Selection of Recombinants Lecture Notes PDF
Document Details
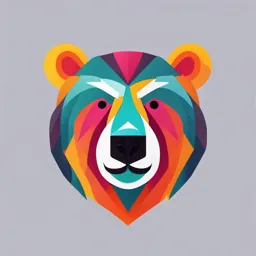
Uploaded by RespectableNobility892
DY Patil University
Dr. Navami Pai
Tags
Summary
This document is a lecture on the selection of recombinants. It covers various methods, including colony hybridization, plaque hybridization, western blotting, immunological screening, blue-white screening, and insertional inactivation. These methods are crucial for identifying and characterizing recombinants in molecular biology.
Full Transcript
Selection of recombinants Lecture by: Dr. Navami Pai Screening: The essential steps for colony hybridization are described as follows: (i) The initial step in screening a library—a colony of host cells are plated onto solid medium containing antibiotics. The presence of...
Selection of recombinants Lecture by: Dr. Navami Pai Screening: The essential steps for colony hybridization are described as follows: (i) The initial step in screening a library—a colony of host cells are plated onto solid medium containing antibiotics. The presence of antibiotics in the medium ensures growth of only transformed cells due to its antibiotic resistance gene in their plasmid. (ii) When a discrete colony is formed on the plate, it is then transferred onto nitrocellulose or nylon membrane commonly referred as solid matrix. The exact position of cell colony on the plate is maintained on the matrix. (iii) Once the cells are attached to solid matrix (nitrocellulose paper) cells are lysed, deproteinised and released DNA is denatured by alkaline reagent. (iv) The labelled DNA probe is mixed in the next step, to facilitate hybridization between target DNA and DNA probe. The unbound probe is washed off from the matrix. (v) Matrix is then subjected to autoradiography to determine the location of cells containing hybridized DNA. (vi) Development of dark spot on the X-ray film indicates the presence of target DNA (gene). The dark spot on X-ray film corresponds to the cell colony on the master plate. Once transformed cell colony is identified, it is then sub-cultured and maintained as it carries cloned DNA of interest. Screening by Colony Hybridization: Principally this screening technique involves hybridization between labelled DNA probe and a target DNA sequence. Therefore, a target gene sequence can be accurately identified. Plaque Hybridization: A genomic library is screened by infecting phage viruses containing inserted DNA on a lawn of bacteria. The steps are described as follows: (i) A lawn of bacterial cultures is initiated on the agar plate. (ii) Several thousands of phage particles containing inserted DNA are spread on the lawn of bacteria. (iii) Phage infects bacteria and plaques are obtained. These are then transferred to nitrocellulose paper; DNA is denatured to single strand and bound firmly onto solid matrix. (iv) 32P labelled DNA/RNA probe is added to ensure hybridization. (v) The location of the bound probe is determined by hybridization. (vi) The position of spot on the X-ray film corresponds to original site on agar plate. Once the desired gene is identified, it is then isolated and sub-cultured. Western Blotting To determine specific proteins of interest Immunological Screening: This is another novel method of screening a gene library. This method can be used as an alternative to DNA probe. The cells are allowed to transcribe and translate and the presence of protein can be identified by corresponding antibody molecule. The technique is described as follows: (i) Discrete colonies are formed on the master plate, (ii) Transfer sample of cells from each colony to nitrocellulose or nylon membrane. (iii) Allow the cells to transcribe and translate. The cells are then lysed and proteins are bound to matrix. (iv) Initially primary antibody treatment is done to faciliate the binding of primary antibody to protein molecule bound on the solid matrix. (v) Add secondary antibody that binds only to the primary antibody. Colorimetric reaction takes place only if the secondary antibody is present (Enzyme bound secondary antibody develops coloured compound when treated with respective substrate). (vi) A colony on the master plate that corresponds to a positive response on the matrix is identified. The cells from the positive colony on the master plate are sub-cultured as this may carry insert DNA that encodes the protein that bind primary antibody Screening based on gene expression- Blue-White Screening IDENTIFICATION OF RECOMBINANT BACTERIA Blue-white screening is a rapid and efficient technique for the identification of recombinant bacteria. It relies on the activity of β-galactosidase, an enzyme occurring in E. coli, which cleaves lactose into glucose and galactose. DISRUPTING THE LACZ GENE The presence of lactose in the surrounding environment triggers the lacZ operon in E. coli. The operon activity results in the production of β-galactoisdase enzyme that metabolizes the lactose. Most plasmid vectors carry a short segment of lacZ gene that contains coding information for the first 146 amino acids of β- galactosisdase. The host E. coli strains used are competent cells containing lacZΔM15 deletion mutation (N-terminal). When the plasmid vector is taken up by such cells, due to α- complementation process, a functional β-galatosidase enzyme is produced. The plasmid vectors used in cloning are manipulated in such a way that this α-complementation process serves as a marker for recombination. A multiple cloning site (MCS) is present within the lacZ sequence in the plasmid vector. This sequence can be nicked by restriction enzymes to insert the foreign DNA. When a plasmid vector containing foreign DNA is taken up by the host E. coli, the α-complementation does not occur, therefore, a functional β-galactosidase enzyme is not produced. If the foreign DNA is not inserted into the vector or if it is inserted at a location other than MCS, the lacZ gene in the plasmid vector complements the lacZ deletion mutation in the host E. coli producing a functional enzyme. HOW DOES BLUE WHITE SCREENING WORK? For screening the clones containing recombinant DNA, a chromogenic substrate known as X-gal is added to the agar plate. If β-galactosidase is produced, X-gal is hydrolyzed to form 5- bromo-4-chloro-indoxyl, which spontaneously dimerizes to produce an insoluble blue pigment called 5,5’-dibromo-4,4’- dichloro-indigo. The colonies formed by non-recombinant cells, therefore appear blue in color while the recombinant ones appear white. The desired recombinant colonies can be easily picked and Figure 1.A schematic representation of a typical plasmid vector that can be used for blue-white screening. Figure 2.A schematic representation of a typical blue-white screening procedure. Screening based on gene expression- Replica plating technique A technique in which the pattern of colonies growing on a culture plate is copied. A sterile filter plate is pressed against the culture plate and then lifted. Then the filter is pressed against a second sterile culture plate. This results in the new plate being infected with cell in the same relative positions as the colonies in the original plate. Usually, the medium used in the second plate will differ from that used in the first. It may include an antibiotic or without a growth factor. In this way, transformed cells can be selected. Screening using auxotrophs- Use of histidine auxotrophs as host Auxotrophs are organisms which do not grow on a minimal medium. They require specific nutritional requirement. When cloning vector carrying gene of interest along with his+ gene is used to transform his-colonies, the cells which take up the vector are transformants as his+ gene from the cloning vector will complement the his- host. Hence, the his- cells will no more be an auxotroph after acquiring the cloning vector. Selection by alpha complementation E. coli is a model bacterium used in the lab for molecular biology. And it has an enzyme called beta-galactosidase that helps E. coli break down sugars like lactose. Interestingly, the functional beta-galactosidase enzyme, capable of breaking down lactose and its analogs like X-gal, is formed by the tetramerization of four identical monomers of beta-galactosidase polypeptides. Tetramerization – it means is that the four monomers (small molecules that can bond together) bond with each other to form a tetramer (a larger molecule made up of four small molecules, or monomers). identical monomers or beta-galactosidase polypeptides as well as the complete tetramer. Within each beta-galactosidase enzyme, there are sequences of amino acids. When amino acid residues 11-41 are removed from the amino-terminus, the monomers cannot form the tetramer (tetramerize). To get technical, the peptide missing these residues is called the omega peptide (ω). Without the monomers being able to tetramerize, the complete enzyme cannot form, and therefore E. coli cannot break down x-gal. beta-galactosidase identical monomers missing amino acid residues 11-41 (ω-peptides). The result is no tetramerization, and therefore no complete beta-galactosidase enzyme is formed. Beta-galactosidase is a functional enzyme when it is composed of the joined four identical beta-galactosidase polypeptides. This is what is called a homotetramer (same/four). When working with this concept, researchers manipulate the sequences of these peptides to form an alpha (α) peptide and an omega (ω) peptide. The ω-peptide is the beta-galactosidase polypeptide, but as discussed, it is missing the amino acid residues 11-41 Scientists call this specific modification the lacZΔM15 mutation. Because of this mutation, the ω-peptides cannot form a homotetramer, which means it is not functional. This mutation is present in the genomes of E. coli cloning strains. Meanwhile, there is another side of this whole equation – that missing piece that would allow the monomer to form a homotetramer. This is the α-peptide, a small sequence with amino acid residues 1-59, which complements the missing amino acids in the ω-peptide. Once fully formed, the complete beta-galactosidase enzyme can break down x-gal. alpha-complementation with the ω-peptide and the α -peptide. Summary In panel A, you’ll see the identical monomers represented. When they come together, forming the homotetramer, the result is a functional beta- galactosidase enzyme that can break down x-gal. In panel B, we’re just looking at the polypeptides with the missing amino acid residues (11 – 41). Because of this missing part, beta-galactosidase polypeptides cannot tetramerize, and no enzymatic activity happens. Finally, in panel C, we have the ω-peptide with the missing amino acid residues and the α-peptide with amino acid residues 1-59. The result is alpha-complementation – like putting our treasure map halves together, leading to functional monomers that can tetramerize to form a functional beta-galactosidase enzyme. Alpha complementation for screening of recombinants Now we can take a look at this process within a host cell (usually E. coli cloning strains) and a plasmid that works as a vector carrying genetic material into the host cell. Figure 6 shows the sequence encoding the α-peptide (pink) and the sequence encoding the ω-peptide. The sequence encoding the α- peptide is found within the plasmid while the sequence encoding the ω-peptide is within the E. coli genome. Once the plasmid containing the α-peptide is within the E. coli cell, alpha-complementation can take place Insertional Inactivation (Refer earlier example) Antibiotic resistance as a marker An antibiotic resistance marker is a gene that produces a protein that provides cells with resistance to an antibiotic. Bacteria with transformed DNA can be identified by growing on a medium containing an antibiotic. Recombinants will grow on these medium as they contain genes encoding resistance to antibiotics such as ampicillin, chloro amphenicol, tetracycline or kanamycin, etc., while others may not be able to grow in these media, hence it is considered useful selectable marker. Antibiotic resistance in combination with blue white screening A cloning vector carrying antibiotic resistance gene can be used as a selectable marker for screening recombinants. As lac Z gene carries MCS, it is used a a site for insertion of gene of interest (GOI). If GOI is inserted at proper site and in turn is taken up by an antibiotic sensitive host, the transformants will turn antibiotic resistant and will have non-functional lac Z. Thus, transformants will show white colonies and non-transformants will show blue colonies. Screening using Restriction enzyme cleavage patterns (RFLP) Restriction fragment length polymorphism (RFLP) is a technique invented in 1984 by the English scientist Alec Jeffreys during research into hereditary diseases. It is used for the analysis of unique patterns in DNA fragments in order to genetically differentiate between organisms – these patterns are called Variable Number of Tandem Repeats (VNTRs). Genetic polymorphism is defined as the inherited genetic differences among individuals in over 1% of normal population. The RFLP technique exploits these differences in DNA sequences to recognize and study both intraspecies and interspecies variation. Most RFLP markers are co-dominant (both alleles in heterozygous sample will be detected) and highly locus-specific. An RFLP probe is a labeled DNA sequence that hybridizes with one or more fragments of the digested DNA sample after they were separated by gel electrophoresis, thus revealing a unique blotting pattern characteristic to a specific genotype at a specific locus. Short, single- or low-copy genomic DNA or cDNA clones are typically used as RFLP probes. The RFLP probes are frequently used in genome mapping and in variation analysis (genotyping, forensics, paternity tests, hereditary disease diagnostics) How it works Eg: Detection of Sickle cell anemia by RFLP A mutation associated with a genetic disease may cause the loss or addition of a restriction site either within the gene or in a flanking region. A small number of RFLPs are associated with genes known to cause diseases, as the following example about sickle-cell anemia illustrates. In sickle-cell anemia (SCA), a single base-pair change in the gene for the β–globin polypeptide of hemoglobin results in an abnormal form of hemoglobin, Hb-S, instead of the normal Hb-A. Hb-S molecules associate abnormally, leading to sickling of the red blood cells, tissue damage, and possibly death. The sickle-cell mutation changes an A–T base pair to a T–A base pair so that the sixth codon for β-globin is changed from GAG to GUG. As a result of this SNP allele, valine is inserted into the polypeptide instead of glutamic acid. THANK YOU