Structure_of_Proteins_2024.pdf
Document Details
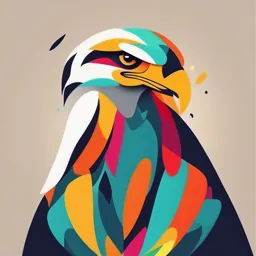
Uploaded by DecisiveMorningGlory
2024
Tags
Full Transcript
BCCB2000 Foundations of Biochemistry Protein Structure Ricardo L. Mancera 1 WARNING This material has been reproduced and communicated to you by or on behalf of Curtin University in accordance with section 113P of the Copyright Act 1968 (the Act) The material in this communication may be subject to...
BCCB2000 Foundations of Biochemistry Protein Structure Ricardo L. Mancera 1 WARNING This material has been reproduced and communicated to you by or on behalf of Curtin University in accordance with section 113P of the Copyright Act 1968 (the Act) The material in this communication may be subject to copyright under the Act. Any further reproduction or communication of this material by you may be the subject of copyright protection under the Act. Do not remove this notice. 2 1 Learning objectives When you complete this lecture you will be able to Understand and identify key concepts in protein structure: Peptide bonds Primary, secondary, tertiary and quaternary structure Recognise the different ways in which protein structure can be visualised Appreciate the importance of proteins Recognise and describe the many roles and functions of proteins Understand key concepts in protein folding Appreciate the role of mutations in disease Understand and describe the principal characteristics of proteins 3 Primary structure: the ‘backbone’ of proteins A peptide is comprised of at least three amino acids linked by two peptide bonds Peptide < 50 amino acids; polypeptide or protein > 50 amino acids in a linear sequence Amino acids in proteins are called residues C O O O H3N O CH + C H3N C O H3N O CH + CH R3 R2 R1 H 2O O R1 Amino or N-terminus H N CH H3N C O R3 C CH R2 O CH N H C O Carboxy or C-terminus -carboxyl and -amino groups when combined in a peptide bond lose their charge 4 2 Primary structure of proteins The bonds either side of the -carbon can rotate and are defined by phi () and psi () angles The peptide/protein chain backbone can thus bend or fold to form compact structures phi () and psi (ψ) angles on either side of the -carbon The peptide bond is rigid and planar R groups most often alternate on either side of the ‘backbone’ Nelson & Cox, 2000 5 The peptide bond has partial double-bond like character As an amide bond it is stabilised by electron resonance Shorter than a single bond and fairly rigid and planar Polar (has a dipole) and so can form hydrogen bonds Many C=O and N-H groups from the protein backbone form hydrogen bonds that help to stabilise the final folded conformation of a protein partial negative charge on carbonyl oxygen electric dipole resonance structure partial positive charge on amide nitrogen resonance structure 6 3 R groups in peptides most often alternate on either side of the backbone Peptide bonds are usually in a trans configuration due to steric constraints in the positioning of successive R groups in the peptide chain Cis configuration can occur (infrequent) with proline due to the cyclic nature of its side chain 7 Secondary structure of proteins Regular arrangements of the backbone of amino acids located next to each other in 3D space Internal protein structures and patterns can be seen in this molecular representation of a protein (carboxypeptidase A, PDB code 3CPA): Helices (pink coils) Beta strands (yellow ribbons) Beta sheet (yellow ribbons next to each other) Turns (blue thin ribbon) 8 4 The -helix Each helical turn has 3.6 amino acids Hydrogen bonds stabilise the structure Occur between backbone amide (N-H) and carbonyl C=O) Oriented parallel to the axis of the helix Join residue n to residue n+4 in the chain Ferrier 2014 9 The -helix There is no "hole" in the centre of a -helix! Side view Top view R-groups point ‘outwards’ 5Å Hydrogen bonding shown by dotted lines 3.6 residues per turn This corresponds to 10 to 11 atoms if counting only the main backbone atoms of N, C and C in a residue 10 5 The -helix The helical content of proteins differs markedly 11 β-strands and β-sheets β-strand: 5-10 consecutive amino acids in an almost fully extended conformation β-sheet: regular geometric arrangement of two or more β-strands close to each other in 3D space β-strands interact with each other via hydrogen bonds to form β-sheets Hydrogen bonds occur between backbone atoms of strands (N-H of amide and C=O of carbonyl group) Anti-parallel or parallel arrangement of β-strands Harvey & Ferrier (2011) 12 6 β-turns ‘Irregular’ geometry of structures in the polypeptide backbone Point where the polypeptide turns and changes direction Hairpin turns where the chain abruptly changes direction Reverse turns where the chain reverses direction Connects anti-parallel and parallel strands of a βsheet 13 Other turns and loops Flexible parts of proteins that connect -helices and β-strands. Usually found on the surface of proteins and in the N- and C-termini 14 7 Supersecondary structure Structural ‘motifs’ combining -helices and β-sheets Lippscott, Williams and Wilkins (2005) 15 Tertiary structure of proteins Final, active, 3D shape of a folded protein: native structure (native conformation) For example, myoglobin is a water-soluble protein and a good example of the tertiary structure of a so-called globular protein Water-soluble proteins fold into compact tertiary structures with nonpolar (hydrophobic) cores The hydrophobic force is one of the key driving forces of protein folding Space-filled representation of myoglobin Polar side chains Non-polar side chains Prosthetic group (in this case it is heme) 50% cross-section through myoglobin 16 8 Tertiary structure of proteins Human serum albumin has a molecular weight of 64,500 D and has 585 residues Comparison of β-extended and -helical conformations with its native structure reveals how formation of tertiary structure allows proteins to be very compact 17 Disulfide bonds and tertiary structure Covalent bonds between cysteine residues provide some rigidity to the tertiary structure of proteins Many but not all proteins have one or more disulfide (cystine) bonds Proteins may have either or both intraor inter-molecular disulfide (cystine) bonds S -S Intramolecular Lys-Glu-Thr-Cys-Ala-Lys-Phe-Tyr-Cys-Ala-Tyr N-terminus (free -amino) Intermolecular C-terminus (free -carboxyl) Lys-Glu-Thr-Ala-Ala-Lys-Phe-Tyr-Cys-Ala-Tyr S N-termini C-termini S Ile-Ala-Tyr-Cys-Phe-Val-Glu-Lys Ferrier 2014 18 9 Structural domains in proteins Domains are the fundamental 3D structural units of polypeptides Usually ‘built’ from combinations of secondary structures and supersecondary structural elements (motifs) Each domain is usually structurally independent of the other domains 19 A multi-domain (‘mosaic’) protein Domain architecture of a fragment of hematopoetic cell kinase (PDB coded 1QCF) Structure contains protein kinase (PK) and src homology 2 and 3 (SH2 and SH3) domains Ponting and Russell 2002 20 10 Quaternary structure Association of two or more protein chains (subunits) into a large, relatively permanent structure Subunits interact and ‘bind’ mainly through non-covalent interactions Subunits may be the same (homopolypeptides) or slightly different (heteropolypeptides) The quaternary structure may, or may not, be the active biological molecule Haemoglobin has four subunits and is the active structure Insulin is stored in a complex of six subunits but the monomer is the active structure Ferrier 2014 21 Prosthetic groups in proteins Some small organic and inorganic ions can be tightly associated with proteins and be essential to function Cu, Fe, Zn Haem in haemoglobin or myoglobin This is one chain (subunit of haemoglobin Ferrier 2014 22 11 Hierarchy (levels) of protein structure Secondary structure Supersecondary structure Domain structure Nelson and Cox (2005) Ferrier 2014 23 Molecular visualisation of proteins Experimental data: X-ray diffraction (XRD) crystallography, nuclear magnetic resonance (NMR) spectroscopy, molecular models 3D data: atomic coordinates (x,y,z) Deposited in the Research Collaborative for Structural Bioinformatics (RCSB) Protein Data Bank (PDB): www.rcsb.org Molecular visualisation programs: Swiss-PDBViewer, VMD, Pymol Myoglobin (Wikipedia) 24 12 Visualisation of the secondary structure The backbone of a protein can be traced using the sequence of carbons, carbonyl (C=O) carbons and/or amide (NH) nitrogens This allows to appreciate the overall “shape” of the protein (e.g. wireframe and ribbon traces of backbone in molecular visualisation programs) Trace of the -carbons in the polypeptide backbone of a protein 25 Styles of visualisation of proteins Stick Ball and Stick Space-fill Opaque Plastic Metal Cartoon / ribbon 26 13 The importance of proteins Proteins are amazing molecular machines with great diversity The constellation of all proteins in a cell is called its proteome Unravelling the proteome is the current challenge! It is important to characterise protein structure and interactions in order to understand the molecular basis of life as well as normal and disease states 27 The importance of proteins Genes receive much attention, but it is proteins that perform most life functions and even make up the majority of cellular structures This is because proteins are structurally diverse and versatile and, consequently, have many important roles Unlike the relatively unchanging genome, the dynamic proteome changes from minute to minute in response to tens of thousands of intra- and extra-cellular environmental signals 28 14 Protein structure and function determines Protein sequence determines Protein structure secondary supersecondary domain tertiary quaternary Protein function catalysis transport storage motion structural support immunity immune response to foreign matter growth and development communicating sensing regulation other ‘special purposes’ 29 Biological functions of proteins Catalysis break and form covalent bonds transform one chemical into another e.g. hexokinase and many different enzymes Transport carry small molecules or ions e.g. haemoglobin carries oxygen Storage store small molecules or ions e.g. ferritin stores iron (Fe) source of amino acids e.g. casein Motion generate movement in cells and tissues e.g. myosin in muscle Structural support structural support to cells and tissues e.g. collagen in tendons and ligaments, tubulin in cells Immunity immune response to foreign molecules (antigens) e.g. antibodies Growth direct growth and development e.g. growth hormones Communication transmit and send ‘messages’ within cells or between cells and tissues e.g. hormones and growth factors (between cells) Regulation switch genes ‘on’ or ‘off’ e.g. transcription factors Other ‘special purposes’ many other specific uses e.g. green fluorescent protein in jellyfish 30 15 Biotechnological applications of proteins Therapeutics (drugs) – Insulin for diabetes (> $15 billion annually) – Factor VIII for haemophilia – Herceptin for cancer Industry – Food supplements flavour and appearance of food brewing and cheese making Part of the biotechnology industry – components of washing powder – reagents for biological/biochemical assays Diagnostic markers – 1-antitrypsin for cancer – Insulin for diabetes Diagnostic analysis – Reagents in ELISA and other diagnostics 31 Protein folding The primary structure determines the tertiary structure of a protein Numerous interactions between side chains (including those far apart in the sequence), and between side chains and water, guide the overall folding (not just secondary structure) of the polypeptide chain to form compact structures These interactions include hydrogen bonds, hydrophobic interactions, ionic interactions and disulphide bonds Ferrier 2014 32 16 Protein folding Proteins fold into a conformation of lowest (free) energy (native state) The final folded conformation (structure) adopted by any polypeptide chain is generally the one in which the (free) energy is at its lowest During protein folding all molecular forces operate in concert and are optimised Hydrophobic interactions Electrostatic interactions Hydrogen bonding Dispersion interactions 33 Protein folding Protein folding occurs in milliseconds to minutes in the cell, using shortcuts through the maze of all the folding possibilities Short- and long-range interactions between side chains of amino acids dictate the folding process hydrophobic forces also drive the folding process Chaperone proteins (also called “heat shock” proteins) are required for the correct folding of many proteins in vivo Levinthal’s paradox: there is an enormous difference between the calculated and observed folding times of proteins! For a small protein of 100 amino acids, it can be estimated that there would be at least 10200 different conformations If the time required for the interconversion between conformations is about 10-13 seconds, it would require about 10193 years of random searching to sample all conformational space for the 100 amino acid protein! Ferrier 2014 34 17 Many proteins are only marginally stable For example, the thermal stability of collagen, like most proteins, is low Source Melting temp ˚C Body temp ˚C calf skin 39 37 shark skin 29 24‐28 cod skin 16 10‐14 Protein denaturation results in the unfolding and disorganisation of secondary and tertiary structure Denaturation can be caused by changes in temperature and/or pH, mechanical mixing, detergents, ions and heavy metals, solvents (e.g. alcohol), etc. 35 Protein misfolding and aggregation Protein folding is a highly complex process that can sometimes result in improperly folded proteins Misfolded proteins are usually “tagged” and degraded within the cell. However, sometimes misfolded proteins can accumulate, particularly as individuals age Amyloidoses (such as Alzheimer’s disease) and prion diseases are examples of cytotoxic protein aggregation events Ferrier 2014 36 18 A single amino acid change can lead to disease Sickle cell haemoglobin Glu6 in the β-chain is mutated to Val This allows the deoxygenated form of haemoglobin to stick to itself and form fibres 3D structure of a fibre of sickle cell haemoglobin 3D structure of haemoglobin http://pdb101.rcsb.org/motm/41 37 A single amino acid change can lead to disease Mutations in collagen (insoluble fibrous protein) or collagen-processing enzymes Lethal form of osteogenesis imperfecta in which fractures appear in utero, as revealed by this radiograph of a stillborn foetus Stetchy skin of Ehlers-Danlos syndrome, which is caused by deficiency of collagenprocessing enzymes 38 19 Summary of characteristics of proteins Proteins are the ‘building blocks’ of cells Proteins perform most of the functions in the cell the ‘workhorse’ of the cell Proteins comprise most of the dry mass of a cell an indication of their importance Linear polymers (‘chains’) of monomer amino acids are joined by peptide bonds amino acids are the ‘building blocks’ of proteins Amino acids are called ‘residues’ when connected together in a protein Proteins are also called polypeptides Proteins are highly specific act through 3D stereospecific interactions specificity is important because proteins have diverse functions due to the many ‘peptide’ bonds in the linear chain of the protein A protein chain has a linear direction from an amino end known as the ‘N terminus’ to the carboxyl end known as the ‘Cterminus’ 39 Summary of characteristics of proteins The linear sequence of amino acids specifies the complete 3D structure of a protein The 3D protein structure determines its function The linear protein chain spontaneously folds into its final 3D structure The folded 3D structure is the ‘native conformation’ and usually has low energy compared with other conformations The final 3D folded state of a protein can be unfolded, or denatured, by various conditions high temperature, pH, detergents or solvents (may be reversible) Protein structure has a hierarchy Protein chains fold into geometric structures Primary, secondary, supersecondary, domains, tertiary and quaternary e.g. -helices and β-sheets. Proteins can be comprised of domains one domain or more than one domain domains are independently folded structures (within the tertiary structure) that can be found in two or more proteins proteins with more than one domain are called ‘mosaic’ proteins 40 20 Summary of characteristics of proteins Proteins can be classified into families Proteins can generally be described as ‘globular’ or ‘fibrous’ most proteins have a ‘globular’ or compact shape even though they may be large and complicated some proteins are fibrous and elongated each member of the family has an amino acid sequence and 3D structure which resemble each other Proteins are dynamic and not static structures proteins can change their conformation (shape) to respond to an environmental stimulus or to execute their function Proteins can interact with each other this is the essence of how proteins can achieve so much in the cell A complex network of protein-protein interactions essentially defines what a cell is and what a cell does! e.g. collagen, -keratin, and elastin Proteins have a ‘native’ state and a denatured or unfolded state 41 Summary of characteristics of proteins Proteins contain a wide range of chemical functional groups Prosthetic groups are sometimes critical for protein function e.g. acids, amines, bases, and thiols prosthetic groups are organic molecules (other than amino acids) or inorganic ions covalently bound to a protein that ‘help’ a protein execute its function Proteins can act as pH buffers proteins have many ionisable functional groups (contributed by the side chains) that can act as buffers 42 21 Key concept map for protein structure The unit BIOL3011 Biomolecular Structure and Function delves deeper into these concepts and how protein structure and interactions are characterised through experimental and molecular modelling methods 43 Further reading Protein Structure (online course from Western Oregon University): https://wou.edu/chemistry/courses/online-chemistry-textbooks/ch450-and-ch451biochemistry-defining-life-at-the-molecular-level/chapter-2-protein-structure/ Ponting, C. P., & Russell, R. R. (2002). The natural history of protein domains. Annual Review of Biophysics and Biomolecular Structure, 31(1), 45-71. https://www.annualreviews.org/doi/abs/10.1146/annurev.biophys.31.082901.13431 4 PDB 101 at RSCB: https://pdb101.rcsb.org/ Proteopedia: Life in 3D: http://www.proteopedia.org/wiki/index.php/Main_Page Molecular visualisation website: First Glance in Jmol: http://bioinformatics.org/firstglance/fgij/ 44 22