (i.e. in vivo environment) by some means of specimen collection and growing them in the artificial environment of the laboratory (i.e. the in vitro environment). By appropriate procedures they have to be grown separately (isolated) on culture media and obtained as pure cultures for study. Once grown in culture, most bacterial populations are easily observed without microscopy and are present in sufficient quantities to allow laboratory testing to be performed Types of bacteria culture Batch Culture or Closed System In the laboratory, bacteria are typically grown in broth Contained in a tube or flask, or on an agar plate with limited amount of nutrients. These are considered batch or closed systems.to maintain the growth and yield and survival of cells the organisms must be transferred to a continuous culture mode. To maintain cells in a state of continuous growth, nutrients must be continuously added and waste products removed. This is called continuous culture or open system. Continuous culture is using a chemo stat in which cells of a growing culture are continuously harvested and nutrients continuously replenished. The second type of continuous culture system, the turbid stat, has a photocell that measures the absorbance or turbidity of the culture in the growth vessel. The flow rate of media through the vessel is automatically regulated to maintain a predetermined turbidity or cell density Main purpose of bacteria cultivation. Bacterial cultivation has three main purposes: 1. To grow and isolate all bacteria present in an infection. 2. Infection and contaminants or colonizers: To determine which of the bacteria that grow are most likely causing infection and which are likely contaminants or colonizers. 3. Identification and characterization: To obtain sufficient growth of clinically relevant bac- teria to allow identification and characterization. Bacteria nutrition. The minimum nutritional requirements for growth and multiplication of bacteria are water, a source of carbon, a source of nitrogen and some inorganic salts. The water content of bacterial cells can vary from 75 to 90 percent of the total weight and is the vehicle for the entry of all cells and for the elimination of all waste products. It participates in the metabolic reactions and also forms an integral part of the protoplasm Categories of Requirements for Microbial Growth. The requirements for microbial growth can be divided into two main categories: (i) chemical and (ii) physical. 1. Chemical Requirements Chemical requirements include sources of carbon, nitrogen, sulfur, phosphorus, trace elements, and organic growth factors. 1. Carbon Source o All bacteria require a carbon source to produce energy and build cellular structures: Heterotrophs: Obtain carbon from organic compounds like sugars, proteins, or lipids. Autotrophs: Use inorganic carbon (like carbon dioxide) to produce organic compounds via processes like photosynthesis or chemosynthesis. 2. Nitrogen Source o Nitrogen is essential for synthesizing amino acids, proteins, and nucleic acids. Bacteria can obtain nitrogen from: Ammonia (NH₃), nitrates (NO₃⁻), or nitrogen gas (N₂). Some bacteria can fix atmospheric nitrogen into usable forms (like ammonium). 3. Sulfur o Sulfur is necessary for the synthesis of certain amino acids (like cysteine and methionine) and vitamins. It is obtained from compounds like sulfates, hydrogen sulfide, or sulfur-containing organic molecules. 4. Phosphorus o Phosphorus is important for the synthesis of nucleic acids (DNA, RNA), ATP, and phospholipids in cell membranes. It is typically obtained from inorganic phosphates (PO₄³⁻). 5. Trace Elements (Minerals) o Bacteria need certain trace elements for enzyme activity and metabolic processes, including: Iron: Essential for electron transport and metabolism. Magnesium: Required for stabilizing ribosomes and enzyme function. Calcium: Important for cell wall stability and some enzyme activities. Zinc, manganese, and copper: Serve as cofactors for various enzymatic reactions. 6. Organic growth factors for bacteria are essential compounds that bacteria cannot synthesize on their own and must obtain from their environment in order to grow and reproduce. These factors include: 1. Vitamins: Many bacteria require vitamins like B vitamins (e.g., thiamine, riboflavin, and biotin) to carry out metabolic processes. 2. Amino acids: Some bacteria cannot synthesize certain amino acids and must take them up from their surroundings to build proteins. 3. Purines and pyrimidines: These are the building blocks for nucleic acids (DNA and RNA). Bacteria that cannot synthesize these compounds will require them from external sources. 4. Fatty acids: Essential for membrane synthesis and other cellular functions, fatty acids might need to be provided externally for some bacteria. 5. Coenzymes: Many bacterial enzymes require coenzymes (such as coenzyme A, NAD+, or FAD) to function properly in metabolic pathways. 6. Peptides: Short chains of amino acids, such as small peptides, may also be needed by certain bacteria for growth. Microbiological Assays Ideally the amount of growth resulting is directly proportional to the quantity of growth factor present. This is the principle of microbiological assays which are specific, sensitive, and simple. They still are used in the assay of substances like vitamin B12 and biotin. Physical Requirements/ Physical Factors Influencing Microbial Growth 1. Temperature Bacteria have specific temperature ranges within which they grow which is known as Optimum temperature.Each bacterial species has an optimal temperature for growth and a temperature range above and below will blocked the growth. Bacteria grow best at optimum temperature Thus, bacteria pathogenic for humans usually grow at 37ºC (our body temperature). Bacteria are divided into three groups on the basis of temperature ranges through which they grow: a. Psychrophiles: Thrive in cold temperatures, typically below 15°C. b. Mesophiles: Grow best at moderate temperatures, around 20°C to 45°C (many human pathogens fall into this group). c. Thermophiles: Prefer high temperatures, ranging from 45°C to 80°C. Hyperthermophiles: Grow optimally at temperatures above 80°C, often found in extreme environments like hot springs or deep-sea vents. 2. pH.Bacteria have specific pH ranges they can tolerate: Neutrophiles: Prefer neutral pH (around 7). Acidophiles: Thrive in acidic environments (pH below 7). Alkalophiles: Prefer alkaline conditions (pH above 7). 3. Oxygen.Oxygen availability is a major factor influencing bacterial growth. Based on their O2 requirements, prokaryotes can be separated into aerobes and anaerobes. Aerobic bacteria require oxygen for growth and may be: i. Obligate aerobes: They have an absolute or obligate requirement for oxygen (O2), like the cholera vibrio. Anaerobic bacteria do not require oxygen for their growth e.g. Clostridium tetani, Bactericides fragilis. Many groups exist of each: Obligate aerobes: Require oxygen for growth.in the absence of oxygen bacteria growth stops.e.g. Mycobacterium tuberculosis, Pseudomonas aeruginosa, Neisseria species Obligate anaerobes: Cannot tolerate oxygen and grow only in the absence of it. e.g. Clostridium species (e.g., Clostridium botulinum, Clostridium tetani), Bacteroides species (e.g., Bacteroides fragilis) Facultative anaerobes: Can grow with or without oxygen, but generally grow better in its presence. E.g. Staphylococcus spp.; Escherichia coli, etc. Most bacteria of medical importance are facultative anaerobes. Microaerophiles: Require low levels of oxygen (less than atmospheric concentration). They grow best at low oxygen tension (~5%) e.g. Campylobacter spp., Helicobacter spp. Aerotolerant: Do not require oxygen but can tolerate its presence.e.g. (e.g., Enterococcus faecalis,lactobacillus) 4. Moisture.Water is essential for bacterial metabolism and growth. Bacteria require a moist environment to maintain cellular functions, including enzymatic activity, nutrient transport, and waste removal. 5. Pressure. Some bacteria, especially those found in deep-sea environments, require high hydrostatic pressures for growth. These are known as barophiles or piezophiles. Other bacteria may require standard atmospheric pressure for optimal growth. 6. Carbon Dioxide All bacteria require small amount of carbon dioxide for growth. Thus, this requirement is usually met by the carbon dioxide present in the atmosphere, or produced endogenously by cellular metabolism. Some organisms such as Brucella abortus, require much higher levels of carbon dioxide (5-10%) for growth, especially on fresh isolation (capnophilic). Pneumococci and gonococci are other capnophilic which grow better in air supplemented with 5 to 10 percent CO2. 7. Light Darkness provides a favorable condition for growth and viability of bacteria. Bacteria are sensitive to ultraviolet light and other radiations as ultraviolet rays from direct sunlight or a mercury lamp are bactericidal. Bacteria are also killed by ionizing radiations. Exposure to light may influence pigment production. Photo chromogenic mycobacteria form pigment only on exposure to light. 8. Osmotic Effect Tolerance to osmotic variation: Bacteria are more tolerant to osmotic variation because of the mechanical strength of the cell wall. Except for the mycoplasma and other cell wall defective organisms, the majority of the bacteria are osmotically tolerant. Plasmolysis: When microorganisms with rigid cell walls are placed in a hypertonic environment, water leaves and the plasma membrane shrinks away from the wall, a process known as plasmolysis. This occurs more readily in gram-negative bacteria than in gram-positive bacteria. Plasmoptysis: Sudden transfer of bacteria from concentrated solution to distilled water may also cause plasmoptysis due to excessive osmotic imbibition of water leading to swelling and rupture of the cell. 9. Mechanical and Sonic Stresses In spite of tough walls of bacteria, they may be ruptured by mechanical stress such as grinding or vigorous shaking with glass beads. Exposure to ultrasonic vibration may also disintegrate bacteria. Major elements (Macro elements or macronutrients) Elements that make up cell constituents are called major elements (macro elements or macronutrients) and over 95 percent of cell dry weight is made up of a few major elements. These include carbon, oxygen, hydrogen, nitrogen, sulfur, phosphorus, potassium, magnesium, calcium, and iron. Bacteria also require some elements in small amount called trace elements. They include cobalt, zinc, copper, molybdenum, and manganese. These elements form parts of enzymes or may be 1required for enzyme function. They aid in the catalysis of reactions and maintenance of protein structure. Very small amounts of these trace elements are found in most natural environments, including water. Energy Sources Organisms derive energy either from sunlight or metabolizing chemical compounds. Phototrophs: Organisms that gain energy from light are called phototrophs (photo means “light”). Chemotrophs: Organisms that obtain energy by metabolizing chemical compounds are called Chemotrophs (chemo means “chemical”) Bacteria metabolism. Metabolism of the substance is defined as the series of changes of substance (carbohydrate, protein or fat) within the bacterial cell from absorption to elimination. Metabolism may be aerobic or anaerobic and can be divided into two classes of chemical reactions: those that release energy and those that require energy. There are three critical processes of bacterial energy production; aerobic respiration, anaerobic respiration and fermentation. There are two general aspects of energy production: the concept of oxidation-reduction and the mechanisms of ATP generation Patterns of energy release. Oxidation-reduction (Redox) Reactions Oxidation is the removal of electrons, and reduction is the addition of electrons. In other words, each time one substance is oxidized, another is.simultaneously reduced. The pairing of these reactions is called oxidation-reduction or a redox reaction. Generation of ATP. Much of the energy released during oxidation-reduction reactions is trapped within the cell by the formation of ATP. Specifically, a phosphate group, P, is added to ADP with the input of energy to form ATP: The symbol ~ designates a “high-energy” bond— that is, one that can readily be broken to release usable energy. The high-energy bond that attaches the third P (phosphate group) in a sense contains the energy stored in this reaction. When this P (phosphate group) is removed, usable energy is released. The addition of P (phosphate group) to a chemical compound is called phosphorylation Mechanisms of phosphorylation: There are two general mechanisms for ATP production in bacterial cells. Substrate-level phosphorylation in substrate level phosphorylation, high energy phosphate bonds produced by the central pathways are donated to adenosine diphosphate (ADP) to form ATP. Additionally, pyruvate, a primary intermediate in the central pathways, serves as the initial substrate for several other pathways that also can generate ATP by substrate level phosphorylation. These other pathways constitute fermentative metabolism, which does not require oxygen and produces various end products, including alcohols, acids, carbon dioxide, and hydrogen. The specific fermentative pathways used, and hence end products produced, vary with different bacterial species. Detecting these products serves as an important basis for laboratory identification of bacteria. Fermentation is carried out by both obligate and facultative anaerobe Oxidative phosphorylation: In oxidative phosphorylation, an electron transport system is involved that conducts a series of electron transfers from reduced carrier molecules such as NADH2 and NADPH2, produced in the central pathways, to a terminal electron acceptor. The energy produced by the series of oxidation-reduction reactions is used to generate ATP from ADP. The process is known as aerobic respiration when oxidative phosphorylation uses oxygen as the terminal electron acceptor. Anaerobic respiration refers to processes that use final electron acceptors other than oxygen Glycolysis/embden Meyerhof pathway Glycolysis is the earliest discovered and most important process of carbohydrates metabolism. Glycolysis – metabolic pathway in which glucose is transformed to pyruvate with production of a small amount of energy in the form of ATP or NADH it is an anaerobic process (it does not require oxygen). Glycolysis pathway is used by anaerobic as well as aerobic organisms. The glycolytic pathway consist of ten enzyme catalyzed reactions that begin with a glucose and split it into two molecules of pyruvate in glycolysis one molecule of glucose is converted into two molecules of pyruvate. In both prokaryotic and eukaryotic cells, glycolysis takes place in the cytoplasm. Pyruvate can be further metabolized to: (1) Lactate or ethanol (anaerobic conditions) (2) Acetyl CoA (aerobic conditions). Acetyl CoA is further oxidized to CO2 and H2O via the citric acid cycle ,Much more ATP is generated from the citric acid cycle than from glycolysis Glycolysis pathway Pentose phosphate pathway The Role of Pentose Phosphate Pathway (phosphogluconate pathway) 1. Synthesis of NADPH (for reductive reactions in biosynthesis of fatty acids and steroids) 2. Synthesis of Ribose 5-phosphate (for the biosynthesis of ribonucleotides (RNA, DNA) and several cofactors) 3. Pentose phosphate pathway also provides a means for the metabolism of “unusual sugars” , 4, 5 and 7 carbons. The Role of Pentose Phosphate Pathway (phosphogluconate pathway) Pentose phosphate pathway does not function in the production of high energy compounds like ATP. Pentose Phosphate pathway TCA cycle/tricarbosylic acid cycle Tricarboxylic acid (TCA) cycle, electron transport and oxidative phosphorylation Pyruvate produced from glycolysis and other metabolic pathways is metabolized in various ways depending on the organism and growth conditions. Pyruvate is either used as a precursor for biosynthesis, or is oxidized completely to CO2 under aerobic conditions. This chapter is devoted to the mechanisms of pyruvate oxidation, electron transport and oxidative phosphorylation. 5.1 Oxidative decarboxylation of pyruvate Pyruvate is oxidized by the pyruvate dehydrogenase complex to acetyl-CoA and CO2 reducing NADþ under aerobic conditions. The pyruvate dehydrogenase complex consists of 24 molecules of pyruvate dehydrogenase containing thiamine pyrophosphate (TPP), 24 molecules of dihydrolipoate acetyltransferase containing dihydrolipoate and 12 molecules of dihydrolipoate dehydrogenase containing flavin adenine dinucleotide (FAD). In addition, NADþ and coenzyme A participate in the reaction. This reaction is irreversible, and takes place in the mitochondrion in eukaryotic cells. The pyruvate dehydrogenase complex shares its properties with other 2-keto acid dehydrogenase complexes that produce acyl-CoA such as 2-ketoglutarate dehydrogenase (reaction 4 and 2- Ketobutyrate dehydrogenase but their activities are controlled differently. Pyruvate dehydrogenase activity is controlled by its substrate, products and the adenylate energy charge. Pyruvate and AMP activate enzyme activity, and acetyl-CoA, NADH and ATP repress it. Pyruvate and acetyl-CoA are precursors for amino acid and fatty acid biosynthesis, respectively. Tricarboxylic acid (TCA) cycle this cyclic metabolic pathway was discovered by Krebs and his colleagues in animal tissue. It is referred to as the tricarboxylic acid (TCA) cycle, Krebs cycle or citric acid cycle. Acetyl-CoA produced by the pyruvate dehydrogenase complex is completely oxidized to CO2, reducing NADþ, NADPþ and FAD. These reduced electron carriers are oxidized through the processes of electron transport and oxidative phosphorylation to form the proton motive force and to synthesize ATP. Oxidative decarboxylation of pyruvate by the pyruvate dehydrogenase complex. Pyruvate dehydrogenase (E1) decarboxylates pyruvate and its prosthetic group thiamine pyrophosphate (TPP) binds the resulting hydroxyethyl group, which binds to the lipoate (Lip) of dihydrolipoate acetyltransferase (E2), reducing its disulfide. E2 transfers an acetyl group to coenzyme A to form acetyl- CoA. Dihydrolipoate dehydrogenase (E3) transfers electrons from reduced lipoate to NADþ. Acetyl-CoA oxidation through the tricarboxylic acid cycle. Enzymes: 1, citrate synthase; 2, aconitase; 3, isocitrate dehydrogenase; 4, 2-ketoglutarate dehydrogenase complex; 5, succinate thiokinase (succinyl- CoA synthetase); 6, succinate dehydrogenase; 7, fumarase (fumarate hydratase); 8, malate dehydrogenase. TCA cycle Fermentation is a metabolic process in which microorganisms like yeast, bacteria, or molds convert sugars (such as glucose) into simpler compounds, producing energy (ATP) in the absence of oxygen. It is an anaerobic process, end products of fermentation are: Lactic acid, Alcohol, Acetic acid and Butyric acid Types of Fermentation: Alcohol Fermentation: In this process, yeast (such as Saccharomyces cerevisiae) converts glucose into ethanol and carbon dioxide. This is the process used in the production of alcoholic beverages like beer, wine, and spirits. C6H12O6-------2C2H5OH + 2CO2 Lactic Acid Fermentation: Certain bacteria (such as Lactobacillus) and muscle cells in animals use this pathway, where glucose is converted into lactic acid. This type of fermentation occurs when oxygen is scarce, such as during intense exercise.C6H12O6____2C3H6O3 Acetic Acid Fermentation Organisms Involved: Acetic acid bacteria (e.g., Acetobacter species). Process: In acetic acid fermentation, ethanol is oxidized to acetic acid (vinegar) in the presence of oxygen. This is an aerobic process and distinct from the other two types, which are anaerobic. C2H5OEH+O__CH3COOH + H2O Summary of fermentation Metabolism of Pyruvate to Ethanol Ethanol is formed from pyruvate in yeast and several other microorganisms in anaerobic conditions. Two reactions required: The first step is the decarboxylation of pyruvate to acetaldehyde. Enzyme - pyruvate decarboxylase. Coenzyme - thiamine pyrophosphate (derivative of the vitamin thiamine B1) the second step is the reduction of acetaldehyde to ethanol. Enzyme - alcohol dehydrogenase (active site contains a zinc coenzyme_ NADH The conversion of glucose into ethanol is an example of alcoholic fermentation. The net result of alcoholic fermentation is: Glucose+2Pi + 2ADP + 2H+ 2 ethanol + 2CO2 + 2ATP + 2H2O There is no net NADH formation in the conversion of glucose into ethanol. NADH generated by the oxidation of glyceraldehyde 3-phosphate is consumed in the reduction of acetaldehyde to ethanol. Sensitivity and Resistance. When an organism has been isolated from a specimen, its sensitivity (susceptibility) to antimicrobial agents or antibiotics is tested. An infectious agent is sensitive to an antibiotic when the organism's growth is inhibited under safe dose concentrations. Conversely, an agent is resistant to an antibiotic when its growth is not inhibited by safe dose concentrations. Because of a number of factors, such as mutations, an organism's sensitivity, resistance, or both to antibiotics are constantly changing.1 Sterilization and disinfection: Sterilization [Latin sterilis, unable to produce offspring or barren] is defined as the process by which an article, surface, or medium is freed of all living microorganisms either in the vegetative or spore state. When sterilization is achieved by a chemical agent, the chemical is called sterilant. Disinfection is the killing, inhibition, or removal of microorganisms that may cause disease. From the beginning of recorded history, people have practiced disinfection and sterilization, even though the existence of microorganisms was long unsuspected. The Egyptians used fire to sterilize infectious material and disinfectants to embalm bodies, and the Greeks burned sulfur to fumigate buildings. Mosaic Law commanded the Hebrews to burn any clothing suspected of being contaminated with the leprosy bacterium. Methods of sterilization and disinfection 1. Physical agents 2. Chemical agents. 1. Physical agents a. Sunlight b. drying c. Heat d. Filtration e. Radiation f. Ultrasonic and sonic vibrations. Dry heat I. Incineration II. Red heat III. Flaming IV. Hot air sterilizer V. Microwave ovens. b. Moist heat I. Pasteurization II. Boiling III. Steam under normal pressure IV. Steam under pressure. 2. Chemical agents 1. Agents that damage the cell membranes a. Surface-active disinfectants b. Phenolic compounds c. Alcohols. 2. Agents that damage proteins a. Acids and alkalies b. Alcohols. 3. Agents that modify functional groups of proteins and nucleic acids a. Heavy metals b. Oxidizing agents c. Dyes d. Alkylating agents Applications of Sterilization and Disinfection: Important applications in practical microbiology and in the practice of medicine and surgery. 1. Aseptic techniques: Used in microbiological research, the preservation of food and the prevention of the disease. 2. Sterile apparatus and culture media: Laboratory work with pure cultures requires the use of sterile apparatus and culture media. 3. The need to avoid infecting the patient: Requires the use of equipment, instruments, dressings and parenteral drugs that are free from living microorganisms, or at least from those which may give rise to infection. Antiseptics are chemical agents applied to tissue to prevent infection by killing or inhibiting pathogen growth; they also reduce the total microbial population. Antiseptics are generally not as toxic as disinfectant because they must not destroy too much host tissue. Factors that Determine the Potency of Disinfectants 1. The concentration and stability of the agent. 2. Nature of the organism. 3. Time of action. 4. pH 5. Temperature. 6. The presence of organic (especially protein) or other interfering substances. 7. Nature of the item to be disinfected.