The Effects of Minimum Quantity Lubrication (MQL) on Machining Force, Temperature, and Residual Stress PDF
Document Details
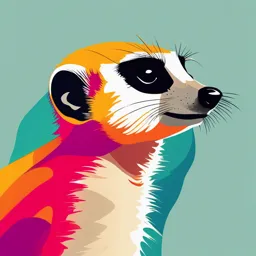
Uploaded by FriendlyOrphism
McMaster University
2014
Xia Ji, Beizhi Li, Xueping Zhang, and Steven Y. Liang
Tags
Related
- Minimum Staffing Levels PDF - Chesapeake Fire Department Policy
- Minimum Viable Product PDF
- OMB Chapter 8 Ed 3 Rev 33 - Operations Manual PDF
- Li et al. - 2024 - Minimum Quantity Lubrication (MQL) Review PDF
- A Technology Enabler for Green Machining: Minimum Quantity Lubrication (MQL) PDF
- Evaluation of Cryogenic Machining of Inconel 718 (PDF)
Summary
This article investigates the effects of minimum quantity lubrication (MQL) on machining parameters such as cutting force, temperature, and residual stress. The study compares MQL machining with dry and flood cooling machining. The results show that MQL can significantly reduce cutting force and temperature, supporting process planning for desired residual stress profiles.
Full Transcript
INTERNATIONAL JOURNAL OF PRECISION ENGINEERING AND MANUFACTURING Vol. 15, No. 11, pp. 2443-2451 NOVEMBER 2014 / 2443 DOI: 10.1007/s12541-014-0612-6 The Effects of Minimum Quantity Lubrication (MQL) on Machining Force, Temperature, and Residual Stres...
INTERNATIONAL JOURNAL OF PRECISION ENGINEERING AND MANUFACTURING Vol. 15, No. 11, pp. 2443-2451 NOVEMBER 2014 / 2443 DOI: 10.1007/s12541-014-0612-6 The Effects of Minimum Quantity Lubrication (MQL) on Machining Force, Temperature, and Residual Stress Xia Ji1,#, Beizhi Li1, Xueping Zhang2, and Steven Y. Liang3 1 School of Mechanical Engineering, Donghua University, Shanghai, 201620, China 2 School of Mechanical Engineering, Shanghai Jiao Tong University, Shanghai, 200240, China 3 George W. Woodruff School of Mechanical Engineering, George Institute of Technology, Atlanta, GA 30332-0405, USA # Corresponding Author / E-mail: [email protected], TEL: +86-021-6779-2564, FAX: +86-021-6779-2439 KEYWORDS: Cutting force, Cutting temperature, Residual stress, Minimum Quantity Lubrication (MQL) Minimum quantity lubrication (MQL) machining has achieved noticeable attention in both academic and industry research areas due to its minimum costs and maximum environmental protection. This paper focuses on the analysis of the effects of MQL parameters such as the flow rate of lubricant and the air-oil mixture ratio on cutting performances in terms of cutting force, cutting temperature, and residual stress. Additionally, the cutting performances in MQL machining are also compared with the dry and flood cooling machining. The results show that the cutting fluid can considerably reduce the cutting force and cutting temperature in machining. For MQL machining, there is a maximum effective flow rate of lubricant and it is influenced by the cutting speed. When the flow rate of lubricant is beyond the maximum effective value, the air-oil mixture ratio will no longer affect the cutting performances in machining. This research can support the process planning in achieving the desired residual stress profile by strategically adjusting the MQL parameters. Manuscript received: May 4, 2014 / Revised: July 14, 2014 / Accepted: July 15, 2014 comparable to the conventional flood cooling machining.2-5 Therefore, NOMENCLATURE the implementation of MQL machining as a viable alternative to traditional flood cooling machining is an important research area. f = feed rate A large number of studies have appeared in the literature to Fc = cutting force investigate the effects of MQL on cutting performances in terms of the Ft = thrust force cutting force,6-8 cutting temperature,9-12 tool wear,6,13 dimensional accuracy,9,14 and surface finish of machined components1,14,15 in various machining processes, such as turning,2,7,9 milling,3,16 drilling,17 and grinding.4,18-20 As for the cutting force, experimental results show that 1. Introduction there is a considerable reduction in cutting force components for the MQL technique as compared to dry cutting and flood cooling Reducing the production costs and improving the product quality machining.21 But for MQL machining, the cutting forces decrease with are the effective ways to strengthen the competitiveness of enterprises. the increase of lubricant within a certain range, and beyond this range This need gave birth to a new technology called minimum quantity the reduction is no longer significant.22 Compared with dry machining, lubrication (MQL). MQL machining refers to the use of a small amount MQL enables substantial reduction in cutting temperature, which of cutting fluid, and has achieved noticeable attention in academic and improves the chip-tool interaction and maintains sharpness of the industry research areas. This is because the usage of minimal quantities cutting edges.9 But comparing with flood cooling machining, the of fluid in MQL machining can definitely reduce the cost of using cooling effect of MQL in grinding is not as good as lubrication effect.18 cutting fluids and the auxiliary equipment.1,2 In addition, the available In addition, the experimental results show that MQL can significantly researches indicate that under certain experimental conditions, the extend the tool life compared to dry condition.4 The results of turning MQL machining has better performances than dry machining, and is AISI 1040 steel show that the application of MQL improves the © KSPE and Springer 2014 2444 / NOVEMBER 2014 INTERNATIONAL JOURNAL OF PRECISION ENGINEERING AND MANUFACTURING Vol. 15, No. 11 dimensional accuracy due to the reduction of wear and damage at the tool tip.9 Surface roughness obtained in MQL is comparable to that of flood cooling, while it is better than that of dry cutting.21 The superficial residual stresses of the machined components under different lubrication conditions are analyzed by experimental results in turning of AISI 52100. Results show that the tool nose radius and feed rate are the most influencing parameters on both maximum and minimum principal stresses, while the lubrication is the least important factor.23 A predictive model of residual stress in MQL machining is developed by the analytical method, and the effects of MQL on cutting force, cutting temperature, and residual stress are analyzed based on the analytical predictive model.24,25 It is worth noting that while a large number of articles have Fig. 1 Experimental setup appeared regarding the effects of lubrication condition on machinability characteristics such as cutting force, cutting temperature, tool wear, and the surface finish of the machined components, only a few articles have been published on the analysis of residual stress in MQL machining, even though the surface residual stresses on the final part is critical to the function behavior of the workpiece. What is more, the existing researches of residual stress in MQL machining mainly focus on the effects of cutting parameter and tool geometry on the cutting performances, few of them investigate the effects of MQL parameters. Therefore, more investigations on how machining-induced residual stresses vary with MQL could supply an effective way to control the quality of machined components through MQL. This paper presents an experimental investigation of cutting force, cutting temperature, and residual stresses in MQL machining. Unlike the previous research, this paper focuses on the analysis of the MQL Fig. 2 MQL supply system parameters such as flow rate of lubricant and air-oil mixture ratio, but not the cutting parameters and tool geometry. Additionally, the cutting performances in MQL machining are also compared with dry 4.775 mm. machining and the flood cooling machining. For MQL machining, the air-oil mixture was supplied by a UNIST system at a pressure of 40 psi. Coolube 2210, a vegetable oil, was chosen as the cutting fluid. The position and the angle of the MQL 2. Experimental Procedure nozzle were adjusted by experimental observation to avoid being blocked by chips, as shown in Fig. 2. Different flow rates of lubricant 2.1 Cutting condition and three different air-oil mixture ratios were selected to investigate the Experiments were carried out by orthogonal turning a tube of AISI effects of MQL parameters on the cutting performances. The fluid 4130 alloy steel with 63.5 mm outside diameter and 4.775 mm utilized for flood cooling machining was an emulsion of water and oil thickness in a Hardinge T42SP CNC turning center. Before starting the (Coolube 2210) ejected on the wokrpiece with a flow rate of 2.5 l/min. tests all the workpieces, coming from the same batch, were stress relieved in order to eliminate all the residual stresses induced in the 2.2 Measurement system material by previous production processes. During the experiment, the The cutting performances in terms of the cutting force, cutting workpiece was held by a three-jaw chuck as shown in Fig. 1. The temperature, and residual stress under different cutting conditions were carbide tool insert (N123K2-0600-0004-CR 1125, SANDVIK) with measured. The cutting forces were measured by a tool-post PVD coating was mounted on a standard SANDVIK LC123K08- dynamometer (Kistler 9257B), as shown in Fig. 1. The cutting 2525CM tool holder, which resulted in the 50 rake angle and 110 temperatures in machining process were measured by thermocouple clearance angle. The tool edge radius is about 40 mm which is supplied and thermal camera. The thermocouple is located underneath the tool by the tool supplier. insert with a distance of 4.3mm from the tool tip. It was covered by To evaluate the cutting performances under different cutting thermal conductive composite as indicated in Fig. 3. The thermal conditions, representative levels of cutting parameters are selected camera (Flir SC6000) was fixed in front of the lathe, as shown in Fig. based on the results of sensitivity analysis of the cutting performance. 4. For safety considerations, the glass door of the lathe must be kept The selected cutting parameters were as follows: the cutting speed with closed during the flood cooling machining, while the infrared light 1.049 m/s, 2.098 m/s, and 3.147 m/s; the feed rate with 0.0508 mm/rev, cannot go through the glass door of the lathe. Therefore, during the 0.1016 mm/rev, and 0.1524 mm/rev; the width of cut is a constant with actual experiment process, the thermal camera only recorded the INTERNATIONAL JOURNAL OF PRECISION ENGINEERING AND MANUFACTURING Vol. 15, No. 11 NOVEMBER 2014 / 2445 Fig. 3 Position of thermocouple Fig. 5 The schematic of residual stress measurement Fig. 4 Location of thermal camera temperature history under dry and MQL machining. The residual stresses are measured by the X-ray diffractometer (ProtoLXR type). In order to obtain the residual stress distribution along the depth into the workpiece, several points are subjected to different degrees of corrosion by different etching times. The corrosive depth of each point is measured by optical profilometer. The schematic of residual stress measurement for the workpiece is shown in Fig. 5. 3. Experimental Results and Discussion 3.1 Cutting force Fig. 6 Comparisons of cutting force in dry, MQL, and flood cooling In order to explore the effects of lubrication conditions in the machining machining process, keep the cutting parameters invariant, the measured cutting forces under dry, MQL, and flood conditions are compared as shown in Fig. 6. In this analysis, the cutting speed is 3.147 m/s, the magnitude with the increase of feed rate. This is because when the feed flow rate of lubricant is 48 ml/h, the width of cut is 4.775 mm, and the rate increases, the cut area increases, so the cutting forces will increase air-oil mixture ratio is medium quantity. From the comparison results, in magnitude as well. it is found that the cutting force in dry machining is the largest among In actual experiments, the MQL parameters include the flow rate of three different lubrication conditions. The cutting force in MQL lubricant, the air pressure, the air-oil mixture ratio, the diameter and the machining is slightly larger than the flood cooling machining under position of the MQL nozzle, etc. In this analysis, the flow rate of lower feed rate condition. But in general, there is no significant lubricant and the air-oil mixture ratio are changed to investigate the difference in the cutting force between MQL and flood cooling effects of MQL parameters on cutting forces. First, the measured machining. The results in Fig. 6 also show that, no matter which cutting forces under different flow rates of lubricant are shown in Fig. lubrication condition is applied, the cutting forces increase in 7 and Fig. 8. In this analysis, the width of cut is 4.775 mm, and the air- 2446 / NOVEMBER 2014 INTERNATIONAL JOURNAL OF PRECISION ENGINEERING AND MANUFACTURING Vol. 15, No. 11 Fig. 7 Relationship between cutting force and flow rate of lubricant Fig. 9 Relationship between cutting force and air-oil mixture ratio (Cutting speed = 3.147 m/s) (Cutting speed = 2.098 m/s, flow rate of lubricant = 16 ml/h) to fill the peak and valley of tool-workpiece contact area. The experimental results are consist with the conclusion of Zhang.26 From the comparisons between Fig. 7 and Fig. 8, it is found that when the cutting speed is 3.147 m/s, the maximum effective flow rate of lubricant is 48 ml/h. While the cutting speed is 1.049 m/s, the maximum effective flow rate of lubricant is about 32 ml/h. From the comparisons between Fig. 7 and Fig. 8, it is found that for the experimental value explored, the maximum effective flow rate of lubricant is slightly influenced by the cutting speed, but not by the feed rate. In order to investigate the relationship between the air-oil mixture ratio and the cutting force, three different air-oil mixture ratios and two feed rates are selected. The measured cutting forces under different air- Fig. 8 Relationship between cutting force and flow rate of lubricant oil mixture ratios are shown in Fig. 9 and Fig. 10. Results in Fig. 9 (Cutting speed = 1.049 m/s, feed rate = 0.1524 mm/rev) show that under low flow rate condition, a low quantity or high quantity air-oil mixture ratio is not beneficial to reduce the cutting force oil mixture ratio is medium quantity. Three feed rates and two cutting in MQL machining. From the results in Fig. 10, it is found that when speeds are chosen. From the experimental results shown in Fig. 7 and the flow rate of lubricant is 48 ml/h, the relationship between the Fig. 8, it is found that the cutting force decreases in magnitude with the cutting force and air-oil mixture ratio is not clear. increase of flow rate at first. When the flow rate attains a certain From the comparisons between Fig. 9 and Fig. 10, it is found that magnitude, the cutting force no longer changes. This is because the the when the flow rate of lubricant is 48 ml/h, the air-oil mixture ratio boundary lubrication model exists in MQL machining. Under boundary has minimal effect on the cutting force. This is because when the flow lubrication condition, the lubrication effect is not only depended on rate of lubricant is beyond the maximum effective magnitude, no amount of lubricant, but also determined by the property of lubricant, matter how the air-oil mixture ratio changes, the effective amount of the material property of the workpiece and the tool, the surface finish lubricant permeated into the cutting zone will not change. Therefore, of the workpiece, etc. The maximum effective amount of lubricant is for the values explored, when the flow rate of lubricant is beyond 48 INTERNATIONAL JOURNAL OF PRECISION ENGINEERING AND MANUFACTURING Vol. 15, No. 11 NOVEMBER 2014 / 2447 Fig. 10 Relationship between cutting force and air-oil mixture ratio Fig. 11 Comparisons of cutting temperature under dry, MQL, and (Cutting speed = 2.098 m/s, flow rate of lubricant = 48 ml/h) flood condition ml/h, the cutting force will not be significantly affected by the air-oil mixture ratio. According to the analysis, the flow rate in Fig. 9 is not beyond the maximum effective value. Therefore, the cutting force will be influenced by the air-oil mixture ratio. When the air-oil mixture ratio is too low, the amount of lubricant passed through the cutting zone in the unit time is larger than the air, and the lubrication effect is more effective than the cooling effect. When the air-oil mixture ratio is too high, the volume of air in the unit time is larger than the lubricant, and the cooling effect is more effective than the lubrication effect. While the air-oil mixture ratio is medium, the lubrication effect and the cooling effect can reach a balance. Therefore, the cutting force is lowest under medium air-oil mixture ratio. Fig. 12 Relationship between cutting temperature and flow rate of lubricant 3.2 Cutting temperature The cutting temperatures measured by thermocouple and thermal camera under dry, MQL, and flood cooling machining are shown in measurement is not long enough to reach the steady state. The thermal Fig. 11. In this analysis, the cutting speed is 2.098 m/s, the flow rate camera records the instantaneous cutting temperature where the of lubricant in MQL is 16 ml/h, and the air-oil mixture ratio is medium infrared light radiates, while the temperature measured by quantity. Results indicate whether by thermocouple or by thermal thermocouple is non-instantaneous due to the conductivity of the camera, the flood cooling machining gets the lowest cutting workpiece. Therefore, the cutting temperatures measured by temperature among the three lubrication conditions. Compared to the thermocouple will not be discussed in the following. results measured by thermocouple and by thermal camera, it is found In order to investigate the effects of MQL parameters on the cutting that the cutting temperature measured by thermocouple is significantly temperature, the temperatures measured by thermal camera under lower than the results measured by thermal camera. The discrepancy different flow rates of lubricant are shown in Fig. 12. In this analysis, may be attributed to the fact that the cutting time for temperature the cutting speed is 3.147 m/s, and the air-oil mixture ratio is medium 2448 / NOVEMBER 2014 INTERNATIONAL JOURNAL OF PRECISION ENGINEERING AND MANUFACTURING Vol. 15, No. 11 Fig. 13 Relationship between cutting temperature and air-oil mixture Fig. 14 Comparisons of residual stress distribution under dry, MQL, ratio and flood machining quantity. Results indicate that for the experimental value explored, the MQL machining. The results are consistent with the analysis of cutting cutting temperature decreases with the increase of flow rate of temperature. So it is concluded that the superficial residual stress of the lubricant, but the trend is not significant. This is because the continuous machined workpiece is mainly determined by the thermal stress chip produced during the machining process may block the sprayed air- produced by cutting temperature in the machining process. oil mixture, and it will affect the cooling effect of MQL in the From the comparison results in Fig. 14(a), it is also found that the machining process. application of cutting fluid can significantly reduce the depth of The cutting temperatures measured by the thermal camera under maximum compressive residual stress. In the cutting direction, the different air-oil mixture ratios are shown in Fig. 13. In this analysis, the maximum compressive residual stress in dry machining is the largest in cutting speed is 2.098 m/s. Results indicate that the lower cutting magnitude among three lubrication conditions, and this trend is not temperature is obtained under the high quantitatively of air-oil mixture clear in the transverse direction. It is concluded that the depth and ratio. It is also found that when the flow rate of lubricant is 48 ml/h, magnitude of the maximum compressive residual stress in the the air-oil mixture ratio has little effect on the cutting temperature. The machined workpiece mainly depend on the mechanical stress produced result is consistent with the cutting force analysis. This may be because by the cutting force during the machining process. In addition, the under this cutting condition, the flow rate of lubricant is beyond the results in Fig. 14 show that the penetration depth of residual stress maximum effective value. Therefore, the cutting temperature and decreases with the increase of cutting fluid in cutting direction, but is cutting force will no longer be influenced by the air-oil mixture ratio. not very clear in the transverse direction. This is because the penetration depth of residual stress depends not only on the cutting 3.3 Residual stress force, but also on the tool geometry. In this analysis, the tool geometry First, the measured in-depth residual stresses under dry, MQL, and is the same under three lubrication conditions. flood machining are compared as shown in Fig. 14. In this analysis, the The measured in-depth residual stresses under different flow rates of cutting speed is 1.049 m/s, the feed rate is 0.0508 mm/rev, the flow rate lubricant are shown in Fig. 15. In this analysis, the cutting speed is of MQL is 16 ml/h, and the air-oil mixture ratio is medium quantity. 3.147 m/s, the feed rate is 0.1016 mm/rev, and the air-oil mixture ratio Results indicate that the flood cooling machining obtains the minimum is medium quantity. Results show that the depth of maximum tensile residual stress at the surface of the machined workpiece among compressive residual stress decreases in magnitude with the increase of three lubrication conditions, while there is no significant difference of the flow rate of lubricant. This trend is consistent with the analysis of the superficial tensile residual stresses between the dry machining and cutting force, and it can be inferred that the depth of maximum INTERNATIONAL JOURNAL OF PRECISION ENGINEERING AND MANUFACTURING Vol. 15, No. 11 NOVEMBER 2014 / 2449 Fig. 15 Residual stress distributions under different flow rates of Fig. 16 Residual stress distributions under different air-oil mixture lubricant ratios compressive residual stress mainly depends on the mechanical stress compared with the dry and flood cooling machining. produced by cutting force during the machining process. Results also The comparisons of experimental results show that the application show that, for the experimental value explored, the flow rate of of cutting fluid considerably reduces the cutting force and cutting lubricant has little effect on the residual stress at the surface of the temperature in machining. However, there is no significant difference machined wokpiece and the maximum compressive residual stress in in cutting force between MQL and flood cooling machining. For MQL MQL machining. machining, there exists a maximum effective flow rate of lubricant in The measured in-depth residual stresses under different air-oil machining, and it is slightly influenced by the cutting speed, but not by mixture ratios are shown in Fig. 16. In this analysis, the cutting speed feed rate. When the flow rate of lubricant is beyond the maximum is 2.098 m/s, the feed rate is 0.1016 mm/rev, and the flow rate is 48 ml/ effective value, the air-oil mixture ratio will no longer affect the cutting h. Results show that the air-oil mixture ratio has little effect on the force, cutting temperature, and residual stress. residual stress distribution. According to the analysis of cutting force Experimental results show that the residual stress at the surface of and cutting temperature in Section 3.1 and 3.2, when the flow rate of the machined workpiece mainly depends on the thermal stress lubricant is beyond the maximum effective value, the air-oil mixture produced by the cutting temperature in machining process, and ratio will no longer affect the cutting force and cutting temperature, decreases with the application of cutting fluid. The depth and while these two attributes are the main sources of residual stress in magnitude of maximum compressive residual stress are more machining. influenced by the mechanical stress produced by cutting force in machining. The penetration depth of residual stress depends not only on the cutting force but also on the tool geometry. 4. Conclusion The results of this study can be used to support parameter optimization and process planning in achieving desired residual stress This research presented an experimental investigation of cutting profile in industry. force, cutting temperature, and residual stress in MQL machining. A specific analysis of the effects of MQL parameters such as flow rate of lubricant and air-oil mixture ratio on cutting force, cutting temperature, ACKNOWLEDGEMENT and residual stress are discussed based on the measured results. Additionally, the cutting performances in MQL machining are The authors would like to thank the National Natural Science 2450 / NOVEMBER 2014 INTERNATIONAL JOURNAL OF PRECISION ENGINEERING AND MANUFACTURING Vol. 15, No. 11 Foundation of China (No. 51175331) for supporting the research. Lubrication with Al2O3 Nanoparticles on Surface Roughness, Tool Wear and Temperature Dissipation in Machining Inconel 600 Alloy,” Proceedings of the Institution of Mechanical Engineers, Part N: REFERENCES Journal of Nanoengineering and Nanosystems, Vol. 228, No. 1, pp. 3-16, 2011. 1. Leppert, T., “Effect of Cooling and Lubrication Conditions on 13. Marksberry, P. and Jawahir, I., “A Comprehensive Tool-Wear/Tool- Surface Topography and Turning Process of C45 Steel,” International Life Performance Model in the Evaluation of NDM (Near Dry Journal of Machine Tools and Manufacture, Vol. 51, No. 2, pp. 120- Machining) for Sustainable Manufacturing,” International Journal of 126, 2011. Machine Tools and Manufacture, Vol. 48, No. 7, pp. 878-886, 2008. 2. Dhar, N., Ahmed, M., and Islam, S., “An Experimental Investigation on Effect of Minimum Quantity Lubrication in Machining AISI 1040 14. Islam, M. N., “Effect of Additional Factors on Dimensional Accuracy Steel,” International Journal of Machine Tools and Manufacture, and Surface Finish of Turned Parts,” Machining Science and Vol. 47, No. 5, pp. 748-753, 2007. Technology, Vol. 17, No. 1, pp. 145-162, 2013. 3. Yuan, S. M., Yan, L. T., Liu, W. D., and Liu, Q., “Investigation of 15. Sreejith, P., “Machining of 6061 Aluminium Alloy with MQL, Dry Machinability in Minimum Quantity Lubrication Milling of GH4169 and Flooded Lubricant Conditions,” Materials Letters, Vol. 62, No. Aerospace Superalloy,” Applied Mechanics and Materials, Vol. 34, 2, pp. 276-278, 2008. No. pp. 666-670, 2010. 16. Zhong, W., Zhao, D., and Wang, X., “A Comparative Study on Dry 4. Li, K. M. and Lin, C. P., “Study on Minimum Quantity Lubrication Milling and Little Quantity Lubricant Milling based on Vibration in Micro-Grinding,” The International Journal of Advanced Signals,” International Journal of Machine Tools and Manufacture, Manufacturing Technology, Vol. 62, No. 1-4, pp. 99-105, 2012. Vol. 50, No. 12, pp. 1057-1064, 2010. 5. Nguyen, T. K., Do, I., and Kwon, P., “A Tribological Study of 17. Kilickap, E., Huseyinoglu, M., and Ozel, C., “Empirical Study Vegetable Oil Enhanced by Nano-Platelets and Implication in MQL Regarding the Effects of Minimum Quantity Lubricant Utilization Machining,” Int. J. Precis. Eng. Manuf., Vol. 13, No. 7, pp. 1077- on Performance Characteristics in the Drilling of Al 7075,” Journal 1083, 2012. of the Brazilian Society of Mechanical Sciences and Engineering, Vol. 33, No. 1, pp. 52-57, 2011. 6. Yuan, S. M., Yan, L. T., Liu, W. D., and Liu, Q., “Effects of Cooling Air Temperature on Cryogenic Machining of Ti-6Al-4V Alloy,” 18. Hadad, M. and Sadeghi, B., “Thermal Analysis of Minimum Quantity Journal of Materials Processing Technology, Vol. 211, No. 3, pp. Lubrication-MQL Grinding Process,” International Journal of 356-362, 2011. Machine Tools and Manufacture, Vol. 63, No. pp. 1-15, 2012. 7. Varadarajan, A., Philip, P., and Ramamoorthy, B., “Investigations on 19. Tawakoli, T., Hadad, M. J., and Sadeghi, M. H., “Influence of Oil Hard Turning with Minimal Cutting Fluid Application (HTMF) and Mist Parameters on Minimum Quantity Lubrication-MQL Grinding Its Comparison with Dry and Wet Turning,” International Journal of Process,” International Journal of Machine Tools and Manufacture, Machine Tools and Manufacture, Vol. 42, No. 2, pp. 193-200, 2002. Vol. 50, No. 6, pp. 521-531, 2010. 8. Liu, Z. Q., Xu, J., Han, S., and Chen, M., “A Coupling Method of 20. Lee, P. H., Nam, J. S., Li, C., and Lee, S. W., “An Experimental Study Response Surfaces (CRSM) for Cutting Parameters Optimization in on Micro-Grinding Process with Nanofluid Minimum Quantity Machining Titanium Alloy under Minimum Quantity Lubrication Lubrication (MQL),” Int. J. Precis. Eng. Manuf., Vol. 13, No. 3, pp. (MQL) Condition,” Int. J. Precis. Eng. Manuf., Vol. 14, No. 5, pp. 331-338, 2012. 693-702, 2013. 21. Rahman, M., Senthil Kumar, A., and Salam, M., “Experimental 9. Dhar, N., Islam, M., Islam, S., and Mithu, M., “The Influence of Evaluation on the Effect of Minimal Quantities of Lubricant in Minimum Quantity of Lubrication (MQL) on Cutting Temperature, Milling,” International Journal of Machine Tools and Manufacture, Chip and Dimensional Accuracy in Turning AISI-1040 Steel,” Journal Vol. 42, No. 5, pp. 539-547, 2002. of Materials Processing Technology, Vol. 171, No. 1, pp. 93-99, 2006. 22. Cai, X. J., Liu, Z. Q., Chen, M., and An, Q. L., “An Experimental Investigation on Effects of Minimum Quantity Lubrication Oil 10. Li, K. M. and Liang, S. Y., “Modeling of Cutting Temperature in Near Supply Rate in High-Speed End Milling of Ti-6Al-4V,” Proceedings Dry Machining,” Journal of Manufacturing Science and Engineering, of the Institution of Mechanical Engineers, Part B: Journal of Vol. 128, No. 2, pp. 416-424, 2006. Engineering Manufacture, Vol. 226, No. 11, pp. 1784-1792, 2012. 11. Ueda, T., Hosokawa, A., and Yamada, K., “Effect of Oil Mist on 23. Attanasio, A., Ceretti, E., Gelfi, M., and Giardini, C., “Experimental Tool Temperature in Cutting,” Journal of Manufacturing Science and Evaluation of Lubricant Influence on Residual Stress in Turning Engineering, Vol. 128, No. 1, pp. 130-135, 2006. Operations,” International Journal of Machining and Machinability 12. Vasu, V. and Reddy, G. P. K., “Effect of Minimum Quantity of Materials, Vol. 6, No. 1, pp. 106-119, 2009. INTERNATIONAL JOURNAL OF PRECISION ENGINEERING AND MANUFACTURING Vol. 15, No. 11 NOVEMBER 2014 / 2451 24. Ji, X., Zhang, X., and Liang, S. Y., “Predictive Modeling of Residual Stress in Minimum Quantity Lubrication Machining,” The International Journal of Advanced Manufacturing Technology, Vol. 70, No. 9-12, pp. 2159-2168, 2014. 25. Ji, X., Zhang, X. P., Li, B. Z., and Liang, S. Y., “Predictive Modeling of Minimum Quantity Lubrication: Cutting Force, Temperature and Residual Stress,” Applied Mechanics and Materials, Vol. 365, No. pp. 1181-1184, 2013. 26. Zhang, C. Y., “Study on the Mechanism of MQL Cutting and Its Application Fundament,” Ph.D. Thesis, Department of Mechanical Manufacturing and Automation, Jiangsu University, 2008.