Lesson: Secondary Structure PDF
Document Details
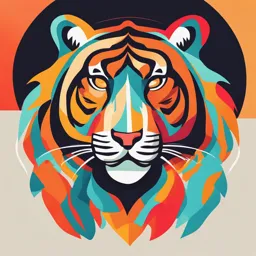
Uploaded by AdventuresomeSatyr
Tags
Summary
This document details secondary structure in proteins, explaining alpha-helices, beta-sheets, and beta-turns, and the role of hydrogen bonds. It also provides details on the conformational preferences of amino acids in these structures and how to analyze relevant diagrams.
Full Transcript
Lesson: Secondary structure → By [email protected] This lesson is designed to prepare you to talk about secondary structure in class. At the end of this lesson, you need to be able to... - illustrate the role of hydrogen bonds between main-chain atoms in secondary structure...
Lesson: Secondary structure → By [email protected] This lesson is designed to prepare you to talk about secondary structure in class. At the end of this lesson, you need to be able to... - illustrate the role of hydrogen bonds between main-chain atoms in secondary structure elements - describe and analyse the structure and properties of α-helices, β-sheets, and β-turns - recognize how secondary structure is defined by the permitted ϕ and ψ angles of the amino acids within the segment and how the Ramachandran plot visually depicts this - explain and apply the conformational preferences of amino acids within secondary structure elements Secondary structure Here, we will explore protein secondary structure. Secondary structure is the local spatial arrangement of the polypeptide’s backbone atoms ignoring the conformations of its side chains. It includes the stable and widely occurring α-helices, β-sheets, and β-turns. Hydrogen bonds Hydrogen bonds are crucial to α-helices and β-sheets. Hydrogen atoms on electronegative atoms, e.g. N-H or O-H, are sufficiently electron poor that they will interact with lone pairs usually on N or O atoms (see figure). This is because the bonding pair is pulled towards the nitrogen or oxygen atom, which leaves that end of the bond partially negative (δ-) and the hydrogen end partially positive (δ+). The group that provides the hydrogen atom is referred to as the hydrogen bond donor; the group that provides the lone pair is referred to as the hydrogen bond acceptor. Hydrogen bonds are much weaker than covalent bonds, yet they are strong enough - when many form simultaneously - to help stabilize specific structures! They have a bond dissociation energy (i.e. the energy required to break the bond) between 2 to 40 kJ/mol, compared to ~350 kJ/mol for the covalent C-C bond. Hydrogen bonds are also longer than covalent bonds; their bond distances range from ~ 2 Å, compared to ~ 1 Å for a single covalent bond. The strongest hydrogen bonds have the hydrogen-bond donor, the hydrogen atom, and the hydrogen-bond acceptor along a straight line. Help: Covalent and noncovalent bonds Refresh your knowledge on covalent and noncovalent bonds, like the hydrogen bond, (seen in A- level chemistry and/or university chemistry modules), see: Lehninger Principles of Biochemistry; David L. Nelson, Michael M. Cox 2.1 Weak Interactions in Aqueous Systems (but not “Osmotic Pressure”) Biochemistry; Jeremy M. Berg, Gregory J. Gatto Jr., Justin K. Jines, John L. Tymoczko, Lubert Stryer 1.3 Concepts from Chemistry Explain the Properties of Biological Molecules: Covalent bonds and noncovalent interactions are important for structure and stability of biological molecules; and The formation of DNA’s double helix is an expression of the rules of chemistry Both are available through Kortext using your [email protected] and password. α-helix The α-helix was first described by Linus Pauling, Robert Corey, and Herman Branson. It is stabilised by hydrogen bonds between C=O and N-H groups that are four residues along, i.e. x and x+4 (see figure, stick side view, green and yellow). Except for amino acids near the ends of an α- helix, all the main-chain C=O and N-H groups are hydrogen bonded. The side-chains project outwards from the α-helix; thus, steric interference with the backbone or with other sidechains is avoided (see figure, stick side and top views, purple). Each residue is related to the next one by a rise of 1.5 Å along the axis and a rotation of 100 degrees, which gives 3.6 amino acid residues per turn (see figure, stick and cartoon side views, red). Thus, amino acids spaced three and four apart in the sequence, i.e. x and x+3 and x+4, are spatially quite close to one another in an α-helix. The pitch of the α-helix, which is the distance for one complete turn, is equal to the product of the rise between two amino acids (i.e. 1.5 Å) and the number of residues per turn (i.e. 3.6), is 5.4 Å (see figure, stick and cartoon side views, red). The screw sense of an α-helix can be right-handed (rotation is clockwise as the polypeptide chain is followed N to C) or left-handed (counter clockwise) (see figure, stick top view, light blue). Yet essentially all α-helices found in proteins are right-handed. A small electric dipole exists in each peptide bond (see earlier). These dipoles are connected through the hydrogen bonds of the helix, resulting in an overall helix dipole, extending along the helix that increases with helix length (see figure, cartoon side view, orange). β-sheet Another common secondary structure is the β-sheet, which contains extended stretches of polypeptide chain with hydrogen bonds between neighbouring strands between C=O and N-H groups. In a parallel β-sheet, polypeptide strands run in the same direction (i.e. from N- to C- terminus) (see figure 1), whereas in an antiparallel β-sheet, neighbouring strands extend in opposite directions (see figure 2). In the parallel arrangement, for each amino acid, the N-H group is hydrogen bonded to the C=O group of one amino acid on the adjacent strand, whereas the C=O group is hydrogen bonded to the N-H group on the amino acid two residues along the chain (see figure 1). In the antiparallel arrangement, the N-H group and the C=O group of each amino acid are respectively hydrogen bonded to the C=O group and the N-H group of a partner on the adjacent chain (see figure 2). The strand in a β-sheet is almost fully extended. The distance between adjacent amino acids along a strand is approximately 3.5 Å (in contrast with a distance of 1.5 Å along an α-helix). The side chains of adjacent amino acids point in opposite direction. Many strands, typically 4 or 5, but more are possible, can come together in β-sheets. The β-sheets can be purely antiparallel, purely parallel, or mixed. Loops and turns Loops and turns connect α-helices and β-sheets and can cause a change in direction of the polypeptide’s chain. Loops can vary in lengths and shapes. They are generally located on the surface of the protein and are rich in polar amino acids. In general, α-helices and β-sheets are evolutionary more conserved than loops. Small loops with only 3 to 6 amino acids, a regular hydrogen bond pattern, and specific dihedral angles are called turns. There are many classes of turns, the most common one is the β-turn. A β-turn (also known as a reverse turn) is a 180° turn involving four amino acid residues. The C=O group of residue x is hydrogen bonded to the N-H group of residue x + 3. This interaction stabilizes abrupt changes in direction of the polypeptide chain (see figure). β-turns are further divided into classes based on the φ and ψ angles of the residues at positions x + 1 and x + 2 (see figure). Types I and II are the most common. Type I turns have approximately φx+1 = - 60°, ψx+1 = -30°, φx+2 = -90°, ψx+2 = 0°, and type II approximately φ2 = -60°, ψ2 = 120°, φ3 = 80°, ψ3 = 0°. The x + 2 residue of the type II turn can only be occupied by glycine. Ramachandran plot and secondary structures All secondary structure elements fall within the ‘allowed’ conformation areas of the Ramachandran plot (see earlier). The different secondary structures can be distinguished by their range of φ and ψ values with the values of different secondary structures mapping to different regions of the Ramachandran plot (see figure). Secondary structure propensity scores and proline Certain amino acids are observed more frequently than others in α-helices, β-sheets or β-turns, reflecting the properties of the R-group and the φ and ψ angles it can adopt. Chou and Fasman investigated this phenomenon in 1974. They calculated the propensity of each amino acid to participate in α-helices, β-sheets or β-turns, i.e. P(α), P(β), and P(turn), based on observed frequencies in a set of sample proteins of known structure. They concluded that: - Ala, Met, and Glu are α-helix formers, - Tyr, Ile, and Val are β-sheet formers, - Pro, Asn, and Gly are most frequent in β-turns. Proline is a peculiar amino acid. It is unique because it is the only amino acid where the side chain is connected to the protein backbone twice, forming a five-membered nitrogen-containing ring (see figure). Peptide bonds between amino acid residues other than proline are all in the trans configuration. When proline is involved, about 6% of the bonds are in the cis configuration. Prolines are rarely found in α-helices and β-sheets: - The nitrogen atom of a proline residue in a protein has no substituent hydrogen to participate in hydrogen bonds with other residues further down the chain. - In proline, rotation about the N-Cα bond is not possible. Proline introduces a destabilizing kink in an α-helix. Obviously, the position of an amino acid residue relative to its neighbours is also important. For example, if a polypeptide chain has a long block of Glu residues, this segment of the chain will not form an α-helix at pH 7.0. The negatively charged carboxyl groups of adjacent Glu residues repel each other so strongly that they prevent formation of the α-helix. In class? In class, you will answer exam-style questions on the concepts covered in this lesson. You will not be marked on your in-class answers. 1. If the polypeptide chain shown below were in a right-handed α-helix then the hydrogen of the α-amino group of amino acid 10 (the one with the R10 sidechain) would be in a hydrogen bond with the oxygen of the α-carboxyl group of... ❑ R5 ❑ R6 ❑ R7 ❑ R13 ❑ R14 ❑ R15 How far apart would residues 4 and 10 be?... 2. Where in a protein are you most likely to find the β-strand Leu – Asp – Met – Arg – Ala – Glu – Ile? ❑ Fully solvent-exposed ❑ Buried in the interior of the protein ❑ On the surface of the protein What is the approximate length of this β-strand?... 3. Analyse the information below. Is the β-turn shown... Type I? Yes / No Type II? Yes / No 4. Which of the following polypeptides is most likely to form an α-helix? Peptide 1: E M L Q S A L A E R Peptide 2: T A N D S G P R R Y ❑ Peptide 1 will most likely form an α-helix, peptide 2 is least likely to form an α-helix ❑ Peptide 2 will most likely form an α-helix, peptide 1 is least likely to form an α-helix ❑ Both peptides have roughly the same likelihood to form an α-helix ❑ Neither peptide is likely to form an α-helix ❑ You cannot predict secondary structure from primary structure Resources More information can be found in the following textbooks: Lehninger Principles of Biochemistry; David L. Nelson, Michael M. Cox 2.1 Weak Interactions in Aqueous Systems (but not “Osmotic Pressure”) 4.2 Protein Secondary Structure (but not “Circular Dichroism”) Biochemistry; Jeremy M. Berg, Gregory J. Gatto Jr., Justin K. Jines, John L. Tymoczko, Lubert Stryer 1.3 Concepts from Chemistry Explain the Properties of Biological Molecules: Covalent bonds and noncovalent interactions are important for structure and stability of biological molecules; and The formation of DNA’s double helix is an expression of the rules of chemistry 2.4 Secondary Structure: Polypeptide Chains Can Fold into Regular Structures Both are available through Kortext using your [email protected] and password.