Evaluation of Cryogenic Machining of Inconel 718 (PDF)
Document Details
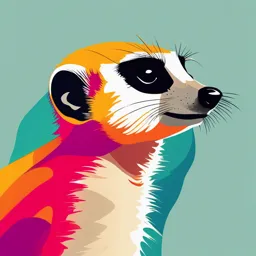
Uploaded by FriendlyOrphism
McMaster University
Yusuf Kaynak
Tags
Summary
This article evaluates the machining performance of Inconel 718 using cryogenic machining and compares it with dry and minimum quantity lubrication (MQL) machining. Experimental data on cutting forces, tool wear, and surface quality is presented to assess the advantages of cryogenic machining for high-temperature aerospace alloys.
Full Transcript
Int J Adv Manuf Technol (2014) 72:919–933 DOI 10.1007/s00170-014-5683-0 ORIGINAL ARTICLE Evaluation of machining performance in cryogenic machining of Inconel 718 and comparison with dry and MQL machining Yusuf Kaynak Received: 12 September 2013 / Accepted: 31 January 2014 / Published online: 2...
Int J Adv Manuf Technol (2014) 72:919–933 DOI 10.1007/s00170-014-5683-0 ORIGINAL ARTICLE Evaluation of machining performance in cryogenic machining of Inconel 718 and comparison with dry and MQL machining Yusuf Kaynak Received: 12 September 2013 / Accepted: 31 January 2014 / Published online: 2 March 2014 # Springer-Verlag London 2014 Abstract There has been significant work on establishing 1 Introduction relationships between machining performance and the cutting parameters for various work materials. Recent trends in ma- About 50 wt% of aero-engine alloys are nickel-based alloys chining research show that major efforts are being made to. They exhibit higher strength to weight ratio, relative to understand the impact of various cooling/lubrication methods steel that is denser. Nickel-based superalloys are also used on machining performance and surface integrity characteris- for other applications such as marine equipment, nuclear tics, all aimed at improving process and product performance. reactors, etc. They are used in these aggressive environ- This study presents the experimental results of cryogenic ments because of their ability to maintain high resistance to machining of Inconel 718, a high-temperature aerospace alloy, corrosion, mechanical and thermal fatigue, mechanical and and comparison of its performance in dry and minimum thermal shock, creep, and erosion at elevated temperatures. quantity lubrication machining. Experimental data on force Inconel 718 is the most frequently used of nickel-based alloy components, progressive tool wear parameters such as flank. According to the literature, Inconel 718 does not have wear, notch wear, crater wear, cutting temperature, chip mor- good machinability when it is compared with aluminum al- phology, and surface roughness/topography of machined sam- loys, mild steel, or stainless steels due to its mechanical and ples are presented. New findings show that cryogenic machin- thermal properties such as strain-hardening characteristics and ing is a promising research direction for machining of high- poor thermal conductivity, etc.. High temperatures gener- temperature aerospace alloy, Inconel 718, as it offers im- ated in cutting process reduce the tool life, deteriorate the proved machining performance in terms of reduced tool wear, surface quality of machined parts, etc.; therefore, the machin- temperature, and improved surface quality. It was also found ing industry has been using oil-based or water-based emulsion that the number of nozzles in cryogenic machining plays a cutting fluids to cool and lubricate the machining process. vital role in controlling cutting forces and power consumption Unfortunately, conventional cutting fluids create both health in cryogenic machining of Inconel 718. and environmental problems, and also increase production costs. For these reasons, specific methods and well- defined sustainability elements were proposed for improving Keywords Cryogenic machining. MQL. Machining the machining processes in materials to make them more sus- performance. Inconel 718 tainable. Elements included machining cost, environmental friendliness, energy consumption, waste management, opera- tional safety, and personal health. Meeting requirements of these elements assists in simultaneously reducing environmen- tal problems and improving machining performance. In ma- Y. Kaynak (*) chining of Inconel 718 alloy, these elements certainly need to be Mechanics and Machine Elements Division, University of Marmara, taken into account because machining is one of the significant Goztepe Campus, Kadikoy 34722, Istanbul, Turkey processing steps to make products from Inconel 718 alloys for e-mail: [email protected] aerospace components. These elements are also a driving force Y. Kaynak in obtaining more sustainable products. A very strong interrela- e-mail: [email protected] tionship exists between sustainable process and product. In 920 Int J Adv Manuf Technol (2014) 72:919–933 order to produce more sustainable products, the first significant the effectiveness of liquid nitrogen, a medium commonly used step is to focus on process that is required to produce compo- in cryogenic machining. Since cryogenic cooling is proposed nents. Then, achieving improvements in machining as an alternative approach to offer cooling as well as lubrica- performance through a greater understanding of the prevalent tion, it should be tested and compared with MQL in machin- relationships between the effective parameters in the process ing of different materials. To the best knowledge of the author, and machining outputs becomes important. This requires more there is no systematic study comparing cryogenic cooling with focused and systematic research on machining of Inconel 718. MQL and dry cutting in machining of Inconel 718 alloy, To simultaneously improve the machining performance of these particularly considering the machining performance such as alloys and reduce environmental concerns, manufacturing cost, cutting forces, progressive tool wear, cutting temperature, chip and other previously stated elements, one of the proposed morphology, etc. Machining is one of the significant effective ways is to utilize an alternative cooling/lubricating manufacturing processes to produce functional components approach instead of traditional way of lubricating/cooling in for aerospace industries, and Inconel 718 is a major material the machining process. During the last decades, there have been for aerospace engine application; hence, a greater in-depth major efforts in developing and implementing more effective understanding of the machining performance of this material cooling/lubrication methods in machining of hard-to-cut aero- under different cooling/lubricating conditions would be ex- space alloys, including Inconel 718. The most common pected to make a significant contribution to this area from the among these methods were high pressure coolant applications, scientific and practical applications points of view. In this minimum quantity lubrication (MQL), and more recently, cryo- study, the influence of cooling/lubrication is the focused topic genic cooling [5, 6]. These methods were proposed for improv- due to the growing trends in utilizing alternative cooling in ing the machining performance of Inconel 718 alloys and machining processes, and the associated need for greater reducing the production costs, and to make the processes more understanding of the fundamental behavior of the coolants/ environmentally friendly [1, 2, 7, 8]. The effect of MQL ma- lubricants. Therefore, the major aim of this work is to contrib- chining including flow rate and nozzle position on machining ute to the understanding of cryogenic machining-induced performance of Inconel 718 and the comparison of MQL with machining performance of aerospace material, Inconel 718, dry and wet machining of this alloy have been extensively and comparison of its performance with dry and MQL studied [7, 9, 10]. However, few studies focused on cryogenic conditions. machining of Inconel 718 and its effects on machining perfor- mance as it was presented in Table 1. Studies presented in Table 1 reported that cryogenic machining helps to reduce tool 2 Experimental procedure wear by reducing cutting temperature and surface roughness by reducing thermal effects and tool wear in comparison with dry The work material used in this study is Inconel 718 AMS and conventional machining. 5663M with a diameter of 32 mm, which was hot rolled, As reported in the literature review, although MQL has solution-treated, and aged. The cutting experiments were con- been extensively studied in machining as an effective and ducted on a Mazak CNC turning center. Uncoated 890 grade timely alternative to flood cooling, in recent years the overall carbide inserts were used with ISO designation CNMG120408, superior performance of the use of cryogenic methodologies whose edge radii were measured and found between 21 and has placed a stronger emphasis on the basic understanding of 24 μm. An example of measured edge radius of one of the used Table 1 Summary of studies on the effect of cryogenic cooling on machining performance measures in turning process of Inconel 718 Measurements Cooling/lubricating Cutting forces Tool wear/tool life Cutting temp. Chip morphology Surface roughness Cryogenic, dry ✓ ✓ Conventional, plasma hybrid ✓ ✓ ✓ (plasma + cryogenic) Conventional, high pressure ✓ jet assisted, cryogenic Dry, cryogenic, MQL, cryogenic + MQL ✓ Cryogenic, dry ✓ Conventional, hybrid (plasma, cryogenic, ✓ ✓ ultrasonic vibration assisted) Dry, MQL, cryogenic, cryogenic + MQL ✓ ✓ ✓ Int J Adv Manuf Technol (2014) 72:919–933 921 inserts is shown in Fig. 1. The tool holder was MCGNL-164D Table 2 Table of experimental cutting conditions with 95° approach angle,−6° normal rake angle, and −6° end Exp. # ap f Vc Exp. # ap f Vc cutting edge angle. Machining experiments with the feed rate, [mm] [mm/rev] [m/min] [mm] [mm/rev] [m/min] f=0.075 mm/rev, cutting speed, V=60 and 120 m/min, and depth of cut, ap =0.8 mm, were conducted under dry, MQL, and 1 0.20 0.18 90 9 1.07 0.18 30 cryogenic cooling conditions. 2 0.20 0.25 120 10 1.07 0.05 90 It has to be noted that Pusavec et al. in their study 3 0.20 0.05 30 11 1.07 0.25 60 conducted 16 machining experiments using same work mate- 4 0.20 0.12 60 12 1.07 0.12 120 rial, cutting tools, and experimental setup as those are given 5 0.63 0.05 60 13 1.50 0.05 120 above. Their experiments were design based on an orthogonal 6 0.63 0.18 120 14 1.50 0.12 90 matrix. Their experimental cutting conditions are shown in 7 0.63 0.25 90 15 1.50 0.18 60 Table 2. 8 0.63 0.12 30 16 1.50 0.25 30 Pusavec et al. determined optimal machining condi- tions for Inconel 718 work material and cutting tools using genetic algorithm approach. In this current study, further tests ZYGO 3D-New View 7300, respectively. Tool wear and chip were conducted. In this current study, selected machining morphology were measured with an optical microscope. Tem- parameters except 120 m/min cutting speed have been found perature was measured using FLIR SC7650 infrared camera. to be within the optimal range of parameters for machining As a result of calibration, the emissivity of chip was found as Inconel 718 for the given cutting tool. For the MQL 0.48 and implemented to measure temperature. A direction of experiments, commercially available UNIST Coolube delivering of liquid nitrogen and applied MQL’s direction are 2210EP metalworking lubricant was used at a flow rate of shown in Fig. 2. 60 ml/h and approximately under 0.4 MPa air pressure. In the cryogenic machining process, liquid nitrogen was applied to the cutting process under 1.5 MPa pressure and approximately10 g/s mass flow rate at the tool’s flank face, 3 Experimental results and this is expected to influence the force components mea- sured. Therefore, flank face cooling was compared with flank 3.1 Cutting forces and rake face cooling by taking force measurements. In this experimental work, the applied pressure was kept at the same Force components are the fundamental and significant indica- level as in all previous cryogenic tests. tors of the power requirements and energy consumption in In the experimental work, cutting force components and machining processes. Force components are influenced by surface roughness were measured with three-component pie- many parameters, such as tool–chip friction, properties of zoelectric dynamometer and interferometry optical profiler work material, tool material and the coatings, and the geom- etry of the cutting tool, cutting conditions, cooling/lubricating, etc. This current work presents the effects of cooling/ lubricating on cutting force variation. According to experi- mental results shown in Fig. 3, applying MQL in cutting Inconel 718 yielded lower cutting forces in comparison with dry and cryogenic condition at low cutting speed of 60 m/min. In comparison with MQL, cryogenic cooling increased the components of radial force, feed force, and cutting force approximately 20, 36, and 25 %, respectively. The difference between dry and cryogenic cooling is less than 5 % in all three force components at this lower cutting speed, 60 m/min. However, at the higher cutting speed of 120 m/min, all three force components are lower compared to both, dry and MQL machining. Figure 4 shows the patterns of tool–chip contact in all three cooling/lubricating conditions at low speed of 60 m/min. These contact regions show significant variations. While the measured maximum contact length along the chip flow direc- Fig. 1 Measurement of cutting tool edge radius using ZYGO 3D-New tion is approximately the same, the measured cutting edge View 7300 optical profiler contact lengths (Lc) are 1.57 mm in dry condition, 1.36 mm in 922 Int J Adv Manuf Technol (2014) 72:919–933 Fig. 2 a Experimental setup, and a b b the directions of liquid nitrogen and MQL delivery Cutting tool Cryo rake face /MQL direction Tool holder Cryo flank face Inconel 718 Chip direction MQL conditions, and 1.69 mm in cryogenic cooling condition. addition, more consistent force values were recorded in cryo- The cutting edge contact length in cryogenic cooling condi- genic cooling. Variations over the cutting time in cryogenic tions is approximately 24 % larger than that in MQL condi- cooling were less than that of dry and MQL conditions. This tions. And this is in good agreement with the measured main observation was also attributed to tool wear progress during cutting force components for MQL and cryogenic machining, cutting. It needs to be noted that newly generated chip’s shown in Fig. 3. Also, as seen in Fig. 4, the chip side-spread behavior (i.e., strain hardening and/or thermal softening be- under cryogenic conditions seems to be slightly larger, and this havior due to changing cutting temperature, resulting from is most likely due to the pressure applied by the cryogenic different cooling/lubricating conditions), and consequently liquid jet on the tool face, which is very likely to sway the chip the effect of newly generated chips thermo-mechanical prop- flow. At higher cutting speed, however, the chip flow mecha- erties such as compressive stress, hydrostatic pressure, etc., is nism is likely to overpower the liquid application pressure not taken into account in the evaluation of recorded force while reducing the effective contact on the tool face between results; though, while it is also known that these factors play the tool and the chip, thus resulting in reduced forces. a role on determining force requirements. Under the lubrica- Increasing cutting speed under same cooling/lubricating tion condition, Williams and Tabor emphasized the point conditions substantially changed the force’s results as shown that chip is moving relative to the face of the tool, and there in Fig. 3. In higher cutting speed, cryogenic cooling helped to will be a hydrodynamic action sweeping the lubricant out of reduce force components as compared to dry and MQL con- the interface and this effect can be more pronounced as the ditions. This observation can be attributed to tool–chip contact chip speed increases. Hence, the higher the cutting speed, the length and tool wear, to be discussed in following section. As less effective the lubrication effect would be in the chip– seen in Fig. 13, in higher cutting speed, contact length is workpiece contact region. Also, only considering tool smaller than those measured in dry and almost same with wear may not be enough to explain the increased force com- MQL conditions. Recording maximum difference in feed ponents in MQL conditions at high speed. force among cryogenic, dry, and MQL was attributed to flank Cryogenic machining or cooling is a generic terminology wear rate at the nose of the tool. Increased flank wear led to used in the literature to define the use of applied liquid increasing contact area between tool and work material, and, nitrogen during the cutting process; however, a more specific consequently, increased friction force in feed direction. In definition is needed to better understand and analyze the obtained results. While it is possible to deliver liquid nitrogen Radial Feed Main cutting through multiple nozzles, one nozzle can be used to obtain 300 improved machining performance, and in the meantime re- 250 duce the amount of liquid nitrogen consumed during the cutting process. In this paper, unless otherwise stated, cryo- 200 Force (N) genic cooling means one nozzle and delivered from the flank 150 face of the cutting tool. Hong et al. [14, 15] investigated the impact of number of nozzles on machining performance of Ti 100 64 alloys, in particular, from the cutting temperature, tool life, 50 and chip breakability perspective. Their experimental results 0 show that the number of nozzles and direction of liquid Dry MQL Cryo Dry MQL Cryo nitrogen delivery play an important role in determining ma- V= 60 m/min V= 120 m/min chining performance of Ti 64 alloys and minimize the use of Fig. 3 Cutting force comparison under different cooling/lubricating con- liquid nitrogen. Although their work focused on Ti 64 alloy, ditions (f=0.075 mm/rev, ap =0.8 mm) more research is required to use liquid nitrogen more Int J Adv Manuf Technol (2014) 72:919–933 923 Fig. 4 Tool–chip contact area and length as a function of cooling/lubricating in machining of Inconel 718 (V=60 m/min., f=0.075 mm/rev, ap =0.8 mm) Dry MQL Cryogenic efficiently and, in the meantime, improve machining perfor- geometry can be among the major effective factors for the mance as much as possible for different materials too. There- delivery of liquid nitrogen. Although the effect of the type of fore, in addition to cryogenic cooling (one nozzle), cryogenic chip breakers and chip groove on tool wear, cutting forces, has rake and flank cooling (two nozzles) was also tested in this set been extensively studied by researchers [16–18], the effect of of experiments to compare their effect on force requirement. grooved tool on liquid nitrogen delivery and the resulting Positioning of nozzles has been already shown in Fig. 2. Force lubrication/cooling effect has not been addressed extensively measurements in this work show that the number of nozzles with the exception of work by Hong and Ding who plays a considerable role in determining force components in investigated the effect of chip breaker on the effectiveness of cryogenic machining. Depending on the number of nozzle, cryogenic rake cooling in machining of Ti 64, and concluded measured force values made remarkable difference at these that depending on its geometry, chip breaker influences the cutting conditions and selected material. Adding one more penetration of cryogenic cooling between tool and chip con- nozzle from rake face leads to approximately 15 to 25 %in- tact area. Therefore, they preferred specially designed chip crease in force components as shown in Fig. 5. breakers and delivered liquid nitrogen through such chip In order to better understand the reason for increased force breakers to reach the chip–tool contact area. However, components with liquid nitrogen delivery through two noz- specialized/customized tools are not so common, and also it zles, it is definitely necessary to more closely look at the raises the cost of machining process. Therefore, in this work, a cutting region. Based on the observation during the cutting commercially available tool, which has MF1 type chip breaker process, cryogenic cooling with one nozzle on the flank and chip groove, was selected. The plane view and the cross- surface of the cutting tool and the newly generated machined sectional profile of this chip breaker are shown in Fig. 6. surface was quite effective, while the liquid does barely reaches the unmachined surface of the work material. On the other hand, cryogenic flank and rake cooling (two nozzles delivering simultaneously) is not only effective in the region of machined surface and flank surface but it is also expected to penetrate the tool–chip contact area, and because of the deliv- ery method, it also reaches the unmachined work material as shown in the schematic of the effective region in Fig. 7. Depending on nozzles and direction of nozzles, cutting tool’s geometric features such as the chip breaker type and its a b Radial Feed Main cutting 300 250 200 Force (N) 150 100 a b 50 0 Cryo (one nozzle) Cryo (two nozzles) Fig. 5 Variation of cutting force components with one and two nozzles used in cryogenic machining (V=60 m/min) Fig. 6 Chip breaker geometry of cutting tool 924 Int J Adv Manuf Technol (2014) 72:919–933 The presented experimental results supported Hong and Ding’s initial assumptions and findings. As seen in Fig. 7, new generated chip was restricted by cutting tool’s groove and the contact with backwall of tool, verified by observing cutting tool’s backwall area marked in Fig. 7 in which measured contact length was approximately 0.8 mm and very close to the width of generated chip discussed in Section 3.3. Flow directions of chip (chip flow angle, ηs, is found as 31°) prevented/blocked liquid nitrogen from pene- trating the tool–chip interface, as presented in Fig. 7. Accord- ing to previous published work discussed at the beginning of this section, liquid nitrogen has lubrication aspects ; how- ever, lubrication aspects might not be effectively utilized due to limited penetration of liquid nitrogen to the tool–chip Fig. 8 Yield and ultimate tensile strengths of Inconel 718 forged, solu- interface resulting from the chip flow direction and grooved tion-treated, and aged conditions tool used in the cryogenic machining process. This can be one of the reasons for the deployed second nozzle not contributing increased percentage of yield strength of Inconel 718 and to reduce the force components. increased percentage of force components in two nozzles Although lubrication aspects of cryogenic cooling from assisted cryogenic machining of Inconel 718. Therefore, al- rake face cannot be utilized due to tool geometry and chip tered temperature of work material can be one of the reasons flow, it directly reaches the work material owing to the posi- for increased cutting forces in two nozzles cryogenic machin- tion of nozzle on the rake face and flooding liquid nitrogen ing of Inconel 718. towards the cutting zone. In Fig. 7, it schematically shows the Another significant finding from cryogenic cooling is in- flooded liquid nitrogen from rake face reaching the creased chip thickness as compared with other conditions. unmachined work material. Liquid nitrogen boiling tempera- Friction stress distribution is determined by the mechanical ture is around −196 °C. It is well known that material’s strength, under the deformation conditions of the cutting deformation behavior and thermal properties are influenced experiment, of the chip material adjacent to the rake face of by the temperature changes. the tool and by the distribution over the rake face of the ratio of It should be noted that the thermal properties of Inconel the real to apparent area of contact between chip and tool. 718 such as thermal conductivity, thermal expansion, and Kato et al. also emphasized that stress distribution specific heat considerably change at cryogenic temperature depended not only on the frictional coefficient between tool. In this work, forged, solution-treated, and aged Inconel and chip but also on the deformation characteristics of the 718 were used as work material. According to mechanical test work material, such as strain hardenability. From this perspec- in cryogenic temperatures conducted by Tobler , yield and tive, chip’s material temperature is high in the primary defor- ultimate tensile strength of forged, solution-treated, and aged mation zone during the chip formation, but applying cryogen- Inconel 718 increased approximately 15 and 17 %, respec- ic cooling from the rake face directly reaches and penetrates tively, as shown in Fig. 8. There is a good agreement between into the chip and thus contributing to reducing thermal Fig. 7 Chip flow with effective tool–chip contact region in the cutting tool inserts and the Chip and tool’s LN2 affected area with cryogenic cooling application backwall rake face cooling region contact area Tool’s groove Tool’s groove LN2 affected area ηs with rake face Chip cooling Cutting tool LN2 affected area with flank face cooling Int J Adv Manuf Technol (2014) 72:919–933 925 softening in the secondary deformation area. This quick tran- 1,200 °C at the rake face. Even if such a high temperature sition might result in strain hardening, and hence increased is not reached, the hardness of cemented carbide tool is frictional shear stress. Since friction stress is empirically relat- seriously impacted by the elevated temperature. For instance, ed to shear flow stress of chip material , this can be the hardness of carbide cutting tool decreases more than 50 % another reason for the increased cutting force components at around 800 °C, compared with the hardness of carbide tool with the delivery of liquid nitrogen through two nozzles. at the room temperature. Since high temperatures generat- In summary, it is possible to control the force components ed at the tool’s cutting edge leads to impairment, they are depending on selected cutting speeds and delivering cryogen- subjected to high compressive stresses acting on the tool tip ic cooling. It is found that there is a strong relationship leading to plastic deformation of the tool edge, severe between tool–chip contact length/area and the measured cut- notching, and flank wear. Thus, selection of appropriate ting forces. In cryogenic machining, using cryogenic rake face cooling/lubricating method plays a significant role in control- cooling did not help to reduce cutting forces for selected ling tool wear rate and tool life. conditions and work material; instead, it leads to increasing In machining, the flank wear is the most common criteria all three force components. From the force point of view, because it defines the cutting edge retreat and has a significant delivering liquid nitrogen from two nozzles brings about some influence on the workpiece dimensional accuracy [30–32]. In additional issues if the cutting tool or tool holder is not this experimental study, progressive flank wear with respect to designed appropriately. When the aim is to deliver more liquid the time and crater wear have been observed at 60 m/min nitrogen to the cutting region for more penetration between cutting speed, and also flank and crater wear are observed at tool and chip to show a lubrication effects, chip breaker of the 120 m/min. Progressive flank wear as a function of cooling/ cutting tool must be taken into account. It should be kept in lubrication is shown in Fig. 9. At the beginning, rapid flank mind that if liquid nitrogen cannot be utilized as lubricant due wear at the nose region was observed. It was followed by to the nozzle position, flow rate, chip breaker geometry, tool gradual increases until 150 min and then the tool wear was groove, etc., the delivered liquid nitrogen predominantly accelerated again. reaches the unmachined part of work material causing chang- During the test, no major fracture was observed; however, es in the thermal and mechanical properties of work material, higher buildup edge was observed at approximately the first and, most likely, hardening of material that significantly im- 100 s in dry and cryogenic cooling as compared to MQL. pacts cutting force results. Highest flank wear rate, with respect to time, was measured in dry cutting process. Cryogenic cooling helped to reduce flank 3.2 Tool wear/tool life wear at nose region and increased tool life in machining of Inconel 718. The wear rate of tool used in MQL cutting In order to understand the machining performance of any showed similar performance to the cryogenic condition up to material, tool life/tool wear rate needs to be considered. This 150 s. After that time, the flank wear in MQL condition factor is important for manufacturing cost, machined parts rapidly increased and showed a similar trend with dry ma- dimensional accuracy, and surface quality. In machining chining. Figure 9 shows that the performance of MQL is of aerospace components, the cost of cutting tool may be between dry and cryogenic cooling. negligible as compared to the cost of work materials; however, Although flak wear at the nose region of the tool had a recent research has shown that tool wear has a significant dominant effect on dimensional accuracy and surface quality impact on surface integrity of finish products , a key factor of machined components, this primary factor led to failure or in machining of aerospace components. Therefore, under- reaching the end of the tool life was notch wear in machining standing of tool wear in machining processes may contribute of high-temperature alloys, in particular, Ni-based alloys [28, to the control of the surface integrity and improved product 33]. Figure 10 shows good agreement with the given literature quality. However, it needs to be kept in mind that the tailored that progressive notch wear is more dominant. After 150 s in surface integrity due to tool wear, in turn the product quality, is all three conditions, progressive notch wear exceeded 600 μm usually the results of flank wear at nose. wear limit. Interestingly, while in dry and MQL conditions Cutting tool material played a dominant role on the wear of where there is a steady-state notch wear, cryogenic cooling tool material. Previous studies showed that carbide tools does not show that behavior. Although cryogenic cooling used for machining nickel-based alloys at speeds greater than provides smaller notch wear after 150 s, but the difference 30 m/min failed due to thermal softening of the cobalt binder among all three is negligible. It is possible to conclude that phase and the subsequent plastic deformation of the cutting there was no significant difference among all three conditions edge. This probably occurred because of the relatively from notch wear point of view. low softening point temperature of carbide tool, approximate- Crater wear in different cooling/lubricating conditions does ly 1,100 °C , and considering the poor thermal conductivity not make very significant difference after 150 s cutting time, of Inconel 718 that leads to high cutting temperatures up to as seen from Fig. 11. 926 Int J Adv Manuf Technol (2014) 72:919–933 Fig. 9 Flank wear progression 400 Dry MQL Cryogenic at nose at different cooling/ 375 lubricating conditions 350 (V=60 m/min, f=0.075 mm/rev, 325 ap =0.8 mm) 300 275 VBmax (µm) 250 225 200 175 150 125 100 75 50 25 0 50 100 150 200 250 Time (sec) As mentioned in the previous section, evaluation of mea- than main cutting and feed forces. The finding in this study sured forces with respect to time gives an opportunity for agrees with the literature. Although increases in dry and MQL analysis of the effects of cooling/lubricating on the total power are similar to each other, approximately 150 % in cryogenic consumption during the machining process. Indeed, cutting cooling conditions, increased percentage of radial force was forces are not significantly influenced by tool wear in such approximately 80 %. This percentage is much lower than short cutting time, while in longer cutting time such as the MQL and dry machining. A smaller percentage increase of time required to end the tool life, forces are certainly heavily radial force over the cutting time was attributed to smaller influenced by the wear rate. Figure 12 shows the percentage flank wear at nose. In cryogenic cooling condition, increased increase in force components within 250 s. As expected, main cutting forces among all three conditions were also the forces show an increasing trend as the tool flank and rake smallest. However, in dry conditions, feed force increases surfaces are progressively worn out. were found to be the smallest among all three conditions. Worn flank surface increased the cutting force components Among all three cooling/lubricating conditions, the biggest and particularly the radial force because the tool penetration in variation and increased percentage of force components were the work material became difficult due to the flank wear. found in MQL conditions. As cutting time increased and tool Besides, Bermingham et al. reported that larger tool– wear increased on the rake face, efficiency of lubrication was workpiece engagement area on the minor cutting edge (aux- lost. These observations are likely due to changing tool–chip iliary flank) increases the radial force. Considering the select- interface and contact conditions resulting in increased force ed depth of cut that was equals to nose radius, larger contact and big differences in recorded force components between the area of the flank face primarily in the radial direction due to first 50 and 250 s cutting time. the flank wear mainly affected the variation of radial forces The impact of cooling/lubricating on tool wear (flank and and consequently increases of radial force were much larger crater wear) can be more clearly observed at high speed Fig. 10 Tool notch wear 700 Dry MQL Cryogenic progression at different cooling/ lubricating conditions (V=60 m/ 600 min, f=0.075 mm/rev, ap = 0.8 mm) 500 VNmax (µm) 400 300 200 100 0 50 100 150 200 250 Time (sec) Int J Adv Manuf Technol (2014) 72:919–933 927 Fig. 11 Crater wears as a function of different cooling conditions (V=60 m/min; cutting time, 250 s) Dry MQL Cryo machining of Inconel 718. Indeed, using cryogenic cooling depth of crater wear in cryogenic cooling was the smallest has significant impact on reduction of flank wear as compared among all three conditions, as seen in Fig. 15. to dry and MQL conditions, seen in Fig. 13. The MQL These results demonstrate the importance of controlling condition also made significant contribution to reduced tool cutting temperature through utilization of cooling during ma- wear as compared to dry cutting condition, as shown in chining in efforts to control of tool wear. Utilizing liquid Fig. 13. Increased cutting speed using the selected cutting tool nitrogen for cooling during high speed machining of Inconel under either MQL or cryogenic condition significantly re- 718 also helped to prevent thermal cracks from occurring, duced tool life in comparison with low cutting speed. while in dry and MQL conditions thermal cracks due to The cooling/lubricating effects can also be observed from micro-chipping were observed. It should be noted that al- the result of crater wear measurements shown in Fig. 14. though selected cutting speed (V=120 m/min) seems to sig- While the total area subject to wear at the tool rake face is nificantly reduce tool life, it shows cryogenic cooling efficien- similar in MQL and cryogenic cooling, it was significantly cy clearly. Figure 16 shows the wear mechanism in machining large in dry condition. As the cutting speed increased, reduced of Inconel 718 under the given cutting conditions. In dry tool–chip contact length (Fig. 19) implied that generated high cutting conditions, it is clearly seen that built-up edge forma- temperature on the rake face of cutting tool moved closer to tion occurs on the rake of the tool. the tool tip. Its effect was observed in MQL condition Also, the micro-grooves indicated abrasive wear taking (Fig. 14) where aggressive wear was observed along the tip place. The fracture is closest to the tip of the tool, as shown of the tool. Although catastrophic failure was not observed in in Fig. 16a. In cryogenic cooling conditions, on the rake face, the case of dry machining with increased cutting speed, an micro-grooves are seen as shown in Fig. 16b indicating that extended tool–chip contact was observed over the rake face the abrasive wear mechanism is predominant during the cut- that caused high contact stresses and interface temperature ting process. with the development of larger and deeper crater wear It is well-known fact that when cutting speed increases, the in comparison with MQL and cryogenic cooling as shown in increasing temperature play a vital role on the occurring Fig. 14. plastic deformation, diffusion, abrasion, and resulting progres- In dry machining condition, more localized and deeper sion tool wear. Therefore, it is necessary to carefully crater wear was observed. In the cryogenic and MQL cutting monitor/observe the temperature during the cutting process. conditions, more uniformly distributed wear was observed Since a turning process was conducted in this set of experi- along the contact point (see Fig. 15). It should be noted that ment, it was indeed difficult to measure the cutting tempera- ture. Instead, chip temperature, close to cutting temperature, Main Cutting Radial Feed was measured via infrared camera in all three machining conditions, as shown in Fig. 17. 160 Remarkable temperature difference was recorded among Increased Percentage, % 140 dry, MQL, and cryogenic cooling conditions, as shown in 120 Fig. 18. The overall tool life/tool wear test involved flank 100 wear and crater wear measurements demonstrated cryogenic 80 machining evidently improved performance in reducing tool 60 wear and increasing tool life in machining of Inconel 718 40 under the given parameters compared to dry and MQL con- 20 ditions. MQL also significantly helped to reduce tool wear as 0 compared to dry conditions. It is generally known that MQL is Dry MQL Cryogenic predominantly lubrication rather than cooling and it creates a Fig. 12 Variation of radial force with time (V=60 m/min, f=0.075 mm/ thin film layer between the tool and the chip. This helps to rev, ap =0.8 mm) reduce friction and wear rate. As discussed in the force 928 Int J Adv Manuf Technol (2014) 72:919–933 Fig. 13 Flank wear variation at nose region of cutting tool at different cooling/lubricating condition (V=120 m/min; time, 30 s) Dry (VB = 939 µm) MQL (VB = 340 µm) Cryo (VB = 218 µm) section, particularly depending on cutting tool, chip groove, cutting speed, the diameter of generated helical chip is approx- chip flow direction, and flooded liquid nitrogen, cryogenic imately three times smaller than that generated chips at lower cooling in this set of experiment could not penetrate the speed as seen from Fig. 19. These results are valid in all three intimate tool–chip contact layer, and hence could not be as conditions with some variation. There was a correlation be- effective as needed. One of the biggest advantages of cryo- tween tool–chip contact length over rake face and diameter of genic cooling in machining hard-to-cut materials such as the helical chip in this set of experiment, as illustrated in Inconel 718 is to keep the cutting tool temperature as low as Fig. 19. According to the experimental results, it was found possible to prevent overheating of cutting tool. Figure 18 that the smaller the contact length over the rake face, the smaller shows that compared to other two cutting conditions, cryo- is the diameter of the helical chip. In dry cutting, it did not take genic cooling significantly reduces temperature during ma- place exactly due to extremely high crater wear and high chining process, and thus preventing overheating of the cut- contact area at high speed. Chip morphology and segmentation ting tool. Preventing overheating of cutting tool is very sig- played a predominant role in determining machinability and nificant from the tool wear/tool life perspective in machining tool wear during the machining of hard-to-cut material. of Inconel 718 because the temperature plays a vital role in In machining of some of the hard-to-cut materials like forming most of the wear mechanism, such as diffusion, nickel-based alloys, instabilities in the cutting process occur adhesion, abrasion, thermal crack, etc. The liquid nitrogen as the cutting speed is varied. These instabilities are a for cryogenic cooling is a very powerful tool and it substan- result of thermo-mechanical response of the work material tially assists in reducing tool wear by keeping the cutting under the conditions of cutting. Thermo-plastic instability tool’s temperature low enough to resist against wear. The (strain hardening versus thermal softening) leading to shear measured temperature, flank wear progression, and crater localization results in a serrated chip. The transition speed wears approve the significance of cryogenic cooling on tool at which the chip form changes from a continuous to a shear- wear/tool life in machining of Inconel 718. localized was found to be different for different work materials; for Inconel 718, it was found approximately 61 m/min. 3.3 Chip formation Indeed, the selected cutting speed in this current study was close to the transition speed in the literature. Results obtained In all three conditions (MQL, dry, and cryogenic), observed from this set of experiments showed huge difference among chip breakability was more or less similar with difference cryogenic and MQL and/or dry conditions in terms of chip potentially negligible. These results are related to selected morphology. It is evident that with cryogenic cooling, chip cutting parameters and work material’s properties. In all three thickness profoundly increased compared to dry and MQL con- conditions, obtained chips were long, continuous, and helical ditions, and also segmentation of chip was significantly different type as shown in Fig. 19. However, as a result of increased from the segmented chips generated in dry and MQL conditions. Fig. 14 Crater wear as a function 0.187773 mm2 0.187959 mm2 0.313350 mm2 of different cooling/lubricating (V=120 m/min; cutting time, 30 s) Thermal cracks Thermal cracks MQL Cryogenic Dry Int J Adv Manuf Technol (2014) 72:919–933 929 a LN2 Nozzle Machined Surface Dry Insert Chip Measured Max. Temperature MQL b Chip Measured Max. Temperature Cryo Fig. 15 3-D image of crater wear (V=120 m/min) Figure 20 shows that in cryogenic cooling, chip thickness (t2) increased approximately 30 % as compared to MQL conditions. Dry cutting generated the smallest chip thickness in this set of c experiments. It is evident that in all three conditions, segmented Chip chips were generated. Pitch of the chip generated in MQL Measured Max. condition is smaller than pitch of chip generated in dry and Temperature cryogenic conditions. Cryogenic cooling generates the pitch, which is approximately three times larger than pitch generated in dry and MQL conditions. Furthermore, the valley in cryogenic was smaller than those in dry and MQL. The variation of chip thickness as a function cooling/lubrication might be attributed to shear angle that can be influenced from cutting temperature. In cryogenic cooling conditions, generated higher cutting forces discussed in Section 3.1 can also be a result of thicker chip Fig. 17 Measured highest temperature on chip with infrared camera in a thickness owing to reduced shear angle in the shear plane. cryogenic; b dry; c MQL (V=60 m/min) However, more investigation into including measuring and/or predicting shear angle is necessary to correlate the obtained chip main factors in driving force to chip morphology and chip thickness and force components. In some previous work, the serration [31, 39, 40], and found that very high cutting speed, cutting speed and hardness of work material were considered due to the effect of thermal softening, generated shear-localized Fig. 16 a Rake face of tool used a b in dry cutting; b flank face of tool used in cryogenic machining (V= Micro grooves 120 m/min) Chip flow direction Fracture Micro grooves Built-up-edge 930 Int J Adv Manuf Technol (2014) 72:919–933 900 Dry Measured Max. Temperature 800 700 600 500 t2=115 µm (°C) 400 300 200 100 MQL 0 Dry MQL Cryo Fig. 18 Measured maximum temperature on the generated chip as a function of cooling/lubricating t2=121 µm chip with segments almost completely detached. It should be noted that they did not take cooling/lubricating into account in their study. In cryogenic cooling where the yield points of work mate- Cryo rial increased approximately 15 % before it was deformed and the deformation process in the shear plane, the temperature of material quickly yielded a large temperature difference be- t2=160 µm tween the undeformed chip and generated chips in comparison with dry and MQL conditions. Results obtained from this experimental work should be comprehensively studied to determine if the well-known chip formation/morphology criteria, namely thermo-mechanical shear instability, is valid Fig. 20 Obtained chip morphology as a function of cooling/lubricating in cryogenic cooling conditions. in machining of Inconel 718 (V=60 m/min) 3.4 Surface quality of machined workpiece components and to determine if expected requirements are met through selected cutting and cooling/lubricating condi- As one of the most important machining performance ele- tions. Although developed models and optimum surface qual- ments in metal cutting industry, surface roughness/quality ity criteria can work well for most materials and cutting should to be investigated to measure the quality of machined conditions , the work material, such as Inconel 718, and the conditions, such as cryogenic cooling, require additional investigation of surface roughness. The previously developed empirical model or optimum conditions may not be valid in cases where cutting material has high strain hardening and poor thermal conductivity. Also, it may not be valid under cryogenic cooling condition that significantly changes the cutting temperature of machining process as compared to other common cooling/lubricating methods widely used in manufacturing industries (flood cooling, MQL, dry machin- ing). Machined surface measurements are shown in Fig. 21, illustrating both MQL and cryogenic cooling produce better surface quality in comparison with dry machining. Figure 21 indicates that differences are attributed to the surface topography. In particular, in dry machining conditions some surface defects were observed, which might be the result of built-up edge and have been observed to increase surface roughness and deteriorate surface topography as compared Fig. 19 Chip formation and tool–chip contact length resulting from the with MQL and cryogenic cooling conditions. Otherwise, it variation of cutting speed (cooling conditions, cryogenic cooling) does generate more or less similar quality with MQL and Int J Adv Manuf Technol (2014) 72:919–933 931 a b c Ra= 0.641µm Ra= 0.318 µm Ra= 0.325 µm Fig. 21 Surface topography and roughness of machined components a in dry; b MQL; and c cryogenic conditions (V=60 m/min) cryogenic. It should be noted that a small difference in cutting in comparison with dry and cryogenic at low speed. At high parameters made a considerable difference in the surface speed, cryogenic cooling generated the least forces among all topography of Inconel 718; however, the measured surface three conditions. Increased percentage of force components roughness showed a similar behavior at low speed machining over 250 s cutting time was the highest in MQL, while the of Inconel 718. smallest percentage increases were recorded with cryogenic The machined work material’s surface displayed feed machining in terms of the radial force and main cutting force marks that were less visible at high speed, as shown in components. Hence, it is demonstrated that force components Fig. 22. The machined surface in all three conditions seemed are induced from progressive tool wear for this specific con- deteriorated, although measured surface roughness values did ditions and parameters. In cryogenic cooling, two nozzles did not notably change. With increased speed, surface quality of not provide any advantages in reducing force requirements; dry machining became better. Deteriorated surface morphol- instead, it leads to increased force requirement. Cutting tools ogy and feed marks were attributed to generated high cutting and geometries, in particular chip grooves, had a considerable temperature at higher cutting speeds compared with lower impact on preventing/limiting penetrating liquid nitrogen in cutting speeds, although the average surface roughness was tool–chip contact layer, and had impact in reducing the effi- not significantly changed. Additionally, the flank wear at the ciency of cryogenic cooling in machining performance. nose region at higher cutting speeds compared to lower cutting Therefore, these factors need to be considered in cryogenic speed was much bigger and this deformed the peaks of feed machining. There was a good correlation between tool–chip marks and deteriorated the geometry of feed marks, thus contact length and recorded force components. Increased resulting in more flattened surface. These results were valid speed in MQL and cryogenic conditions reduced tool–chip in all three conditions. contact length as compared to dry cutting. Cryogenic machining within the selected conditions was a better option to reduce progression of flank wear and crater 4 Summary and conclusions wear rate. Different cooling/lubricating did not help in reduc- ing the progressive notch wear; it plays a governing role in This work demonstrated the cryogenic machining of Inconel determining tool life. 718, and compared its performance to dry and MQL condi- Selected cutting parameters and cooling/lubricating condi- tions. The following conclusions can be drawn from machin- tions did not improve chip breakability, while increased cut- ing of Inconel 718 high-temperature aerospace alloys with the ting speed profoundly reduced helical chip diameter. Cryo- given cutting conditions and parameters. genic cooling generated thicker chips that were segmented Machining performance of Inconel 718 in turning process- and had bigger pitch than chips generated in dry and MQL es can be improved by using cooling and lubricating. Cryo- conditions. genic cooling and MQL in general demonstrated contribution Surface quality was improved by using cooling/lubricating. in improving performance if used with suitable cutting param- At higher cutting speeds, cryogenic machining generated the eters. MQL significantly reduced all three force components best surface quality attributed to less tool wear and less a b c Ra= 0.441 µm Ra= 0.413 µm Ra= 0.336 µm Fig. 22 Surface topography and roughness of machined components a in dry; b MQL; and c cryogenic (V=120 m/min) 932 Int J Adv Manuf Technol (2014) 72:919–933 thermal distortion. At lower cutting speed, some surface de- 15. Hong SY, Markus I, Jeong W (2001) New cooling approach and tool life improvement in cryogenic machining of titanium alloy Ti-6Al- fects on the surface of dry machined sample were observed. 4V. Int J Mach Tool Manuf 41(15):2245–2260 Considering the obtained results, using cryogenic cooling 16. Ee KC, Balaji AK, Jawahir IS (2003) Progressive tool-wear mecha- at higher cutting speed in machining of Inconel 718 is highly nisms and their effects on chip-curl/chip-form in machining with recommended; however, using MQL machining at lower cut- grooved tools: an extended application of the equivalent toolface (ET) model. Wear 255:1404–1413 ting speed is recommended to improve machining perfor- 17. Ee KC, Balaji AK, Li PX, Jawahir IS (2001) Force decomposition mance of this particular alloy. model for tool-wear in turning with grooved cutting tools. Wear 249(10–11):985–994 18. Jawahir IS, Ghosh R, Fang XD, Li PX (1995) An investigation of the Acknowledgments The author gratefully acknowledges Professor I.S. effects of chip flow on tool-wear in machining with complex grooved Jawahir for reviewing the draft of this paper and for his very valuable tools. Wear 184(2):145–154 comments. Author thanks the Institute for Sustainable Manufacturing 19. Hong SY, Ding YH, Jeong W (2001) Friction and cutting forces in (ISM) for providing all required equipment for conducting this study. cryogenic machining of Ti-6Al-4V. Int J Mach Tool Manuf 41(15): 2271–2285 20. Hong SY, Ding Y (2001) Micro-temperature manipulation in cryo- genic machining of low carbon steel. J Mater Proc Tech 116(1):22–30 References 21. Marquardt ED, Le JP, Radebaugh R (2000) Cryogenic material properties database. 11th International Cryocooler Conference, pp. 681–687 1. Ezugwu EO (2005) Key improvements in the machining of difficult- 22. Tobler RL (1976) Low temperature effects on the fracture behaviour to-cut aerospace superalloys. Int J Mach Tool Manuf 45(12–13): of a nickel base superalloy. Cryogenics 16(11):669–674 1353–1367 23. Childs THC, Wilcox AB, Richings D (1972) Metal-cutting- 2. Ezugwu EO, Bonney J, Yamane Y (2003) An overview of the mechanics, surface physics and metallurgy. Int J Mech Sci 14(6):359 machinability of aeroengine alloys. J Mater Proc Tech 134(2):233– 24. Kato S, Yamaguch K, Yamada M (1972) Stress distribution at inter- 253 face between tool and chip in machining. J Eng Ind 94(2):683 3. Hong SY, Zhao Z (1999) Thermal aspects, material considerations 25. Childs T, Maekawa K (1990) Computer-aided simulation and exper- and cooling strategies in cryogenic machining. Clean Prod Proc 1: imental studies of chip flow and tool wear in the turning of low alloy 107–116 steels by cemented carbide tools. Wear 139(2):235–250 4. Wanigarathne PC, Liew J, Wang X, Dillon OW, Jawahir IS (2004) 26. Guo YB, Li W, Jawahir IS (2009) Surface integrity characterization Assessment of process sustainability for product manufacture in and prediction in machining of hardened and difficult-to-machine machining operations. Global Conference on Sustainable Product alloys: a state-of-art research review and analysis. Mach Sci Technol Development and Life Cycle Engineering 305–312 13(4):437–470 5. Shokrani A, Dhokia V, Newman ST (2012) Environmentally con- 27. Bouzakis KD, Michailidis N, Vidakis N, Eftathiou K, Kompogiannis scious machining of difficult-to-machine materials with regard to S, Erkens G (2000) Interpretation of PVD coated inserts wear phe- cutting fluids. Int J Mach Tools Manuf 57:83–101 nomena in turning. CIRP Ann-Manuf Technol 49(1):65–68 6. Shokrani A, Dhokia V, Munoz-Escalona P, Newman S (2013) State- 28. Ezugwu EO, Wang ZM, Machado AR (1999) The machinability of of-the-art cryogenic machining and processing. Int J Computer nickel-based alloys: a review. J Mater Process Technol 86(1–3):1–16 Integrated Manufacturing 26(7):616-648 29. Ezugwu EO, Bonney J (2004) Effect of high-pressure coolant supply 7. Kamata Y, Obikawa T (2007) High speed MQL finish-turning of when machining nickel-base, Inconel 718, alloy with coated carbide Inconel 718 with different coated tools. J Mater Proc Tech 192:281– tools. J Mater Process Technol 153:1045–1050 286 30. Poulachon G, Bandyopadhyay BP, Jawahir IS, Pheulpin S, Seguin E 8. Pusavec F, Hamdi H, Kopac J, Jawahir IS (2011) Surface integrity in (2004) Wear behavior of CBN tools while turning various hardened cryogenic machining of nickel based alloy-Inconel 718. J Mater Proc steels. Wear 256(3–4):302–310 Tech 211(4):773–783. doi:10.1016/j.jmatprotec.2010.12.013 31. Poulachon G, Moisan A, Jawahir IS (2001) Tool-wear mechanisms in 9. Obikawa T, Kamata Y, Asano Y, Nakayama K, Otieno AW (2008) hard turning with polycrystalline cubic boron nitride tools. Wear 250: Micro-liter lubrication machining of Inconel 718. Int J Mach Tool 576–586 Manuf 48(15):1605–1612 32. Astakhov VP (2004) The assessment of cutting tool wear. Int J Mach 10. Obikawa T, Yamaguchi M, Funai K, Kamata YSY (2012) Air jet Tool Manuf 44(6):637–647 assisted machining of nickel-base superalloy. Int J Mach Tool Manuf 33. Choudhury I, El-Baradie M (1998) Machinability of nickel-base 61:20–26 super alloys: a general review. J Mater Process Technol 77(1):278– 11. Pusavec F, Deshpande A, M’Saoubi R, Kopac J, Dillon OW, Jawahir 284 IS (2008) Modeling and optimization of machining of high temper- 34. Wanigarathne PC, Kardekar AD, Dillon OW, Poulachon G, Jawahir ature nickel alloy for improved machining performance and enhanced IS (2005) Progressive tool-wear in machining with coated grooved sustainability. Proceedings of the 11th CIRP conference on Modeling tools and its correlation with cutting temperature. Wear 259:1215– of Machining Operations, pp. 21–28 1224 12. Williams J, Tabor D (1977) The role of lubricants in machining. Wear 35. Bermingham M, Kirsch J, Sun S, Palanisamy S, Dargusch M (2011) 43(3):275–292 New observations on tool life, cutting forces and chip morphology in 13. Dudzinski D, Devillez A, Moufki A, Larrouquere D, Zerrouki V, cryogenic machining Ti-6Al-4V. Int J Mach Tools Manuf 51(6):500– Vigneau J (2004) A review of developments towards dry and high 511 speed machining of Inconel 718 alloy. Int J Mach Tool Manuf 44(4): 36. Stephenson DA, Agapiou JS (1997) Metal cutting theory and prac- 439–456 tice. Marcel Dekker, New York, pp 577–611 14. Hong SY, Ding YC (2001) Cooling approaches and cutting temper- 37. Hua J, Shivpuri R (2004) Prediction of chip morphology and seg- atures in cryogenic machining of Ti-6Al-4V. Int J Mach Tool Manuf mentation during the machining of titanium alloys. J Mater Proc Tech 41(10):1417–1437 150(1–2):124–133 Int J Adv Manuf Technol (2014) 72:919–933 933 38. Hou ZB, Komanduri R (1997) Modeling of thermomechanical shear 42. Wang Z, Rajurkar K, Fan J, Lei S, Shin Y, Petrescu G (2003) Hybrid instability in machining. Int J Mech Sci 39(11):1273–1314 machining of Inconel 718. Int J Mach Tools Manuf 43(13):1391–1396 39. Komanduri R, Schroeder T, Hazra J, Vonturkovich BF, Flom DG 43. Pusavec F, Kramar D, Krajnik P, Kopac J (2010) Transitioning to (1982) On the catastrophic shear instability in high-speed machining sustainable production—part II: evaluation of sustainable machining of an aisi 4340 steel. J Eng Ind-T Asme 104(2):121–131 technologies. J Clean Prod 18(12):1211–1221 40. Poulachon G, Moisan A, Jawahir IS (2001) On modelling the influ- 44. Kenda J, Pusavec F, Kopac J (2011) Analysis of residual stresses in ence of thermo-mechanical behavior in chip formation during hard sustainable cryogenic machining of nickel based alloy—Inconel 718. turning of 100Cr6 bearing steel. CIRPAnn-Manuf Technol 50(1):31– J Manuf Sci Eng 133:041009–041007 36 45. Feyzi T, Safavi SM (2013) Improving machinability of Inconel 718 41. Wang ZY, Rajurkar KP (2000) Cryogenic machining of hard-to-cut with a new hybrid machining technique. Int J Adv Manuf Technol materials. Wear 239(2):168–175 66(5–8):1025–1030