DNA and RNA Structure and Function Summary PDF
Document Details
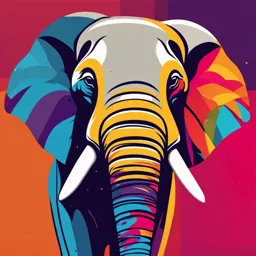
Uploaded by StableElder
Monash University
Tags
Summary
This document summarizes the primary and secondary structures of DNA and RNA, including nitrogenous bases, nucleotides, bonding, and various functions. It also explores the link between DNA structure and physical properties like solubility and UV absorption. This detailed summary will help students grasp the fundamentals of nucleic acid structure and function.
Full Transcript
**Demonstrate an understanding of the primary and secondary structures of DNA and RNA** - Primary structure = linear sequence of nucleotides +-----------------------+-----------------------+-----------------------+ | | DNA | RNA | +======...
**Demonstrate an understanding of the primary and secondary structures of DNA and RNA** - Primary structure = linear sequence of nucleotides +-----------------------+-----------------------+-----------------------+ | | DNA | RNA | +=======================+=======================+=======================+ | Nitrogenous bases | Pyrimidines: | Pyrimidines: | | | | | | | - Thymine | - Uracil | | | | | | | - Cytosine | - Cytosine | | | | | | | Purines: | Purines: | | | | | | | - Guanine | - Guanine | | | | | | | - Adenine | - Adenine | +-----------------------+-----------------------+-----------------------+ | Nucleotides (monomer | Phosphodiester bond | Phosphodiester bond | | of nucleic acids) and | (covalent) -- 5' | (covalent) -- 5' | | their linkage | phosphate (C5) to 3' | phosphate (C5) to 3' | | | OH (C3) | OH (C3) | +-----------------------+-----------------------+-----------------------+ | Information storage | Carries genetic | Carries information | | in base sequences | information for | from DNA to | | | heredity | synthesise proteins | +-----------------------+-----------------------+-----------------------+ | Role | Constitutes and | Mostly involved in | | | encodes our genes | protein synthesis | +-----------------------+-----------------------+-----------------------+ | Stability | Stable: copied and | Short lived (less | | | transferred from cell | stable): 3 main | | | to cell; from | types; messenger, | | | generation to | transfer and | | | generation | ribosomal | +-----------------------+-----------------------+-----------------------+ | Where found | Nucleus and | Cytoplasm, nucleus | | | mitochondria | and mitochondria | +-----------------------+-----------------------+-----------------------+ | Charge | Negatively charged at | Negatively charged at | | | physiological pH | physiological pH | | | therefore acid | therefore acid | +-----------------------+-----------------------+-----------------------+ - Secondary structure = folding of motifs +-----------------------+-----------------------+-----------------------+ | | DNA | RNA | +=======================+=======================+=======================+ | Complementary base | Adenine pairs with | Adenine pairs with | | pairing | Thymine (A-T) | Uracil (A-U), Guanine | | | | pairs with Cytosine | | | Guanine pairs with | (G-C) | | | Cytosine (G-C) | | +-----------------------+-----------------------+-----------------------+ | Structure | Double stranded helix | Typically, | | | | single-stranded; can | | | Anti-parallel | form complex | | | orientations of | secondary structures | | | strands | (e.g., hairpins, | | | | loops) | +-----------------------+-----------------------+-----------------------+ A diagram of a chemical compound Description automatically generated   Pyrimidine nucleosides = cytidine, uridine and thymidine Purine nucleosides = adenosine and guanine Pyrimidine nucleotides = cytidine monophosphate, uridine monosphosphate and thymidine monophosphate Purine nucleotides = adenosine monophosphate and guanine monophosphate Nucleotide = nucleoside + phosphate = monomer of nucleic acids  A diagram of chemical structures Description automatically generated 4 key features that define DNA structure: - Minor and major grooves - Uniform diameter of 2 nm in its most common B form - Right-handed twist with 10-10.5 base pairs per turn - Antiparallel **Explain how DNA can act as a template** - Double stranded nature of DNA - Because each strand of DNA contains a sequence of nucleotides that is exactly complementary to the nucleotide sequence of its partner strand, each strand can act as a template for the synthesis of a new complementary strand  **Associate specific DNA structures to their function in the storage, replication and expression of genetic information.** Structural features of DNA that allow for storage of genetic information: - Made of 4 nucleotides the genetic information is stored in the order of the 4 bases - Sequence of bases which order is maintained by a covalent phosphoribose backbone a stable backbone maintains long term storage of the genetic information (e.g. resistance to heat) - Double helical structure Structural features of DNA that allow for it to be precisely copied (replication: - Weak bonds between strands weak bonds allow separation of the strands by enzymes that replicate DNA - Base complementarity accurate A-T and C-G pairing is essential for the fidelity of DNA replication Structural features of DNA that allow DNA to be read for gene expression: - Base complementarity accurate A-T and C-G pairing essential for transcription - Weak bonds between strands weak bonds allow separation of the strands by enzymes that transcribe DNA **Identify the 4 main species of RNAs and their general functions in the cell** mRNA - Directs amino acid sequence of polypeptides - Code for proteins rRNA - Component of the ribosome that serves as the site of protein synthesis - Forms the basic structure of the ribosome - Catalyses protein synthesis tRNA - Binds to amino acid and directs them to proper locations within the growing polypeptide chain - Central to protein synthesis as adaptors between mRNA and amino acids snRNA - Function in a variety of nuclear processes including the splicing of pre-mRNA **Identify the link between specific structures of DNA and its physical properties** Double stranded helix Provides stability and protection for genetic information Complementary base pairing between strands Ensures accurate replication and transcription Hydrogen bonding that maintains helix Allows strands to separate during replication and transcription, while providing overall stability Length/diameter Facilitates organisation and interaction with proteins Acidity due to negatively charged phosphate groups Influences interactions with proteins and affects solubility and stability **Describe the structural basis of DNA's solubility, viscosity, UV absorption, acidity, denaturation and renaturation** Melting curve: - A graph plotting temperature against denatured DNA (%) representing the transition of DNA from double stranded DNA to single stranded DNA. - The graph can be used to determined T~m~ by working out where the DNA is 50% denatured. - Steepness of transition depends on the complexity of DNA - Steeper -- melts over a narrower temperature range and is less complex Melting temperature equation:  - Example question: - In total, the double-stranded DNA has 30% of guanine bases. The melting temperature (Tm) of dsDNA is given by the formula: Tm = 69.4 + 0.41(%G+C) -- 650/N where N is the number of base pairs. What is the melting temperature? - 69.4 + (0.41 x 30) = 81.7 = closest to 81^o^ Factors that impact T~m~ +-----------------------+-----------------------+-----------------------+ | Factor | Description | Effect on Melting | | | | Temperature (T~m~) | +=======================+=======================+=======================+ | GC Content | The proportion of | Higher GC content | | | guanine (G) and | increases T~m~ due to | | | cytosine (C) bases. | the three hydrogen | | | | bonds between G and | | | | C, compared to the | | | | two hydrogen bonds | | | | between adenine (A) | | | | and thymine (T) | | | | therefore higher | | | | levels of energy | | | | required to denature | | | | or break these bonds | | | | therefore higher | | | | melting temperature | | | | required to melt the | | | | DNA than one of the | | | | same lengths with a | | | | lower GC percentage. | +-----------------------+-----------------------+-----------------------+ | Length of DNA/Size | The total number of | Longer DNA strands | | | base pairs in the DNA | generally have higher | | | sequence. | T~m~ because more | | | | base pairs contribute | | | | to more hydrogen | | | | bonds and stacking | | | | interactions. | | | | | | | | Increased length | | | | increases the | | | | T~m.~ The overall | | | | length of the DNA can | | | | also affect the T~m~. | | | |  Fragments that are | | | | shorter in length | | | | will | | | | denature *quicker* th | | | | an | | | | longer fragments. | | | |  This results in the | | | | transition occurring | | | | over a shorter range | | | | of temperatures and | | | | will increase the | | | | gradient or | | | | \'steepness\' of the | | | | melting curve | +-----------------------+-----------------------+-----------------------+ | Salt Concentration | The amount of salt | Higher salt | | | (e.g., NaCl) in the | concentrations | | | solution. | stabilise the DNA | | | | duplex by shielding | | | | negative charges on | | | | the phosphate | | | | backbone, thus | | | | increasing T~m~. | | | | | | | | MgCl2 stabilises the | | | | double-stranded form. | +-----------------------+-----------------------+-----------------------+ | Presence of | Chemicals like urea | Denaturants lower the | | Denaturants | or formamide that can | Tm by disrupting | | | destabilize DNA. | hydrogen bonds and | | | | base stacking | | | | interactions. | +-----------------------+-----------------------+-----------------------+ | DNA Sequence | The specific | Sequences with more | | | arrangement of | GC pairs or repeated | | | nucleotides. | motifs can have | | | | higher Tm due to | | | | stronger base pairing | | | | and stacking | | | | interactions. | +-----------------------+-----------------------+-----------------------+ | pH of the Solution | The acidity or | Extreme pH levels can | | | basicity of the | destabilise the DNA | | | solution. | duplex, usually | | | | lowering T~m~ by | | | | affecting the | | | | hydrogen bonding and | | | | base stacking. | | | | | | | | Alkaline pH | | | | destabilises dsDNA. | +-----------------------+-----------------------+-----------------------+ | Complexity | The complexity of a | A low complexity of | | | DNA molecule is how | the DNA will result | | | diverse the sequence | in the transition | | | is (i.e. the absence | occurring over a | | | of repeats or short | shorter range of | | | motifs like | temperatures and will | | | TATATATA\...). | increase the gradient | | | | or \'steepness\' of | | | | the melting curve. | +-----------------------+-----------------------+-----------------------+ +-----------------+-----------------+-----------------+-----------------+ | Property of DNA | Reason | Factors that | Factors that | | | | decrease that | increase that | | | | property | property | +=================+=================+=================+=================+ | Solubility | DNA is | Insoluble in | Soluble in | | | polyanionic due | alcohol | water | | | to its high | | | | | phosphate | Precipitates at | | | | content, | neutral pH in | | | | enhancing | salt presence | | | | solubility in | (forms salt | | | | water | with ions) | | +-----------------+-----------------+-----------------+-----------------+ | Viscosity | Large, rigid, | Transition from | Increasing size | | | rodlike | double stranded | of molecule | | | structure of | to single | increases | | | DNA leads to | stranded DNA | viscosity | | | high | decreases | | | | hydrodynamic | viscosity | Lower | | | volume, | | temperature and | | | attracting a | Mechanical | lower pH | | | large water | shearing | increase | | | shell | (fragments DNA | viscosity | | | | molecule | (because double | | | | thereby | stranded DNA is | | | | interrupting | more viscous | | | | gene sequence) | than single | | | | decreases | stranded DNA) | | | | viscosity | | +-----------------+-----------------+-----------------+-----------------+ | UV absorption | Nitrogenous | UV absorbance | Double-stranded | | | bases absorb UV | is higher in | DNA absorbs | | | light maximally | single-stranded | less UV than | | | at 260 nm; dye | DNA compared to | single-stranded | | | in the minor | double-stranded | DNA | | | groove enhances | DNA | | | | light emission | | | | | under UV | | | +-----------------+-----------------+-----------------+-----------------+ | Acidity | DNA is acidic | Shorter DNA | Longer DNA | | | due to its | strands (fewer | strands (more | | | negatively | phosphate | phosphate | | | charged | groups) reduce | groups) | | | phosphate | acidity | increase | | | groups | | acidity | +-----------------+-----------------+-----------------+-----------------+ | Denaturation | Disruption of | Lower | Increasing | | | hydrogen bonds | temperature and | temperature and | | | converts DNA | extreme pH | extreme pH | | | from | decrease | increase | | | double-stranded | denaturation | denaturation | | | to | | | | | single-stranded | | | | | form | | | +-----------------+-----------------+-----------------+-----------------+ | Renaturation | Annealing | Very large, | Smaller | | | occurs under | complex | molecules with | | | favourable | molecules are | complementary | | | conditions, | less likely to | strands are | | | allowing | reform and tend | more likely to | | | reformation of | to clump | renature into | | | double-stranded | | soluble DNA | | | DNA | | | +-----------------+-----------------+-----------------+-----------------+ **Demonstrate an understanding of the process of hybridisation between nucleic acids and the technical use of probes** Hybridisation = DNA renaturation = reformation of the hydrogen bonds between complementary base pairs - For 2 single strand molecules to hybridise, they must have complementary nucleotide sequences that allow base pairing - Nucleic acids from different species can form hybrids through complementary base pairing - Hybridisation is useful for detecting specific nucleotide sequences Process of hybridisation  Hybridisation can occur between DNA or RNA or both i.e. DNA-RNA - DNA Hybridisation - Structure: DNA is double-stranded and has a stable helical structure. - Function: DNA hybridisation is often used in techniques like Southern blotting and in the development of DNA probes for detecting specific sequences. - Applications: Useful in genetic analysis, diagnostics, and detecting genetic disorders. - RNA Hybridisation - Structure: RNA is typically single-stranded and can form complex structures, allowing for more versatility. - Function: RNA hybridisation is crucial for techniques like Northern blotting and in the study of gene expression. - Applications: Often used in studying mRNA levels, detecting specific RNA molecules, and in applications like in situ hybridisation. - Key Differences 1. Stability: DNA is more stable than RNA, making it better suited for long-term storage and detection. 2. Complexity: RNA's ability to fold into various structures can allow for more intricate interactions in hybridisation studies. 3. Complementarity: The base pairing rules (A-T in DNA; A-U in RNA) lead to differences in hybridisation techniques. Probes - Labelled DNA or RNA fragments are used to detect specific nucleic acid (DNA or RNA) sequences by hybridisation - Single stranded - Typically, 30 nucleotides in length - Highly specific - Can be labelled with fluorescent tags - Can be used on membranes, cells, tissues... - Can be used to locate genes on isolated chromosomes FISH - Where some fluorescent probes are used to look at the localisation of specific regions of DNA and can follow whole chromosomes - Labels entire chromosome therefore can detect chromosomal anomalies - Used to detect and localise DNA sequences fluorescently labelled with probes A diagram of dna sequence Description automatically generated **Interpret the behaviour of DNA molecules during gel electrophoresis** Gel electrophoresis separates DNA molecules based on size - Larger DNA fragments move slower - Small DNA fragments move faster - DNA fragments become spread out across the gel according to size, forming a ladder of discrete bands, each composed of a collection of DNA molecules of identical length - DNA is negatively charged so moves from negative to positive end - DNA molecules longer than 500 nucleotide pairs use agarose gel lower discriminative power/resolution - DNA molecules less than 500 nucleotide pairs use polyacrylamide gel higher discriminative power/resolution - Linear DNA = only usable form for reliable gel electrophoresis predictable pattern based on size (and standard ladders made using linear DNA) - Cannot predict migration pattern of circular DNA as depends on shape, size and mixture of complex factors - Supercoiled DNA migrates faster in gel electrophoresis because more compact **Demonstrate an understanding of the Meselson-Stahl experiment** A diagram of a model Description automatically generated Meselson-Stahl Experiment steps 1. Preparation of DNA: Bacteria (E. coli) were grown in a medium containing heavy nitrogen (15N), allowing the incorporation of this heavy isotope into their DNA. 2. Transfer to Light Nitrogen: After several generations, the bacteria were transferred to a medium containing the lighter nitrogen isotope (14N). This switch allowed the bacteria to replicate their DNA in the presence of light nitrogen. 3. Isolation of DNA Samples: Samples of the bacterial culture were taken after one and two rounds of replication. 4. Density Gradient Centrifugation: The DNA samples were subjected to centrifugation in a caesium chloride gradient. This method separated the DNA based on density, allowing visualisation of the different DNA strands. 5. Analysis of Results: - After one round of replication, all DNA had an intermediate density, indicating that each DNA molecule contained one heavy and one light strand. - After two rounds, two distinct densities were observed: one corresponding to fully light DNA (14N/14N) and another corresponding to intermediate density (15N/14N). This experiment demonstrated that during DNA replication, each original strand serves as a template for a new strand, supporting the semi-conservative model of DNA replication. - Semi-conservative model each parent strand is a template for synthesis of a new strand - Two replicated DNA helices contain one parent strand and one newly synthesised strand **List the components involved in DNA replication, identify their function and outline how they come together at the replication fork** +-----------------------+-----------------------+-----------------------+ | Components involved | Definition | Function | | in DNA replication | | | +=======================+=======================+=======================+ | DNA polymerase | Enzyme that | Catalyses the | | | synthesises DNA by | addition of | | | joining nucleotides | nucleotides to the 3' | | | together using a DNA | end of the growing | | | template as a guide; | strand -- nucleotides | | | its substrates are | are added by | | | the 4 | complementary base | | | deoxyribonucleoside | pairing with the | | | triphosphates (dNTPs | template strand | | | = A, G, C, and T) | | | | | Synthesises only in | | | | the 5' to 3' | | | | direction | +-----------------------+-----------------------+-----------------------+ | DNA primase | Enzyme that | Catalyses the | | | synthesises a short | synthesis of short | | | strand of RNA on a | RNA primers to which | | | DNA template, | nucleotides are added | | | producing an RNA | | | | primer for DNA | | | | synthesis | | +-----------------------+-----------------------+-----------------------+ | DNA ligase | Enzyme that joins the | | | | ends of 2 strands of | | | | DNA together with a | | | | covalent bond to | | | | produce one | | | | continuous DNA strand | | +-----------------------+-----------------------+-----------------------+ | DNA helicase | Enzyme that harnesses | Hydrolyses ATP and | | | ATP hydrolysis energy | propels rapidly along | | | to open a region of | a DNA single strand | | | the DNA helix into | prying apart the | | | its single strands as | helix at rates up to | | | an aid to DNA | 1000 nucleotide pairs | | | replication or DNA | per second | | | repair | | +-----------------------+-----------------------+-----------------------+ | dNTP | Substrates during DNA | ATP | | (deoxyribonucleotide | replication (as | | | triphosphates) | they're the building | TTP | | | blocks of the DNA) | | | | and also provide | GTP | | | energy for | | | | polymerisation | CTP | | | reaction by cleavage | | | | of high energy | | | | terminal phosphates | | | | for the | | | | polymerisation | | | | reaction | | +-----------------------+-----------------------+-----------------------+ | SSB (single-stranded | Protein that binds to | Binds tightly and | | DNA binding protein) | the single strands of | cooperatively to | | | the opened-up DNA | exposed single | | | double helix, | stranded DNA without | | | preventing helical | covering the bases | | | structures from | | | | re-forming with the | Stabilises the | | | DNA is being | unwound DNA | | | replicated | | +-----------------------+-----------------------+-----------------------+ | Replication fork | Y-shaped region of a | At the replication | | | replicating DNA | fork, a multienzyme | | | molecule; the point | complex that contains | | | at which the 2 | the DNA polymerase | | | strands of the parent | synthesises the DNA | | | DNA helix are being | of both new daughter | | | separated and the | strands | | | daughter strands are | | | | being formed | | +-----------------------+-----------------------+-----------------------+ | Sliding clamp | Accessory protein | Prevents the | | | that keeps the | polymerase from | | | polymerase firmly on | dissociating without | | | the DNA when it is | impeding the | | | moving but releases | polymerases rapid | | | the polymerase as | movement along DNA | | | soon as it runs into | | | | a double stranded | | | | region of DNA | | +-----------------------+-----------------------+-----------------------+ | Topoisomerase | enzyme that binds to | | | | DNA and reversibly | | | | breaks a | | | | phosphodiester bond | | | | in one or both | | | | strands | | +-----------------------+-----------------------+-----------------------+ | Replication origin | A location on a DNA | Location at which | | | molecule at which | localised unwinding | | | duplication of the | (denaturation) of DNA | | | DNA begins by the | takes place | | | formation of | | | | replication forks | | +-----------------------+-----------------------+-----------------------+ | Primer | | | +-----------------------+-----------------------+-----------------------+ | Okazaki fragments | | | +-----------------------+-----------------------+-----------------------+ | Leading strand | One of the 2 newly | Daughter strand that | | | synthesised strand of | is synthesised | | | DNA found at a | continuously | | | replication fork, | | | | made by continuous | | | | synthesis in the 5' | | | | to 3' direction | | +-----------------------+-----------------------+-----------------------+ | Lagging strand | One of the 2 newly | Daughter strand that | | | synthesised strands | is synthesised | | | of DNA found at a | discontinuously | | | replication fork, | | | | made in discontinuous | | | | lengths that are | | | | later joined | | | | covalently | | +-----------------------+-----------------------+-----------------------+ | Clamp loader | Protein complex that | Regulates the | | | utilises ATP | assembly of the | | | hydrolysis to load | sliding clamp around | | | the sliding clamp | the DNA by opening | | | onto a | and closing the | | | primer-template | sliding clamp in a | | | junction in the | regulated manner | | | process of DNA | | | | replication | | +-----------------------+-----------------------+-----------------------+   How the components of DNA replication come together at the replication fork 1. DNA helicase and single-stranded DNA binding (SSB) proteins help open the DNA helix 2. DNA primase kick-starts the DNA polymerase 3. Clamp increases the processivity of DNA polymerase 4. DNA polymerase catalyses nucleotide polymerisation 5. DNA topoisomerases relieve helical binding 6. RNase H removes the RNA primers which are replaced by DNA 7. DNA ligase seals together discontinuously synthesised lagging-strand DNA fragments **Describe how the DNA polymerase base-pairing recognition and 3'-5' exonuclease activity enhances the fidelity of DNA replication** DNA polymerase base-pairing recognition occurs before covalent binding - After complementary nucleotide binding but before the nucleotide is covalently added to the growing chain, DNA polymerase must undergo a conformational change in which its 'grip' tightens around the active site - Because this change occurs more readily with correct than incorrect base pairing, it allows the polymerase to 'double check' the exact base-pair geometry before it catalyses the addition of the nucleotide - Incorrectly paired nucleotides are harder to add and therefore more likely to diffuse away before the polymerase can mistakenly add them 3' to 5' exonuclease activity occurs after covalent binding - Occurs immediately after those rare instances in which an incorrect nucleotide is added to a growing chain - DNA polymerase enzymes are highly discriminating in the types of DNA chains they will elongate; they require a previously formed, base-paired 3' OH end of a primer strand - DNA molecules with a mismatched nucleotide at the 3' OH end of a primer strand is not effective as templates because the polymerase has difficulty extending such a strand -- correct nucleotide has higher affinity for polymerase (more energetically favourable) - DNA polymerase molecules correct such a mismatched primer strand by means of a separate catalytic site - 3' to 5' proofreading endonuclease clips off any unpaired or mispaired residues at the primer terminus, continuing until enough nucleotides have been removed to regenerate a correctly base paired 3' OH terminus that can prime DNA synthesis - In this way DNA polymerase functions as a self-correcting enzyme that removes its own polymerisation errors as it moves along the DNA Mismatch repair mechanism in bacteria -- strand directed mismatch repair - In E.coli, DNA methylation adds methyl groups to all A nucleotides in the sequence GATC, but not immediately during replication - Therefore, GATC sequences that have not yet been methylated are in the new strands just behind the replication fork - 3 step process: 1. Recognition of a mismatch 2. Excision of the segment of DNA with mismatch 3. Resynthesis of the excised segment using old strand as template - Reduces number of errors made by factor of 100-1000 - Parent DNA molecule is methylated but not the newly synthesised one Mismatch repair in eukaryotes vs prokaryotes Prokaryotes Eukaryotes ----------------------- ------------------------------------------- ------------------------------------------------------------ Recognition MutS detects mismatches MSH proteins (e.g., MSH2-MSH6) detect mismatches Repair proteins MutL and MutH MLH proteins (e.g., MLH1-PMS2) Strand discrimination Based on DNA methylation of parent strand Nicks in the newly synthesised strand Exonuclease Various exonucleases Exo1 and others DNA polymerase Fills in the gap Similar role, with specific polymerases Timing During or immediately after replication Often more complex and integrated with cell cycle controls Complexity Simpler mechanism More complex, involving multiple pathways **Describe the organisation of eukaryotic chromosomes** Eukaryotic chromosomes - DNA is organised as fibre like-structures called chromosomes - Each chromosome consists of a single linear DNA molecule - Number of chromosomes varies between different species - Eukaryotic organisms generally diploid (contain two copies of each chromosome) Nucleosomes - Nucleosomes are the basic unit of eukaryotic chromosome structure - Beadlike structure in eukaryotic chromatin, composed of a short length of DNA wrapped around an octameric core of histone proteins (histones are positively charged) - Nucleosomes are the fundamental structural unit of chromatin - Invariant component of euchromatin and heterochromatin - Composed of: - A core region comprising 2 copies each of the histone proteins H2A, H2B, H3 and H4 (histones are small basic proteins with a positive charge rich in Lys and Arg) - DNA wound around the core (146 bp of DNA/octamer of histones plus approximately 50 bp linker DNA wrapped as slightly less than 2 turns around the core - An associated H1 protein binds the linker DNA and is involved in compaction Chromatin - Complex of DNA, histones and non-histone proteins found in the nucleus of a eukaryotic cell - The material of which chromosomes are made - Density of chromatin packaging is flexible -- alters during different phases of cell cycle - During mitosis and meiosis, chromatin becomes 5-10 times more densely packed -- only time you can visualise chromosomes - Less tightly packed between mitotic cycles  **Apply an understanding of changes in chromosome organisation during the different phases of mitosis to explain the role of centromeres** Centromeres - Situated near the centre of chromosomes - Direct segregation during mitosis and meiosis - Contains site at which sister chromatids are paired before segregation - Section that pulls chromosomes to either pole - Interacts with proteins (kinetochore) and microtubules - Contains short, highly repetitive sequences (satellite DNA) - During mitosis, the centromere attaches the duplicated chromosomes to the mitotic spindle so that a copy of the entire genome is distributed to each daughter cell during mitosis; the special structure that attaches the centromere to the spindle is the kinetochore - The centromere also helps to hold the duplicated chromosomes together until they are ready to be moved apart Changes in chromosome organisation during the different phases of mitosis 1. Prophase: - During prophase, chromatin condenses into visible chromosomes, each consisting of two sister chromatids held together at the centromere. - The centromere is the region where the chromatids are tightly joined and where kinetochores will form. 2. Prometaphase: - The nuclear envelope breaks down, and spindle fibres attach to the kinetochores, which are protein structures assembled at the centromeres. - The proper attachment of spindle fibres to kinetochores is essential for accurate chromosome alignment and segregation. 3. Metaphase: - Chromosomes align at the metaphase plate, with centromeres oriented toward the spindle poles. - The tension generated by the spindle fibres pulling on the kinetochores ensures that each chromosome is correctly positioned and prepares them for separation. 4. Anaphase: - The centromeres play a critical role in the separation of sister chromatids. - As the spindle fibres shorten, the centromere divides, allowing the sister chromatids to be pulled apart toward opposite poles of the cell. - This separation is crucial for ensuring that each daughter cell receives an identical set of chromosomes. 5. Telophase: - After the chromatids reach the poles, the chromosomes begin to decondense. - The centromeres remain important as the nuclear envelope re-forms around each set of chromosomes, completing the process of mitosis. A diagram of different types of cell division Description automatically generated **Demonstrate an understanding of the function and replication of telomeres** Function of telomeres - Cap the end of eukaryotic chromosomes - Contain multiple copies of non-coding tandem repeat sequences - Measuring stick -- can determine how many cell cycles that cell has undergone - Ensure nucleotides not lost during cell division - Ensures that after many generations, the cells will not inherit defective chromosomes and ultimately cease dividing (replicative senescence) - Safeguards against uncontrolled proliferation Replication of telomeres 1. The 3' end of the parental DNA strand is extended by RNA-templated DNA synthesis 2. Replication of the lagging strand at the chromosome end can be completed by DNA polymerase using these extensions as a template  Telomerase - Telomere DNA sequences are recognised by sequence-specific DNA binding proteins that attract telomerase - Telomerase recognises tip of existing telomere DNA repeat sequence and elongates it in the 5' to 3' direction using an RNA template that is a component of the cell itself - The enzymatic portion of telomerase has a reverse transcriptase activity i.e. synthesises DNA using and RNA template - This extends the parental DNA strand so that replication of the lagging strand at the chromosome end can be completed by DNA polymerase **Describe differences in sizes, genomic material and genomic organisations between viruses, parasites and bacteria** +-----------------+-----------------+-----------------+-----------------+ | | Viruses | Parasites | Bacteria | +=================+=================+=================+=================+ | Size | \ 20 cycles without inactivation - Temperature cycling to allow primer binding (annealing temperature) and optimum DNA polymerase activity (extension temperature) Components of PCR Component Function Effect of too little Effect of too much --------------------- ----------------------------------------------------------------------------------------------------------- -------------------------------------------------------------------- ---------------------------------------------------------------------------- Taq polymerase Synthesises new DNA strands by adding dNTPs to primers -- thermostable unlike DNA polymerase usually used Inefficient or no amplification Increased errors in DNA synthesis; potential non-specific amplification DNA template Provides the target sequence for amplification No product or low yield of PCR product Increased background noise; potential for non-specific amplification MgCl2 Acts as a cofactor for Taq polymerase activity Reduced enzyme activity; poor amplification Inhibition of Taq polymerase; can lead to non-specific products Primers Short sequences that initiate DNA synthesis (single stranded DNA) No amplification; failure to bind Non-specific binding; increased chances of primer-dimer formation dNTP Building blocks for new DNA strands Insufficient material for DNA synthesis Imbalance in nucleotide concentrations; potential for incorporation errors PCR buffer Maintains optimal pH and salt conditions for the reaction Unstable reaction conditions; poor yield May inhibit enzyme activity if conditions are not optimal Nuclease free water Dilutes and adjusts the concentrations of other components Increased concentration of other components; inefficient reactions Over-dilution of other components; potential for suboptimal reactions **Describe applications of probes and PCR in medicine and research** Applications of probes - Determining if a specific gene is present and where it is - In situ analysis -provides spatial information - By convention, single strands of DNA and RNA are written in 5' to 3' direction (including probe sequences) Applications of end-point PCR - Altering DNA sequence (site-directed mutagenesis) -- used to introduce amino acid changes, restriction sites and changing regulatory sequences - Amplify DNA for cloning purposes - Amplify DNA to detect a sequence - Obtain genomic or cDNA clones Applications of qPCR - Gene expression profiling - Copy number variation - Molecular diagnostics - Genotyping - Detect level of gene expression PCR applications in medicine and research - Genetic testing - Paternity testing - Forensic science RT-PCR application = amplification for sequencing - RT-PCR (Real time PCR) DNA amplification is detected in real time with the help of a fluorescent reporter, the signal strength of the fluorescent reporter is directly proportional to the number of amplified DNA molecules qRT-PCR application = to determine the level of expression of a certain gene - qRT-PCR (quantitative real time PCR) uses the DNA amplification linearity to detect, characterise and quantify a known sequence in a sample cDNA library application = to determine the differences in genes expressed in muscle vs liver tissue Genome library application = to compare the genetic differences between two different species **Apply an understanding of genomic and cDNA libraries to compare the two approaches**  +-----------------------+-----------------------+-----------------------+ | | Genomic DNA library | cDNA library | +=======================+=======================+=======================+ | How it is generated | Generated by | Generated by reverse | | | digesting genomic DNA | transcribing (using | | | with restriction | reverse | | | enzymes and cloning | transcriptase) mRNA | | | the fragments into | into cDNA and cloning | | | plasmid vectors | it into plasmid | | | | vectors | +-----------------------+-----------------------+-----------------------+ | What it contains | Contains the entire | Contains only the | | | genomic DNA, | cDNA copies of | | | including coding | expressed mRNA, | | | regions (exons), | representing the | | | non-coding regions | genes actively | | | (introns), regulatory | transcribed in a | | | sequences, and | specific cell type or | | | intergenic regions | condition | +-----------------------+-----------------------+-----------------------+ | Applications | Used for mapping | Used for studying | | | genomes, identifying | gene expression, | | | genes, studying gene | identifying expressed | | | function, and | genes in specific | | | analysing genetic | tissues or | | | variations | conditions, and | | | | producing recombinant | | | | proteins | +-----------------------+-----------------------+-----------------------+ | Information provided | Provides information | Provides insights | | | about the entire | into gene expression | | | genome structure, | patterns, alternative | | | including non-coding | splicing, and the | | | regions, gene | presence of specific | | | locations, and | transcripts in a | | | regulatory elements | given cell type or | | | | condition | +-----------------------+-----------------------+-----------------------+ | More information | Exhaustive | Provides information | | | | about the identity | | | Used for sequences | and quantity of each | | | | transcript | | | Untranscribed DNA | | | | used in some | | | | techniques (e.g. | | | | RFLP) | | +-----------------------+-----------------------+-----------------------+ **Demonstrate a basic understanding of the principles of DNA microarrays and its applications to gene expression analysis** Microarray is prepared by attachment or synthesis of DNA probes - Spotted arrays: attachment of probes prepared separately - Oligonucleotide arrays: in situ synthesis of oligonucleotide probe Genome chips (gene arrays) may have \> 5 million probes - Designed for a specific application e.g. human vs mouse, disease-specific, bacterial genotyping...) Microarray starting material mRNA extracted from cells of interest Applications of microarrays for gene expression analysis - DNA microarrays analyse gene expression by monitoring mRNA products of thousands of genes at once - Convert mRNA from cell being studied into cDNA which is then labelled with a fluorescent probe - Labelled probe is hybridised to microarray - Scanned to see which genes the probe binds to - Reference probe used to give relative expression  **Demonstrate an understanding of the role of restriction endonucleases in nature and in the laboratory** - **describe the characteristics of type II restriction enzymes** - Type II Restriction Endonuclease recognise palindromic sequences and cut both strands in the same way, within or close to the recognition site - palindromic means that the sequence is identical whether read from one end or the other (but always in the 5\' to 3\' direction, i.e. a different strand is read for each direction) - Recognition sites are sequence depend, though with varying degrees of stringency - Always cut both strands in the same way (create blunt or sticky ends) - Most sites are 4-8 nucleotides long and palindromic - Requires Mg2+ but not ATP - Used singly or in combination - Cornerstone of recombinant DNA technology - Purified from bacterial systems - Sticky 5' or 3' overhangs or blunt cuttings - Part of bacteria's phage/viral immune system - **describe the nature and diversity of restriction sites** - Enzymes cut near or at recognition sequence (recognition sites often palindromic) - Can cut the DNA in 2 different ways: - Cleavage leaves blunt ends - Cleavage leaves staggered or sticky ends (5' or 3' sticky ends)  Major uses of endonucleases - Generation of reproducible, manageable-sized family of fragments -- DNA sequencing - Gene cloning -- recombinant protein expression (e.g. insulin) - DNA profiling/fingerprinting -- comparative studies, forensics - Gene mapping -- follow the creation/destruction of sites due to mutation of the gene sequence - **understand the role of methylation in phage restriction** - Methylation in bacteria serves as a protective mechanism against phage infection by distinguishing self-DNA from foreign DNA, allowing restriction enzymes to selectively cleave unmethylated viral genomes while preserving the integrity of the bacterial genome **Apply the principles of gene cloning to solve problems in research and medicine.** - **Demonstrate an understanding of the function of the main components in a typical expression plasmid** +-----------------------------------+-----------------------------------+ | Function | Components | +===================================+===================================+ | Efficient expression | Promoter | | | | | | Ribosome-binding site | | | | | | Transcription termination | | | sequence | +-----------------------------------+-----------------------------------+ | Inducible expression | Repressor gene | | | | | | Operator sequence | +-----------------------------------+-----------------------------------+ | Selection | Antibiotic (e.g. ampicillin) | | | | | | Blue-white selection (LacZ gene | | | disruption) | +-----------------------------------+-----------------------------------+ - **Demonstrate an understanding of directional cloning and plasmid-induced selection** Directional Cloning - Definition: A technique that allows for the insertion of a DNA fragment into a plasmid in a specific orientation. - Mechanism: - Different Restriction Sites: Utilises unique restriction enzyme sites at each end of the DNA fragment to be cloned. - Plasmid Preparation: The plasmid is digested with the same restriction enzymes, creating compatible ends for ligation. - Advantages: - Ensures the DNA fragment is inserted in the correct orientation, which is crucial for the proper expression of genes, especially when using promoters or other regulatory elements. A diagram of a dna sequence Description automatically generated Plasmid-Induced Selection - Definition: A method that uses selectable markers within a plasmid to identify successfully transformed cells. - Mechanism: - Selectable Markers: Common markers include antibiotic resistance genes (e.g., ampicillin resistance) or reporter genes (e.g., GFP). - Selection Process: After transformation, only the cells that have taken up the plasmid (containing the selectable marker) will survive in the presence of the antibiotic or express the reporter gene. - Advantages: - Facilitates the identification of successful cloning events, allowing for easy selection of cells containing the desired plasmid with the inserted DNA fragment.  Summary - Directional Cloning enables precise insertion of DNA fragments into plasmids using distinct restriction sites, ensuring correct orientation. - Plasmid-Induced Selection employs selectable markers to identify and isolate successfully transformed cells, enhancing the efficiency of cloning experiments. - **Demonstrate an understanding of the application of gene cloning to the understanding of protein function through site-directed mutagenesis and manipulation of gene expression.** Gene cloning applications in understanding protein function - DNA amplification (genomic library and DNA sequencing) - Protein expression (understanding gene function and applications in biotechnology and medicine) - Transgenic animals and plants (understanding gene function and applications in biotechnology and medicine) Site directed mutagenesis - Allows altering specific sequences - Uses PCR with modified oligonucleotides - Results in a change in the protein sequence (mutation, deletion or insertion) - Template DNA is degraded by using methylation-dependent restriction endonuclease  Manipulation of gene expression - Gene replacement (A) - Gene knockout (B) - Gene addition (C)  - Conditional mutations A diagram of dna binding Description automatically generated **Explain the principles of gene sequencing** - Requires DNA fragment of interest amplified by cloning or PCR - The DNA fragment needs to be denatured to serve as template for replication reaction (i.e. single stranded DNA) - In vireo system for DNA replication/polymerisation - High throughput and accurate method - To determine complete sequence, double stranded DNA is separated into single stranded DNA and one of the strands is used for sequencing - 4 different ddNTPs (dideoxy-ribonucleoside triphosphates) are used in 4 separate synthesis reactions -- ddNTPs differ from dNTPs (deoxyribonucleoside triphosphates) as they lack the 3' hydroxyl group - Each reaction produces a set of DNA copies that terminate at different points in time - The products of these 4 reactions are separated by electrophoresis in 4 parallel lanes of a polyacrylamide gel - Newly synthesised strands are detected by a label- usually isotope P32 or such - In each lane, the bands represent fragments that have terminated at a given nucleotide but at different positions in the DNA - By reading off the bands in order, starting from the bottom of the gel and reading across all 4 lanes, the DNA sequence of the newly synthesized strand can be determined - The sequence is complementary to the template strand Sanger sequencing steps 1. Amplification via PCR: - Use PCR to amplify the DNA fragment with standard deoxyribonucleoside triphosphates (dNTPs), generating a large template quantity. - Only 1 primer needed. 2. Amplification with chain-terminating nucleotides: - Perform a second round of amplification with a mix of dNTPs and a small amount of chain-terminating nucleotides (ddNTPs). - DNA polymerase synthesises new strands, incorporating ddNTPs, which halt further elongation, resulting in fragments of varying lengths. 3. Fragment Separation via Electrophoresis: - Separate the synthesised fragments by size using gel electrophoresis. Traditionally, this involves four reactions (one for each ddNTP) run in separate lanes. - Modern methods use capillary electrophoresis with fluorescently tagged ddNTPs, allowing all reactions in one tube. Fragments are detected by laser excitation of tags, with a computer analysing colours to assemble the DNA sequence.  original DNA fragment based on the gel = 5' TACTGGCAT 3' Next generation sequencing steps 1. Generation of a DNA library 2. DNA sequencing by synthesis -- varies greatly between techniques 3. Sequence alignment Sequencing methods Sanger sequencing Next generation sequencing Shotgun sequencing ------------------- ------------------------------------------------------------------------------------------------------------------ ------------------------------------------------------------------------------------------ --------------------------------------------------------------------------------------------------------------- Description Also known as chain termination method; uses dideoxynucleotides (ddNTPs) to stop DNA synthesis at specific bases High-throughput sequencing allowing millions of fragments to be sequenced simultaneously A method of randomly breaking up DNA sequences into small fragments for sequencing and then reassembling them Accuracy Very high Variable Moderate Throughput Low (up to 96 samples at a time without barcoding) Very high (up to billions of reads) High (can sequence entire genomes) Cost Moderate Low per base Moderate to high Applications Single gene sequencing, small projects Whole genomes, transcriptomes, metagenomics Whole genome sequencing, genome assembly Length of strands Typically, 100 to 1000 bp Can sequence thousands to millions of base pairs Can sequence entire genomes, typically hundreds of genes (\>1000 bp) **Describe how genomes are annotated and general features about the human genome** Annotation of genomes - Comparative genomics uses sequence homology to look at relationships within a family of genes - Genetics/biochemistry uses mutation analysis (i.e. remove gene or change its DNA sequence) to see alteration in function at the level of cell or organism to look at first member of a gene family General features about the human genome - Conserved regions -- critical genes are highly conserved and can be tracked from yeast to human  **Use your understanding of whole genome sequencing to describe some of its applications in research and medicine** Research Applications 1. Genomic Diversity Studies: Understanding genetic variation within and between populations. 2. Evolutionary Biology: Studying evolutionary relationships and phylogenetics among species. 3. Functional Genomics: Investigating gene functions and interactions. 4. Comparative Genomics: Comparing genomes of different species to identify conserved elements and evolutionary changes. 5. Microbial Genomics: Analysing microbial genomes for insights into pathogenicity, antibiotic resistance, and metabolic pathways. 6. Plant Genomics: Studying plant genomes for traits such as disease resistance and yield improvement. 7. Environmental Genomics: Assessing biodiversity and ecosystem functions through the sequencing of environmental samples. Medical Applications 1. Personalised Medicine: Tailoring treatments based on an individual\'s genomic information. 2. Cancer Genomics: Identifying mutations and alterations in cancer cells for targeted therapies. 3. Rare Disease Diagnosis: Providing insights into genetic disorders through the identification of pathogenic variants. 4. Pharmacogenomics: Understanding how genetic variations affect drug response and metabolism. 5. Infectious Disease Outbreak Tracking: Monitoring and identifying pathogens in outbreak scenarios. 6. Prenatal Screening: Assessing foetal genetic conditions through non-invasive prenatal testing. 7. Gene Therapy Development: Identifying suitable targets for gene therapy interventions. **Explain the biological role of transcription** - Synthesise an RNA molecule from a DNA template - Central dogma: DNA RNA protein - Transcription read in 3' to 5' direction while RNA is synthesised in a 5' to 3' direction **Describe the general structure and function of RNA polymerases**  Transcription in prokaryotes one RNA polymerase synthesises all different types of RNA\ Transcription in eukaryotes three separate RNA polymerases: RNA polymerase I, RNA polymerase II, RNA polymerase III A screenshot of a cell chart Description automatically generated 2 forms of prokaryotic RNA polymerase: - Core enzyme (composed of 5 subunits) - Holoenzyme (core plus the sigma-factor subunit) RNA polymerase subunit Function ------------------------ --------------------------------------------------------------------- 2 alpha subunits Help enzyme assembly, promote interactions with regulatory proteins Beta subunit Catalytic centre Beta prime Binds DNA Omega Enzyme assembly, regulation of gene expression Sigma 70 Binds -10 and -35 regions in promoter, helps separate DNA strands **Explain in detail the process of transcription in prokaryotes (including initiation, elongation and termination)**  Initiation 1. The RNA polymerase holoenzyme (core enzyme plus sigma factor) assembles 2. The sigma factor recognises the -35 and -10 regions of the promoter 3. One side of the sigma factor binds to the -35 and -10 regions of the promoter of DNA and the other side forms extensive interactions with the other subunits 4. DNA is unwound around the -10 region and one strand of it is fed into the channel in RNA polymerase that goes all the way through the molecule, and that incorporates the active site marked by the position of the Mg ion Elongation 5. Release of the sigma factor subunit from the polymerase allowing the core enzyme to proceed along the DNA template synthesising mRNA in the 5' to 3' direction 6. DNA is continuously unwound ahead of the core enzyme and rewound behind it Termination  7. Transcription proceeds until after the RNA polymerase transcribes a terminator sequence - Intrinsic terminators (do not require any other factors) - The RNA transcript of the intrinsic terminator sequence has a GC-rich inverse repeat sequence followed by a string of Us - The two inverse repeat sequences are complementary to each other - RNA anneals onto itself in this region, forming a very stable GC-rich hairpin loop - When the string of Us string is synthesized in the transcription bubble, it complements the string of A's on the template DNA - the two are held together by weak hydrogen bonds, this bonding is unstable - When RNA polymerase encounters an unstable strand pairing in the transcription bubble, it normally backtracks to stabilise it. But in this case the hairpin loop creates a 'roadblock' resulting in a release of RNA and dissociation of RNA polymerase from the DNA template - Rho dependent chain termination - The second type of termination mechanism requires the help of the Rho factor - The termination site in this case is preceded by an upstream Rut sequence (Rho utilization) - As RNA polymerase transcribes the Rut sequence, it creates the Rho binding site on RNA. - Rho binds to RNA - As RNA polymerase moves further along the DNA, the Rho factor then moves along the newly synthesised RNA strand, trying to catch up with RNA polymerase - Once RNA polymerase reaches the terminator sequence, it pauses, and Rho finally catches up - Rho is an ATP dependent motor that uses the energy of ATP hydrolysis to unwind the DNA-RNA transcription bubble resulting in the release of RNA and all protein components and termination of transcription  **Summarise the key features of the genetic code** - It is a triplet code -- codons -- consist of 3 consecutive nucleotides - 64 codons -- 61 specify amino acids and 3 specify stop codons - The code is non-overlapping - The code is degenerate because an amino acid can be coded for by multiple codons - The code is unambiguous - The code is read in 5' to 3' direction - The code is virtually/almost universal What is a Gene? Colinearity and Transcription Units \| Learn Science at Scitable **Explain what a reading frame is** 3 possible reading frames (in one direction) - Open reading frame start: initiation codon (AUG; encodes Met) - End point defined by termination codon (UAAA, UAG or UGA) The reading frame - Each reading frame gives a different sequence of codons - Only one reading frame encodes the correct protein - Other reading frames unlikely to encode proteins  **Discuss the implications of the code being degenerate** - Provides some degree of protection against mutations in the DNA sequence as changes to one or two nucleotides in a codon can still result in the same amino acid being incorporated into the protein - An amino acid can be specified by \> 1 codon **Explain how the various key structural features of tRNAs contribute to their function in translation** Cloverleaf Structure: - Description: tRNAs have a characteristic cloverleaf shape formed by several loops and stems. - Function: This structure helps maintain stability and allows tRNAs to fit properly into the ribosome during translation, facilitating interactions with mRNA and ribosomal components. Amino Acid Attachment Site: - Description: The 3\' end of tRNA has a specific site (CCA tail) where amino acids are covalently attached. - Function: This site allows tRNAs to carry specific amino acids to the ribosome, ensuring that the correct amino acid is incorporated into the growing polypeptide chain. Anticodon Loop: - Description: This loop contains the anticodon, a three-nucleotide sequence complementary to the mRNA codon. - Function: The anticodon ensures accurate pairing with the corresponding mRNA codon, facilitating the incorporation of the correct amino acid based on the mRNA sequence. Wobble Binding: - Description: The wobble hypothesis explains why multiple codons can code for a single amino acid. One tRNA molecule can recognise and bind to more than one codon due to less precise base pairs that arise between the 3^rd^ base of the codon and the base at the 1^st^ position on the anticodon. For example, a tRNA with the anticodon \"I\" (inosine) can pair with codons that have A, U, or C in the third position. The wobble hypothesis states that the base at the 5' end of the anticodon is not spatially confined as the other two bases allowing it to form hydrogen bonds with any several bases located at the 3' end of a codon. - Function: This flexibility allows a single tRNA to recognise multiple codons, which reduces the number of tRNA species needed and enhances the efficiency of translation by accommodating codon degeneracy. It allows faster dissociation of tRNA from mRNA and protein synthesis. It also minimises the damage that can be caused by a misreading of the code. Stability and Flexibility: - Description: tRNAs have stable double-stranded regions (stems) and flexible single-stranded regions (loops). - Function: The stability of the stems protects against degradation, while the flexibility allows tRNAs to adapt their shape for efficient interaction with the ribosome and mRNA during protein synthesis. Modified Nucleotides: - Description: tRNAs contain various modified nucleotides that enhance their function. - Function: These modifications can improve the stability of the tRNA structure, increase the accuracy of codon-anticodon pairing, and facilitate recognition by aminoacyl-tRNA synthetases (enzymes that attach amino acids to tRNAs). Variable Loop: - Description: This loop varies in length and composition among different tRNAs. - Function: It helps in the recognition of specific tRNA molecules by other cellular components, contributing to the specificity of translation.   tRNA synthetase - Function: Attach specific amino acids to corresponding tRNA molecules. - Aminoacylation: Catalyse the formation of aminoacyl-tRNA complexes. - Specificity: 20 distinct synthetases for each standard amino acid. - Proofreading: Correct errors in amino acid selection to ensure accuracy. - Role in Protein Synthesis: Essential for translating genetic code into proteins. - Clinical Relevance: Malfunctions can lead to diseases; potential antibiotic targets. - Requires: binding surface for the tRNA anticodon, active site editing i.e. the removal of incorrect amino acids, active site catalysing the covalent attachment of amino acids to tRNAs **Describe the key components of the translation machinery and their basic properties** **The molecular organisation of the ribosome including subunits** +-----------------------+-----------------------+-----------------------+ |  | **Prokaryote** | **Eukaryote** | | | | | |  | | | +=======================+=======================+=======================+ | **rRNA** | 70S | 80S | +-----------------------+-----------------------+-----------------------+ | **Large subunit** | 50S | 60s | +-----------------------+-----------------------+-----------------------+ | **Constituents** | 5S, 23S | 5s,28s, 5.8s | +-----------------------+-----------------------+-----------------------+ | **Function** | Ribozyme catalyses | Ribozyme catalyses | | | the formation of the | the formation of the | | | peptide bonds between | peptide bonds between | | | the amino acids in | the amino acids in | | | the 5' - 3' direction | the 5' - 3' direction | +-----------------------+-----------------------+-----------------------+ |  | | | +-----------------------+-----------------------+-----------------------+ | **Small subunit** | 30S | 40S | +-----------------------+-----------------------+-----------------------+ | **Constituents** | 16S | 18s | +-----------------------+-----------------------+-----------------------+ | **Function** | The 16S subunit binds | The 18s subunit binds | | | mRNA to the binding | mRNA to the binding | | | site of the small | site of the small | | | subunit by | subunit by binding to | | | identifying the shine | the 5' cap | | | Dalgarno sequence (8 | | | | bp) upstream of the | | | | start codon (+1) in | | | | prokaryotes | | +-----------------------+-----------------------+-----------------------+  **Identify the 4 stages of protein synthesis (translation)** 1. Activation of amino acids -- loading of amino acids or charging tRNA 2. Initiation 3. Elongation 4. Termination **Describe the mechanism of each stage (including the 4 steps of the elongation stage and the role of peptidyl transferase activity)** 1\. Activation of Amino Acids (Charging of tRNA) - Role of tRNA Synthetase: - Amino Acid Activation: Each amino acid is activated by a specific aminoacyl-tRNA synthetase. This enzyme catalyses the reaction using ATP to form an aminoacyl-AMP intermediate. - Charging of tRNA: The activated amino acid is then attached to its corresponding tRNA, forming aminoacyl-tRNA. This step ensures that the correct amino acid is paired with the appropriate tRNA. - Proofreading: Many tRNA synthetases have proofreading capabilities to ensure the accuracy of the charged tRNA, reducing the chance of errors during protein synthesis. 2\. Initiation - Formation of the Initiation Complex: - Small Ribosomal Subunit Binding: The small ribosomal subunit binds to the mRNA at the 5\' untranslated region (UTR) and scans for the start codon (AUG). - Initiator tRNA: The initiator tRNA, carrying methionine (in eukaryotes), recognises the start codon and binds to it in the P site of the ribosome. - Large Ribosomal Subunit Assembly: The large ribosomal subunit then joins the complex, forming a functional ribosome with the initiator tRNA positioned at the P site and the A site available for the next aminoacyl-tRNA. 3\. Elongation - Aminoacyl-tRNA Binding: - An aminoacyl-tRNA that corresponds to the next codon on the mRNA enters the A site of the ribosome. - Peptide Bond Formation: - The peptidyl transferase activity of the ribosome (primarily carried out by rRNA) catalyses the formation of a peptide bond between the amino acid in the A site and the growing polypeptide chain in the P site. This involves a nucleophilic attack by the amino group of the A site amino acid on the carbonyl carbon of the P site amino acid. - Translocation: - The ribosome moves down the mRNA by one codon. The tRNA in the A site, now carrying the growing polypeptide chain, shifts to the P site, and the empty tRNA in the P site moves to the E site. - Release of Empty tRNA: - The empty tRNA in the E site is released from the ribosome, making the A site available for the next aminoacyl-tRNA. 4\. Termination - Stop Codon Recognition: - When the ribosome encounters a stop codon (UAA, UAG, or UGA) on the mRNA, no corresponding tRNA can bind to the A site. - Binding of Release Factors: - Release factors (proteins) bind to the ribosome at the A site. These factors recognise the stop codon and trigger the termination process. - Polypeptide Release: - The peptidyl transferase activity catalyses the hydrolysis of the bond between the tRNA in the P site and the polypeptide chain, releasing the newly synthesised protein. - Ribosome Disassembly: - The ribosomal subunits disassociate, and the mRNA and tRNAs are released, allowing the ribosome to be recycled for another round of translation. 1. Activation of tRNAs  2. Initiation  Similarities of initiation in prokaryotes and eukaryotes: - eIF2 has the same role as IF2 in binding to charged initiator tRNA - Release of initiation factor(s) from the small ribosomal subunit is followed by assembly of the complete ribosome 3. Elongation tRNA binding 3.1 = binding of a new aminoacyl tRNA - The aminoacyl tRNA binds the ribosome in the A site as a complex with EF-Tu (GTP) - GTP hydrolysis releases EF-Tu and GDP -- stronger binding of the aminoacyl tRNA - Preferential dissociation of incorrect tRNA (i.e. not matching the codon - The ribosome has three sites: A (aminoacyl), P (peptidyl), and E (exit). - An aminoacyl-tRNA carrying the next amino acid to be added enters the A site - The anticodon of the tRNA pairs with the corresponding codon on the mRNA - The anticodon of tRNAs in some cases can read more than one codon specifying the same amino acid 3.2 = formation of the peptide bond - New peptide bond formed - Aminoacyl bond released - Requires peptidyl transferase activity of the ribosome, mediated by the 23S rRNA - The ribosome catalyses the formation of a peptide bond between the amino acid in the A site and the growing polypeptide chain attached to the tRNA in the P site - This reaction is facilitated by the ribosomal RNA in the large subunit, acting as a ribozyme  3.3 = translocation of the ribosome - The ribosome shifts in the 5\' to 3\' direction along the mRNA by one codon - The tRNA in the P site, now carrying the growing polypeptide, moves to the E site and is released - The tRNA in the A site moves to the P site, making room for a new aminoacyl-tRNA to enter the A site' 3.4 = release of deacetylated tRNA - The tRNA that has just exited the P site (now in the E site) is released from the ribosome - This process ensures that the ribosome is ready for the next aminoacyl-tRNA to bind to the A site, allowing the elongation cycle to continue 4. Termination of translation - Signalled by codons UAA, UAG and UGA - Recognised by protein release factors (RFs) - Causes hydrolysis of last peptidyl-tRNA bond - Releases polypeptide and tRNA - Causes ribosomal subunits to dissociate A diagram of a disease Description automatically generated **Understand how the reading frame is selected in prokaryotes and eukaryotes ** Prokaryotes 1. Shine-Dalgarno Sequence: - Prokaryotic ribosomes recognise the mRNA via the Shine-Dalgarno (SD) sequence, a ribosomal binding site located upstream of the start codon (AUG). - The SD sequence is complementary to a region of the 16S rRNA in the 30S ribosomal subunit, allowing for specific binding. 2. Initiation Complex Formation: - The formation of the initiation complex occurs when the small ribosomal subunit (30S) binds to the mRNA at the Shine-Dalgarno sequence, positioning the start codon (AUG) in the P site. - The first aminoacyl-tRNA (fMet-tRNA) then binds to the start codon. 3. Reading Frame Determination: - The reading frame is determined by the position of the start codon (AUG), which establishes the triplet sequence for translation. - As translation proceeds, the ribosome moves along the mRNA in the 5\' to 3\' direction, maintaining the reading frame established by the initial AUG. Eukaryotes 1. 5\' Cap Recognition: - Eukaryotic mRNAs are modified at the 5\' end with a 7-methylguanylate cap, which is recognised by the eukaryotic ribosome. - The ribosome assembles around the 5\' cap, scanning the mRNA for the start codon (AUG). 2. Scanning Mechanism: - The ribosome (40S subunit) scans the mRNA from the 5\' end until it finds the first AUG codon. - This scanning process allows the ribosome to identify the correct reading frame based on the location of the start codon. 3. Reading Frame Confirmation: - Once the start codon is recognised, the large subunit (60S) joins the complex, and translation begins. - Similar to prokaryotes, the established start codon sets the reading frame for the subsequent amino acids added during translation. Summary - Prokaryotes utilise the Shine-Dalgarno sequence for ribosomal binding and reading frame determination, focusing on the start codon following this sequence. - Eukaryotes rely on the 5\' cap and scanning for the start codon (AUG) to establish the reading frame. **Compare the following between prokaryotes and eukaryotes:** - **Describe how differences in the structure of ribosomes underpin the medical use of antibiotic** Ribosomal Structure Differences 1. Size: - Prokaryotic Ribosomes: Composed of 70S subunits, which include a 50S large subunit and a 30S small subunit. - Eukaryotic Ribosomes: Composed of 80S subunits, which include a 60S large subunit and a 40S small subunit. 2. rRNA and Protein Composition: - Prokaryotic ribosomes have different rRNA and protein components compared to eukaryotic ribosomes. - For example, the 16S rRNA in the small subunit of prokaryotic ribosomes is distinct from the 18S rRNA in eukaryotic ribosomes. 3. Binding Sites: - The arrangement and size of the binding sites for tRNA differ between prokaryotic and eukaryotic ribosomes, affecting how antibiotics can interact with them. Mechanism of Action of Antibiotics 1. Selective Targeting: - Antibiotics like tetracyclines, macrolides, and aminoglycosides target specific features of prokaryotic ribosomes. - For instance, tetracyclines bind to the 30S subunit and block the binding of aminoacyl-tRNA, inhibiting protein synthesis. 2. Inhibition of Translation: - Some antibiotics disrupt the elongation phase of translation. For example, an antibiotic that binds to the 50s subunit would most likely interfere with peptide bond formation. - This inhibition selectively affects bacterial protein synthesis without interfering with eukaryotic ribosomes, reducing potential side effects in human cells. 3. Resistance to Eukaryotic Ribosomes: - Because antibiotics are designed to specifically target prokaryotic ribosomes, they generally do not affect eukaryotic ribosomes due to structural differences. - This selective targeting minimises toxicity to human cells, making these antibiotics effective treatments for bacterial infections.  Part of ribosome Description Role in translation Effect of antibiotics ------------------------- -------------------------------------------------------------------------------- ------------------------------------------------------------------------------------------------------------------------------------------- ---------------------------------------------------------------------------------------------------------------------------- Large subunit Composed of 50S (prokaryotes) or 60S (eukaryotes) Catalyses peptide bond formation between amino acids (ribozyme activity); holds tRNAs in place during elongation. Antibiotics like macrolides bind here, blocking the exit tunnel and preventing peptide chain elongation. Small subunit Composed of 30S (prokaryotes) or 40S (eukaryotes) Binds to the mRNA and ensures correct codon-anticodon pairing; facilitates the initiation of translation. Aminoglycosides and tetracyclines bind to the 30S subunit, causing misreading of the mRNA or blocking tRNA binding. A site (Aminoacyl site) Site on the ribosome where new aminoacyl-tRNA binds. Accepts incoming tRNA carrying the next amino acid based on the codon in the mRNA. Antibiotics may block tRNA entry, inhibiting the addition of new amino acids. P site (Peptidyl site) Site on the ribosome where the growing polypeptide chain is held. Contains the tRNA with the polypeptide chain; catalyses the peptide bond formation with the amino acid in the A site. Some antibiotics prevent peptide bond formation, disrupting protein synthesis. E site (Exit site) Site on the ribosome where empty tRNA exits after transferring its amino acid. Releases the uncharged tRNA after it has donated its amino acid, making room for a new aminoacyl-tRNA. Certain antibiotics can affect tRNA release, preventing recycling of tRNA. mRNA binding site The region where mRNA attaches to the ribosome. Ensures the ribosome reads the mRNA sequence correctly during translation. Antibiotics that target the small subunit can disrupt mRNA binding, halting translation. Ribosomal RNA (rRNA) Structural and catalytic RNA components of the ribosome. Provides the framework for the ribosomal structure and catalyses peptide bond formation; plays a key role in the accuracy of translation. Antibiotics that interact with rRNA can impair ribosomal function and stability, leading to ineffective protein synthesis. - **Analyse differences in the process of translation initiation and in the organisation of polysomes** Translation initiation Prokaryotes Eukaryotes ------------------------- ---------------------------------------------------------- --------------------------------------------------------------------------------------------------- Initiation factors Fewer initiation factors involved (e.g., IF1, IF2, IF3) More complex initiation factors (e.g. eIFs) required for assembly and scanning. mRNA recognition Shine-Dalgarno sequence helps ribosome bind to mRNA. 5\' cap structure recognised by the small ribosomal subunit (40S) for binding. Start codon recognition Start codon (AUG) positioned by Shine Dalgarno sequence. Start codon (AUG) found by scanning the mRNA from the 5\' cap until the first AUG is encountered. Initiation tRNA Formylmethionine (fMet-tRNA) is used as the initiator. Methionine (Met-tRNA) is used as the initiator. Ribosome assembly 30S subunit binds first, then the 50S subunit joins. The 40S subunit binds to the mRNA first, then the 60S subunit joins. Polysome organisation Prokaryotes Eukaryotes --------------------------- ------------------------------------------------------------------------------------------------------------------ ------------------------------------------------------------------------------------------------------------------------------------------------------------------------------- Polysome structure Ribosomes can bind to mRNA in a linear arrangement, forming a polysome that can be more tightly packed. Polysomes form with ribosomes arranged in a circular configuration due to the interaction of the 5\' cap with the poly-A tail, allowing for efficient recycling of ribosomes. Functionality Ribosomes can begin translation on the same mRNA as soon as it is transcribed, enabling rapid protein synthesis. Multiple ribosomes translate a single mRNA simultaneously, with the circular structure facilitating continuous translation. Regulation of translation Less complex regulatory mechanisms; primarily relies on availability of ribosomes and mRNA. More intricate regulatory mechanisms involving various eukaryotic initiation factors (eIFs) and signalling pathways affecting translation efficiency. - **Contrast the spatial and temporal relationships between transcription and translation** - In prokaryotes, transcription and translation are simultaneous or coupled - In eukaryotes, transcription and translation are spatially separated - **Understand how co- and post-translational events affect the function and localisation of proteins** Folding -- the role of chaperones  Addressing to sub-cellular locationsA diagram of cell division Description automatically generated Chemical modifications -- a regulatory protein code Targeted degradation -- proteasome, ubiquitination A diagram of a cell membrane Description automatically generated  Topic 4 Define repressor, co-repressor, inducer and activator ----------------------------------------------------- **Repressor**: A repressor is a protein that binds to specific DNA sequences (usually near a gene) and inhibits the transcription of that gene. By blocking the RNA polymerase or other transcription factors, it prevents gene expression. **Co-repressor**: A co-repressor is a molecule that does not directly bind to DNA but works with a repressor to enhance its ability to inhibit gene expression. Co-repressors often modify the structure of chromatin or interact with the repressor to stabilise its binding to DNA. **Inducer**: An inducer is a molecule that increases gene expression by binding to a repressor and causing it to release from the DNA, or by binding to an activator to enhance its ability to promote transcription. Inducers are often involved in regulatory pathways that respond to environmental changes. **Activator**: An activator is a protein that binds to specific DNA sequences and enhances the transcription of a gene. By facilitating the recruitment of RNA polymerase and other necessary factors, activators promote gene expression. Recognise the role of allostericity in the function of repressor and activator proteins --------------------------------------------------------------------------------------- **Key Concepts:** - **Allosteric Regulation**: A process where the binding of a molecule at one site on a protein affects the activity or function of the protein at a different site. - **Allosteric proteins**: Proteins that exist in two conformational forms (different shapes