Protein Secondary Structure PDF
Document Details
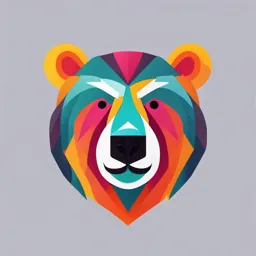
Uploaded by YouthfulGothicArt
Tags
Summary
This document provides a detailed explanation of protein secondary structure, focusing on alpha-helices and beta-sheets, with an emphasis on the factors that dictate their formation and stability. Dihedral angles and Ramachandran plots are used to illustrate the allowed backbone conformations. Examples of different protein structures incorporating these elements are used to reinforce the concepts.
Full Transcript
 Protein secondary structure   #### Secondary structure Leninger Fig 3-13 - Secondary structure reflects the arrangement of residues close in sequence - Most residues in most proteins can be seen to be forming either α...
 Protein secondary structure   #### Secondary structure Leninger Fig 3-13 - Secondary structure reflects the arrangement of residues close in sequence - Most residues in most proteins can be seen to be forming either α-helices or β-strands - But what dictates that these arrangements predominate, and not others? Protein backbone conformation ================================================== - The conformation of the backbone can be understood by examining the bonds that join one residue to the next - Three bonds join each Cα to the next Cα, so a protein's conformation can be described by three angles per residue - These angles are named omega (Ω), phi (Φ), and psi (Ψ) Dihedral angles --definition =================================================  - Given four atoms A-B-C-D connected in a row - The dihedral angle is the angle made between the A-B bond and the C-D bond when looking down the B-C bond - Measured in a clockwise direction - Single bonds are short (\ - The omega angle is the dihedral angle between C of the previous residue and nitrogen of this residue - It is measured clockwise from C~α(-1)~ to C~α~ 3.8 Å #### character - In amide bonds, the C-N bond is **resonance stabilized** - The partial double bond character of the C-N bond imposes a high energetic penalty for distorting the Cα-C-N- Cα dihedral (the Ω angle) away from 180° (or 0°) - In small peptides, Ω is always very close to 180° - Note that this means that the groups joining Cα to Cα are rigid, holding these atoms 3.8 Å apart  - Very precise high-resolution structures show that ω is often slightly twisted (5 to 10˚) from the ideal 180˚ - This distortion causes local strain, but better packing interactions elsewhere within the protein can compensate *cis* (Ω = 0˚) peptide bonds are much less stable than *trans* (Ω = 180˚) -------------------------------------------------------------------------  - For Xaa~1~-Xaa~2~ (where Xaa~2~ is [not] Proline), *cis* peptide bonds are around 20 kJ/mol [less] stable than the corresponding *trans* peptide bond - Consequently, only 0.03% (\~1 in 3000) of amino acids (proline not in the second position) are in the *cis* configuration - Given that the average protein has \~375 a.a., one in tent proteins ### Proline more readily adopts *cis*   - For Xaa-Pro, the Cδ atom clashes [in *trans* conformation] - Therefore, *cis* is only 2 kJ/mol less stable than trans - About 5.4% (\~1 in 18) of Xaa-Pro peptides are *cis* - *cis-*Prolines have important structural roles, e.g. in forming certain types of turns  ### Phi & Psi define backbone conformation - Generally, we can assume peptide bonds are essentially flat, with Ω - Cα(-1), C(-1), O(-1), NH and Cα (\*) [are confined to one plane] - Backbone conformation is then a function of two dihedral angles per residue: phi (φ) and psi (ψ) - I.e. knowing just phi and psi angles for every amino acid is enough to describe the overall structure of a protein (side chains excluded) - Phi (Greek letter φ) is the dihedral angle subtended by the N-Cα bond - It measures the angle between carbonyl carbon (CO) groups in a clockwise fashion - Possible mnemonic: CO-Phi (coffee) - Psi (Greek letter Cα -CO bond - It measures the angle between NH groups in a clockwise fashion - Possible mnemonic: PoSeIdoN (and hey, is that not a trident?) Ramachandran plots ========================================= - Ramachandran plots show the stability of an amino acid in a protein as a function of [Phi] and [Psi] angle - The green areas correspond to conformations where strain and van der Waals clashing is minimal - Note that positive phi values are largely disallowed because the carbonyl oxygen groups tend to clash (on right with C~β~) #### Ramachandran describe allowed backbone conformations - Amino acids need to avoid strongly strained conformations - However, they also need to maximize stabilizing interactions (e.g. H-bonds) - Actual observed conformations (black dots) are a compromise - Typically, \< 2 % of amino acids will be outside the blue areas - Ramachandran plots differ slightly for different amino acids - E.g. Val, Ile and Thr are slightly more restricted by the C~β~ branching Lehninger, 6e 4.9b Ramachandran plot for Proline ==================================================  - The imine ring of proline restricts Phi to values of - Proline is much less flexible than other amino acids Ramachandran plot for Glycine ==================================================== - Glycine (H- sidechain) has no hand and therefore no preference for L- or R- conformations - Glycine is therefore can adopt conformations that would be very strained for any other amino acid  The extended state ----------------------------------------- - If φ = -ψ, this results in a residue with an extended conformation - φ = -135; ψ = 135 is extended and minimally strained - In this maximally extended state, **peptides span 3.5 Å per residue** - Attaining the minimal energy state of the backbone requires minimizing conformational strain, while maximizing interactions - Protein interiors are generally non-polar, so buried backbone amide groups generally hydrogen bond with other backbone amides - Hydrogen bonds are much stronger with optimal geometry, which strongly constrains favourable conformations - The fixed omega angle limits the flexibility of the polypeptide chain - It is only possible for multiple successive buried peptide groups to make good interactions if all adopt a similar pattern of repeated interactions - These **runs of residues in (near) identical conformations, stabilized by repeated patterns of hydrogen bonds,** are the **secondary structure elements** that are characteristic of protein structure #### Favoured regions of the Ramachandran plot are characteristic of specific secondary structures - We can distinguish three general regions of the Ramachandran plot that are unstrained - α (characteristic of α−helices) - β (characteristic of β−strands) - L: left-handed α-helix, infrequent and never forms extended runs Lehninger, 6e 4.9a  α-helix ======= - Helices are characterized by a repeating pattern of hydrogen bonds between residues a small set number apart in sequence - In α-helices, the carbonyl oxygen of **each residue H-bonds with the NH of the residue 4 residues C-terminal** to it (e.g. 1 with 5) - 3.6 residues per turn (=100˚ rotation per a.a.) - The α-helix length increases by 1.5 Å for each - Residues (C~α~-C~β~ bond) point towards N- terminus (so side chains "hang down" like a Christmas tree) - There are 3.6 residues per turn - There are 13 atoms that covalently link the two H- bonded atoms (including the hydrogen atom) - The α-helix is therefore also called a 3.6~13~ helix α-Helix Phi - Psi angles =============================================== - α-helices occur with φ around - Note that the backbone conformation is also unstrained with more negative - This is because despite the lack of clashes, hydrogen bonding is compromised - α-helices are very common, accounting for about one third of a.a. in protein structures  The α- helix is compact and stable ---------------------------------- - The α-helix is tightly packed - Residues on the inside being in van der Waals contacts with each other (note - no hole in the middle) - The α-helix is therefore stabilized by: - tight packing - strong hydrogen bonds between turns - a lack of backbone strain (Ramachandran) α-helices are right-handed =============================================== - Thumb points along the axis - Fingers curl around and "up" the helix -  α-helices, 3~10~ and pi helices are all right- handed Left-handed helices are very rare ================================= - In principle, it is possible to have a run of residues in "L" conformation - These then form a left-handed helix - Such helices are very rare in real proteins - Since the "L" conformation is only marginally stable, the surrounding protein is needed to stabilize such regions - Such helices are very rare (\ - The CαH in β-sheets forms a weak H- bond with the carbonyl oxygen of the opposite strand (orange dash line) - Changes in the atom's chemical shift (measured by NMR) show that this interaction is a hydrogen bond - Crystallography confirms that the hydrogen atom is shifted towards the carbonyl oxygen - These hydrogen bonds help stabilize both parallel and anti-parallel β-sheets β-strand types prefer to not mix ================================ - Parallel and antiparallel β-sheets have slightly different backbone geometry preferences - The geometric compromises required to mix parallel and antiparallel strands in the same sheet creates a small energetic cost - As a result, only about 20% of strands have parallel interactions on one side, and anti-parallel on the other #### Parallel β-strands connect almost exclusively through right-handed connections - This applies whether there is a loop, an α-helix or a whole sub-domain between the strands C N - Rossmann fold domain Polyproline II helices =========================================== - Polyproline II helices are right-handed, with three residues per turn - They make no internal hydrogen bonds - They are almost as extended as β-strands - Generally, the preferred conformation of extended proline tracts - Prolines favour this structure, but it can also occur in sequences with no proline residues - Comprise about 2 % of protein residues - Polyproline I helices are all-*cis* and left-handed -- and even rarer PPII helix as a unique ligand ========================================================================= - Four copies of the acetylcholine esterase oligomerization domain (white) assemble around a single copy of a poly-proline membrane anchor protein (blue) - The anchor protein is proline rich and forms a PPII helix - This complex is important for recruiting acetylcholine esterase to the membrane PPII bundles ============ - Snow flea ice binding protein uses an extended flat surface to bind ice - The structure is built from six co-linear polyproline II helices - The structure is stabilized by hydrogen bonds between poly-Pro II helices that extend out at 120˚ intervals - All interior amino acids are glycine to allow chains to pack closely on all sides - There is no hydrophobic core in this protein - Very few proteins are built this way - Turns are short motifs that allow the protein chain to do a quick 180˚ turn - These very commonly connect adjacent anti-parallel β-strands - β-turns are comprised of four residues and are held together by a main chain hydrogen bond - Type II turns require that the residue in position 3 be in L (left- handed helical conformation) - for this reason, it is generally a glycine Loops =====  - Successive secondary structural elements (helices and strands) are connected by loops - Loops are irregular and can adopt almost any conformation as that is stabilized by available local interactions - Loop residues do need to adopt conformations from [favoured areas of the Ramachandran plot] - Backbone is a mix of α-like and β-like conformations, with no regular order - Loops range from short (e.g. 1 residue) to tens of residues - Loops rarely pack between secondary structural elements - Generally, they are on the surface of the [protein] ### Loops are stabilized by interactions with the rest of the structure - Loops do not have a regular inbuilt set of H-bond interactions - They make H-bonds wherever they can - with other main chain atoms, side chains - Many residues hydrogen bond with water -- hence the preference for surface locations - The lack of prior constraints allows enormous conformational diversity - This is critical -- loops are often the "business end" of a protein - Proteins average \~30 % of residues  - As with the main chain, side chains prefer to adopt conformations that minimize internal steric clashes - Consequently, residues prefer a set of specific conformations known as rotamers - Most non-polar residues have few single bonds (e.g. Leu), and can adopt only a few rotamers - Long hydrophilic residues (e.g. Arg) can adopt many more conformations, but still avoid strain Different amino acids preferentially stabilize different secondary structure ------------------------------------------------------------------------------------------------- - Side chains in α-helices pack relatively closely - This favours amino acids that are linear - MALEK (Met Ala etc.) residues most strongly favour α-helices - Hydrophobic interactions are the primary factor stabilizing β- sheets, so large nonpolar amino acids have the largest sheet- forming propensities - β-branched amino acids (V,I,T) also favour β-strands - Asp, Asn and Ser can form local hydrogen bonds with the main chain, and are preferred at the N-terminus of α-helices and C- terminus of β-strands - Pro breaks the hydrogen bonding pattern, and favours loops - Gly is very flexible and has no methyl group to pack, and favours loops too  Predicting secondary structure ============================== - Chou & Fasman (1970) came up with a simple algorithm for predicting the secondary structure of a protein given the amino acid sequence - They noticed from surveys of available structures that some secondary structure elements are enriched in certain amino acids. - By counting the secondary structural context of each amino acid in all known structures, they generated a table of trends - They found that you could (fairly) reliably predict the secondary structural of new proteins by looking at the s.s. preferences of short (5+) runs of amino acids in the sequence Chou Fasman a.a. preference table ------------------------------------------------------ - P(α) is the preference for that amino acid to form and - P(β) - β-strand preference - P(turn) - preference to be in a turn - A score \ 100 indicates that a residue favours that conformation - Thus P(α) \ 100 are helix called formers e.g. glutamate - Residues with P(α) \ - Using a multiple sequence alignment provides a great deal more information than a single sequence for prediction algorithms - [Secondary structure tends to be more conserved than the] [sequence] - Insertions and deletions generally do not occur in the middle of secondary structural elements (what are two "extra" residues going to do in the middle of a helix?) but rather in loops - The predictions from all sequences can be pooled and averaged to increase confidence - E.g. an amino acid that is generally helix breaking can more confidently be added to a helix if the residue is generally helix promoting in homologous sequences Consensus predictions ===================== - Using multiple tools for predicting secondary structure and then using the consensus gives the most robust results - Jpred will generate a multiple sequence alignment, run several independent secondary structure prediction programs for you and give a consensus secondary structure prediction - [[http://www.compbio.dundee.ac.uk/\~www-jpred/submit.html]](http://www.compbio.dundee.ac.uk/~www-jpred/submit.html) - In general, the agreement between jpred consensus and the real s.s. pretty good (often \\> 75%) - The exact beginning/end of s.s. elements are often off by 1-2 a.a. - Some elements are missed entirely e.g. last helix - Methods that consider local sequence can give good, but not perfect predictions - Recent methods that predict the whole structure (e.g. Alphafold) also predict secondary structure - These predictions are more accurate than secondary structure alone predictions (\~98% accuracy)