Robbins & Cotran Pathologic Basis of Disease, Ninth Edition (2015) PDF
Document Details
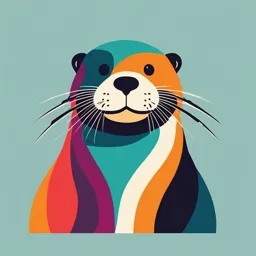
Uploaded by BountifulJadeite4342
LECOM MMS Program
2015
Tags
Summary
This document is an excerpt from "Robbins & Cotran Pathologic Basis of Disease, Ninth Edition (2015)". It provides a general overview of inflammation and repair, including systemic effects, tissue repair processes, and mechanisms of wound healing.
Full Transcript
100 CHAPTER 3 Inflammation and Repair as disseminated intravascular coagulation, hypotensive shock, and metabolic disturbances including insulin resistance and hyperglycemia. This clinical triad is known as septic shock; it is discussed in more detail in Chapter 4. NORMAL KEY CONCEPTS Systemic...
100 CHAPTER 3 Inflammation and Repair as disseminated intravascular coagulation, hypotensive shock, and metabolic disturbances including insulin resistance and hyperglycemia. This clinical triad is known as septic shock; it is discussed in more detail in Chapter 4. NORMAL KEY CONCEPTS Systemic Effects of Inflammation ■ ■ ■ ■ Fever: cytokines (TNF, IL-1) stimulate production of prostaglandins in hypothalamus Production of acute-phase proteins: C-reactive protein, others; synthesis stimulated by cytokines (IL-6, others) acting on liver cells Leukocytosis: cytokines (colony-stimulating factors) stimulate production of leukocytes from precursors in the bone marrow In some severe infections, septic shock: fall in blood pressure, disseminated intravascular coagulation, metabolic abnormalities; induced by high levels of TNF and other cytokines Excessive inflammation is the underlying cause of many human diseases, described throughout this book. Con versely, defective inflammation is responsible for increased susceptibility to infections. The most common cause of defective inflammation is leukocyte deficiency resulting from replacement of the bone marrow by leukemias and metastatic tumors, and suppression of the marrow by ther apies for cancer and graft rejection. Inherited genetic abnormalities of leukocyte adhesion and microbicidal function are rare but very informative; these are described in Chapter 6, in the context of immunodeficiency diseases. Deficiencies of the complement system are mentioned earlier and are described further in Chapter 6. We next consider the process of repair, which is a healing response to tissue destruction caused by inflammatory or non-inflammatory causes. Repair, sometimes called healing, refers to the restoration of tissue architecture and function after an injury. (By convention, the term repair is often used for parenchy mal and connective tissues and healing for surface epithelia, but these distinctions are not based on biology and we use the terms interchangeably.) Critical to the survival of an organism is the ability to repair the damage caused by toxic insults and inflammation. Hence, the inflammatory response to microbes and injured tissues not only serves to eliminate these dangers but also sets into motion the process of repair. Repair of damaged tissues occurs by two types of reactions: regeneration by proliferation of residual (uninjured) cells and maturation of tissue stem cells, and the deposition of connective tissue to form a scar (Fig. 3-24). • Regeneration. Some tissues are able to replace the damaged components and essentially return to a normal state; this process is called regeneration. Regeneration Severe injury REGENERATION SCAR FORMATION Figure 3-24 Mechanisms of tissue repair: regeneration and scar formation. Following mild injury, which damages the epithelium but not the underlying tissue, resolution occurs by regeneration, but after more severe injury with damage to the connective tissue, repair is by scar formation. Tissue Repair Overview of Tissue Repair Mild, superficial injury • occurs by proliferation of cells that survive the injury and retain the capacity to proliferate, for example, in the rapidly dividing epithelia of the skin and intestines, and in some parenchymal organs, notably the liver. In other cases, tissue stem cells may contribute to the restoration of damaged tissues. However, mammals have a limited capacity to regenerate damaged tissues and organs, and only some components of most tissues are able to fully restore themselves. Connective tissue deposition (scar formation). If the injured tissues are incapable of complete restitution, or if the supporting structures of the tissue are severely damaged, repair occurs by the laying down of connec tive (fibrous) tissue, a process that may result in scar formation. Although the fibrous scar is not normal, it provides enough structural stability that the injured tissue is usually able to function. The term fibrosis is most often used to describe the extensive deposition of collagen that occurs in the lungs, liver, kidney, and other organs as a consequence of chronic inflamma tion, or in the myocardium after extensive ischemic necrosis (infarction). If fibrosis develops in a tissue space occupied by an inflammatory exudate, it is called organization (as in organizing pneumonia affect ing the lung). After many common types of injury, both regeneration and scar formation contribute in varying degrees to the ultimate repair. Both processes involve the proliferation of various cells, and close interactions between cells and the extracellular matrix (ECM). We first discuss the general Tissue repair capacity may exist in these tissues, it is insufficient to produce tissue regeneration after injury. Skeletal muscle is usually classified as a permanent tissue, but satellite cells attached to the endomysial sheath provide some regenerative capacity for muscle. In permanent tissues, repair is typically dominated by scar formation. mechanisms of cellular proliferation and regeneration, and then the salient features of regeneration and healing by scar formation, and conclude with a description of cutane ous wound healing and fibrosis (scarring) in parenchymal organs as illustrations of the repair process. Cell and Tissue Regeneration The regeneration of injured cells and tissues involves cell proliferation, which is driven by growth factors and is critically dependent on the integrity of the extracellular matrix, and by the development of mature cells from stem cells. Before describing examples of repair by regen eration, the general principles of cell proliferation are discussed. Cell Proliferation: Signals and Control Mechanisms Several cell types proliferate during tissue repair. These include the remnants of the injured tissue (which attempt to restore normal structure), vascular endothelial cells (to create new vessels that provide the nutrients needed for the repair process), and fibroblasts (the source of the fibrous tissue that forms the scar to fill defects that cannot be cor rected by regeneration). The ability of tissues to repair themselves is determined, in part, by their intrinsic proliferative capacity. Based on this criterion, the tissues of the body are divided into three groups. • • • Labile (continuously dividing) tissues. Cells of these tissues are continuously being lost and replaced by maturation from tissue stem cells and by proliferation of mature cells. Labile cells include hematopoietic cells in the bone marrow and the majority of surface epithe lia, such as the stratified squamous epithelia of the skin, oral cavity, vagina, and cervix; the cuboidal epithelia of the ducts draining exocrine organs (e.g., salivary glands, pancreas, biliary tract); the columnar epithelium of the gastrointestinal tract, uterus, and fallopian tubes; and the transitional epithelium of the urinary tract. These tissues can readily regenerate after injury as long as the pool of stem cells is preserved. Stable tissues. Cells of these tissues are quiescent (in the G0 stage of the cell cycle) and have only minimal prolif erative activity in their normal state. However, these cells are capable of dividing in response to injury or loss of tissue mass. Stable cells constitute the parenchyma of most solid tissues, such as liver, kidney, and pancreas. They also include endothelial cells, fibroblasts, and smooth muscle cells; the proliferation of these cells is particularly important in wound healing. With the exception of liver, stable tissues have a limited capacity to regenerate after injury. Permanent tissues. The cells of these tissues are consid ered to be terminally differentiated and nonproliferative in postnatal life. The majority of neurons and cardiac muscle cells belong to this category. Thus, injury to the brain or heart is irreversible and results in a scar, because neurons and cardiac myocytes cannot regenerate. Limited stem cell replication and differentiation occur in some areas of the adult brain, and there is some evi dence that heart muscle cells may proliferate after myo cardial necrosis. Nevertheless, whatever proliferative Although it is believed that most mature tissues contain variable proportions of continuously dividing cells, quies cent cells that can return to the cell cycle, and nondividing cells, it is actually difficult to quantify the proportions of these cells in any tissue. Also, we now realize that cell proliferation is only one pathway of regeneration and that stem cells contribute to this process in important ways. Cell proliferation is driven by signals provided by growth factors and from the extracellular matrix. Many different growth factors have been described; some act on multiple cell types and others are cell-selective (Chapter 1, Table 1-1). Growth factors are typically produced by cells near the site of damage. The most important sources of these growth factors are macrophages that are activated by the tissue injury, but epithelial and stromal cells also produce some of these factors. Several growth factors bind to ECM proteins and are displayed at high concentrations. All growth factors activate signaling pathways that ulti mately induce the production of proteins that are involved in driving cells through the cell cycle and other proteins that release blocks on the cell cycle (checkpoints) (Chapter 1). In addition to responding to growth factors, cells use integrins to bind to ECM proteins, and signals from the integrins can also stimulate cell proliferation. In the process of regeneration, proliferation of residual cells is supplemented by development of mature cells from stem cells. In Chapter 1 we introduced the major types of stem cells. In adults, the most important stem cells for regeneration after injury are tissue stem cells. These stem cells live in specialized niches, and it is believed that injury triggers signals in these niches that activate quiescent stem cells to proliferate and differentiate into mature cells that repopulate the injured tissue. Mechanisms of Tissue Regeneration The importance of regeneration in the replacement of injured tissues varies in different types of tissues and with the severity of injury. • • In labile tissues, such as the epithelia of the intestinal tract and skin, injured cells are rapidly replaced by pro liferation of residual cells and differentiation of tissue stem cells provided the underlying basement mem brane is intact. The growth factors involved in these processes are not defined. Loss of blood cells is cor rected by proliferation of hematopoietic stem cells in the bone marrow and other tissues, driven by growth factors called colony-stimulating factors (CSFs), which are produced in response to the reduced numbers of blood cells. Tissue regeneration can occur in parenchymal organs with stable cell populations, but with the exception of the liver, this is usually a limited process. Pancreas, adrenal, thyroid, and lung have some regenerative capacity. The surgical removal of a kidney elicits in the remaining kidney a compensatory response that 101 102 CHAPTER 3 Inflammation and Repair consists of both hypertrophy and hyperplasia of proxi mal duct cells. The mechanisms underlying this response are not understood, but likely involve local production of growth factors and interactions of cells with the ECM. The extraordinary capacity of the liver to regenerate has made it a valuable model for studying this process, as described below. Restoration of normal tissue structure can occur only if the residual tissue is structurally intact, as after partial surgical resection. By contrast, if the entire tissue is damaged by infection or inflammation, regeneration is incomplete and is accompanied by scarring. For example, extensive destruction of the liver with collapse of the retic ulin framework, as occurs in a liver abscess, leads to scar formation even though the remaining liver cells have the capacity to regenerate. Liver Regeneration The human liver has a remarkable capacity to regenerate, as demonstrated by its growth after partial hepatectomy, which may be performed for tumor resection or for livingdonor hepatic transplantation. The mythologic image of liver regeneration is the regrowth of the liver of Prometheus, which was eaten every day by an eagle sent by Zeus as punishment for stealing the secret of fire, and grew back overnight. The reality, although less dramatic, is still quite impressive. Regeneration of the liver occurs by two major mechanisms: proliferation of remaining hepatocytes and repopulation from progenitor cells. Which mechanism plays the dominant role depends on the nature of the injury. • Proliferation of hepatocytes following partial hepatectomy. In humans, resection of up to 90% of the liver can be corrected by proliferation of the residual hepatocytes. This classic model of tissue regeneration has been used experimentally to study the initiation and control of the process. Hepatocyte proliferation in the regenerating liver is triggered by the combined actions of cytokines and polypeptide growth factors. The process occurs in dis tinct stages (Fig. 3-25). In the first, or priming, phase, cytokines such as IL-6 are produced mainly by Kupffer cells and act on hepatocytes to make the parenchymal cells competent to receive and respond to growth factor signals. In the second, or growth factor, phase, growth factors such as HGF and TGF-α, produced by many cell types, act on primed hepatocytes to stimulate cell metabolism and entry of the cells into the cell cycle. Because hepatocytes are quiescent cells, it takes them several hours to enter the cell cycle, progress from G0 to G1, and reach the S phase of DNA replication. Almost all hepatocytes replicate during liver regeneration after partial hepatectomy. The wave of hepatocyte rep lication is followed by replication of nonparenchymal cells (Kupffer cells, endothelial cells, and stellate cells). During the phase of hepatocyte replication, more than 70 genes are activated; these include genes encoding transcription factors, cell cycle regulators, regulators of energy metabolism, and many others. In the final, termination, phase, hepatocytes return to quiescence. The nature of the stop signals is poorly understood; Sinusoid TNF EGF, TGF-α Kuppfer cell Space of Disse IL-6 EGFR HGF MET G0 to G1 transition Hepatocytes PRIMING CELL PROLIFERATION Figure 3-25 Liver regeneration by proliferation of hepatocytes. Following partial hepatectomy, the liver regenerates by proliferation of surviving cells. The process occurs in stages, including priming, followed by growth factorinduced proliferation. The main signals involved in these steps are shown. Once the mass of the liver is restored, the proliferation is terminated (not shown). antiproliferative cytokines of the TGF-β family are likely involved. Liver regeneration from progenitor cells. In situations where the proliferative capacity of hepatocytes is impaired, such as after chronic liver injury or inflamma tion, progenitor cells in the liver contribute to repopula tion. In rodents, these progenitor cells have been called oval cells because of the shape of their nuclei. Some of these progenitor cells reside in specialized niches called canals of Hering, where bile canaliculi connect with larger bile ducts. The signals that drive proliferation of pro genitor cells and their differentiation into mature hepa tocytes are topics of active investigation. • KEY CONCEPTS Repair by Regeneration ■ ■ ■ ■ Tissues are classified as labile, stable, and permanent, according to the proliferative capacity of their cells. Continuously dividing tissues (labile tissues) contain stem cells that differentiate to replenish lost cells and maintain tissue homeostasis. Cell proliferation is controlled by the cell cycle, and is stimulated by growth factors and interactions of cells with the extracellular matrix. Regeneration of the liver is a classic example of repair by regeneration. It is triggered by cytokines and growth factors produced in response to loss of liver mass and inflammation. In different situations, regeneration may occur by proliferation of surviving hepatocytes or repopulation from progenitor cells. Repair by Connective Tissue Deposition If repair cannot be accomplished by regeneration alone it occurs by replacement of the injured cells with connective tissue, leading to the formation of a scar, or by a combination of regeneration of some residual cells and scar formation. As discussed earlier, scarring may happen Tissue repair if the tissue injury is severe or chronic and results in damage to parenchymal cells and epithelia as well as to the connective tissue framework, or if nondividing cells are injured. In contrast to regeneration, which involves the restitution of tissue components, scar formation is a response that “patches” rather than restores the tissue. The term scar is most often used in connection to wound healing in the skin, but may also be used to describe the replace ment of parenchymal cells in any tissue by collagen, as in the heart after myocardial infarction. NORMAL Infection or injury TISSUE INJURY Steps in Scar Formation Repair by connective tissue deposition consists of sequen tial processes that follow tissue injury and the inflamma tory response (Fig. 3-26): • Angiogenesis is the formation of new blood vessels, which supply nutrients and oxygen needed to support the repair process. Newly formed vessels are leaky because of incomplete interendothelial junctions and because VEGF, the growth factor that drives angiogen esis, increases vascular permeability. This leakiness accounts in part for the edema that may persist in healing wounds long after the acute inflammatory response has resolved. • Formation of granulation tissue. Migration and prolif eration of fibroblasts and deposition of loose connective tissue, together with the vessels and interspersed leuko cytes, form granulation tissue. The term granulation tissue derives from its pink, soft, granular gross appearance, such as that seen beneath the scab of a skin wound. Its histologic appearance is characterized by proliferation of fibroblasts and new thin-walled, delicate capillaries (angiogenesis), in a loose extracellular matrix, often with admixed inflammatory cells, mainly macrophages (Fig. 3-27A). Granulation tissue progressively invades the site of injury; the amount of granulation tissue that is formed depends on the size of the tissue deficit created by the wound and the intensity of inflammation. • Remodeling of connective tissue. Maturation and reor ganization of the connective tissue (remodeling) produce the stable fibrous scar. The amount of connective tissue increases in the granulation tissue, eventually resulting A Area of injury INFLAMMATION FORMATION OF GRANULATION TISSUE SCAR FORMATION Figure 3-26 Steps in repair by scar formation. Injury to a tissue, such as muscle (which has limited regenerative capacity), first induces inflammation, which clears dead cells and microbes, if any. This is followed by the formation of vascularized granulation tissue and then the deposition of extracellular matrix to form the scar. B Figure 3-27 A, Granulation tissue showing numerous blood vessels, edema, and a loose extracellular matrix containing occasional inflammatory cells. Collagen is stained blue by the trichrome stain; minimal mature collagen can be seen at this point. B, Trichrome stain of mature scar, showing dense collagen, with only scattered vascular channels. 103 104 CHAPTER 3 Inflammation and Repair in the formation of a scar (Fig. 3-27B), which may remodel over time. Quiescent vessel Macrophages play a central role in repair by clearing offending agents and dead tissue, providing growth factors for the proliferation of various cells, and secreting cytokines that stimulate fibroblast proliferation and connective tissue synthesis and deposition. The macrophages that are involved in repair are mostly of the alternatively activated (M2) type. It is not clear how the classically acti vated macrophages that dominate during inflammation, and are involved in getting rid of microbes and dead tissues, are gradually replaced by alternatively activated macrophages that serve to terminate inflammation and induce repair. Repair begins within 24 hours of injury by the emi gration of fibroblasts and the induction of fibroblast and endothelial cell proliferation. By 3 to 5 days, the special ized granulation tissue that is characteristic of healing is apparent. We next describe the steps in the formation of granula tion tissue and the scar. Vasodilation (VEGF) Leading (”tip”) cell (VEGF, Notch signals) Angiogenic factors Pericyte Pericyte detachment (angiopoietin) Basement membrane Basement membrane degradation (MMPs) Endothelium Pericyte recruitment ECM Elongation of vascular stalk Angiogenesis Angiogenesis is the process of new blood vessel development from existing vessels. It is critical in healing at sites of injury, in the development of collateral circulations at sites of ischemia, and in allowing tumors to increase in size beyond the constraints of their original blood supply. Much work has been done to understand the mechanisms underlying angiogenesis, and therapies to either augment the process (e.g., to improve blood flow to a heart ravaged by coronary atherosclerosis) or inhibit it (to frustrate tumor growth or block pathologic vessel growth such as in dia betic retinopathy) are being developed. Angiogenesis involves sprouting of new vessels from existing ones, and consists of the following steps (Fig. 3-28): • • • • • • • Vasodilation in response to nitric oxide and increased permeability induced by vascular endothelial growth factor (VEGF) Separation of pericytes from the abluminal surface and breakdown of the basement membrane to allow forma tion of a vessel sprout Migration of endothelial cells toward the area of tissue injury Proliferation of endothelial cells just behind the leading front (“tip”) of migrating cells Remodeling into capillary tubes Recruitment of periendothelial cells (pericytes for small capillaries and smooth muscle cells for larger vessels) to form the mature vessel Suppression of endothelial proliferation and migration and deposition of the basement membrane. The process of angiogenesis involves several signaling pathways, cell-cell interactions, ECM proteins, and tissue enzymes. • Growth factors. Vascular endothelial growth factors (VEGFs), mainly VEGF-A (Chapter 1), stimulates both migration and proliferation of endothelial cells, thus initiating the process of capillary sprouting in Formation of new vessel Figure 3-28 Angiogenesis. In tissue repair, angiogenesis occurs mainly by sprouting of new vessels. The steps in the process, and the major signals involved, are illustrated. The newly formed vessel joins up with other vessels (not shown) to form the new vascular bed. • angiogenesis. It promotes vasodilation by stimulating the production of NO and contributes to the formation of the vascular lumen. Fibroblast growth factors (FGFs), mainly FGF-2, stimulates the proliferation of endothelial cells. It also promotes the migration of macrophages and fibroblasts to the damaged area, and stimulates epi thelial cell migration to cover epidermal wounds. Angio poietins 1 and 2 (Ang 1 and Ang 2) are growth factors that play a role in angiogenesis and the structural matura tion of new vessels. Newly formed vessels need to be stabilized by the recruitment of pericytes and smooth muscle cells and by the deposition of connective tissue. Ang1 interacts with a tyrosine kinase receptor on endo thelial cells called Tie2. The growth factors PDGF and TGF-β also participate in the stabilization process: PDGF recruits smooth muscle cells and TGF-β suppresses endothelial proliferation and migration, and enhances the production of ECM proteins. Notch signaling. Through “cross-talk” with VEGF, the Notch signaling pathway regulates the sprouting and branching of new vessels and thus ensures that the new vessels that are formed have the proper spacing to effec tively supply the healing tissue with blood. Tissue repair • • ECM proteins participate in the process of vessel sprout ing in angiogenesis, largely through interactions with integrin receptors in endothelial cells and by providing the scaffold for vessel growth. Enzymes in the ECM, notably the matrix metallopro teinases (MMPs), degrade the ECM to permit remodel ing and extension of the vascular tube. Deposition of Connective Tissue The laying down of connective tissue occurs in two steps: (1) migration and proliferation of fibroblasts into the site of injury and (2) deposition of ECM proteins produced by these cells. These processes are orchestrated by locally produced cytokines and growth factors, including PDGF, FGF-2, and TGF-β. The major sources of these factors are inflammatory cells, particularly alternatively activated (M2) macrophages, which are present at sites of injury and in granulation tissue. Sites of inflammation are also rich in mast cells, and in the appropriate chemotactic milieu lym phocytes may also be present. Each of these can secrete cytokines and growth factors that contribute to fibroblast proliferation and activation. Transforming growth factor-β (TGF-β) is the most important cytokine for the synthesis and deposition of connective tissue proteins. It is produced by most of the cells in granulation tissue, including alternatively activated macrophages. The levels of TGF-β in tissues are primarily regulated not by the transcription of the gene but by the posttranscriptional activation of latent TGF-β, the rate of secretion of the active molecule, and factors in the ECM, notably integrins, that enhance or diminish TGF-β activity. TGF-β stimulates fibroblast migration and proliferation, increased synthesis of collagen and fibronectin, and decreased degradation of ECM due to inhibition of metal loproteinases. TGF-β is involved not only in scar formation after injury but also in the development of fibrosis in lung, liver, and kidneys that follows chronic inflammation. TGF-β is also an antiinflammatory cytokine that serves to limit and terminate inflammatory responses. It does this by inhibiting lymphocyte proliferation and the activity of other leukocytes. As healing progresses, the number of proliferating fibroblasts and new vessels decreases; however, the fibro blasts progressively assume a more synthetic phenotype, and hence there is increased deposition of ECM. Collagen synthesis, in particular, is critical to the development of strength in a healing wound site. As described later, col lagen synthesis by fibroblasts begins early in wound healing (days 3 to 5) and continues for several weeks, depending on the size of the wound. Net collagen accumu lation, however, depends not only on increased synthesis but also on diminished collagen degradation (discussed later). Ultimately, the granulation tissue evolves into a scar composed of largely inactive, spindle-shaped fibroblasts, dense collagen, fragments of elastic tissue, and other ECM components (Fig. 3-27B). As the scar matures, there is pro gressive vascular regression, which eventually transforms the highly vascularized granulation tissue into a pale, largely avascular scar. Some of the fibroblasts also acquire features of smooth muscle cells, including the presence of actin filaments, and are called myofibroblasts. These cells contribute to the contraction of the scar over time. Remodeling of Connective Tissue The outcome of the repair process is influenced by a balance between synthesis and degradation of ECM proteins. After its deposition, the connective tissue in the scar continues to be modified and remodeled. The degradation of collagens and other ECM components is accomplished by a family of matrix metalloproteinases (MMPs), so called because they are dependent on metal ions (e.g., zinc) for their activity. MMPs should be distinguished from neutro phil elastase, cathepsin G, plasmin, and other serine proteinases that can also degrade ECM but are not metal loenzymes. MMPs include interstitial collagenases, which cleave fibrillar collagen (MMP-1, -2 and -3); gelatinases (MMP-2 and 9), which degrade amorphous collagen and fibronectin; and stromelysins (MMP-3, -10, and -11), which degrade a variety of ECM constituents, including proteo glycans, laminin, fibronectin, and amorphous collagen. MMPs are produced by a variety of cell types (fibro blasts, macrophages, neutrophils, synovial cells, and some epithelial cells), and their synthesis and secretion are regu lated by growth factors, cytokines, and other agents. The activity of the MMPs is tightly controlled. They are pro duced as inactive precursors (zymogens) that must be first activated; this is accomplished by proteases (e.g., plasmin) likely to be present only at sites of injury. In addition, acti vated collagenases can be rapidly inhibited by specific tissue inhibitors of metalloproteinases (TIMPs), produced by most mesenchymal cells. Thus, during scar formation, MMPs are activated to remodel the deposited ECM and then their activity is shut down by the TIMPs. A family of enzymes related to MMPs is called ADAM (a disintegrin and metalloproteinase). ADAMs are anchored to the plasma membrane and cleave and release extracel lular domains of cell-associated cytokines and growth factors, such as TNF, TGF-β, and members of the EGF family. KEY CONCEPTS Repair by Scar Formation ■ ■ ■ ■ ■ Tissues are repaired by replacement with connective tissue and scar formation if the injured tissue is not capable of proliferation or if the structural framework is damaged and cannot support regeneration. The main components of connective tissue repair are angiogenesis, migration and proliferation of fibroblasts, collagen synthesis, and connective tissue remodeling. Repair by connective tissue starts with the formation of granulation tissue and culminates in the laying down of fibrous tissue. Multiple growth factors stimulate the proliferation of the cell types involved in repair. TGF-β is a potent fibrogenic agent; ECM deposition depends on the balance between fibrogenic agents, metalloproteinases (MMPs) that digest ECM, and the tissue inhibitors of MMPs (TIMPs). Factors That Influence Tissue Repair Tissue repair may be altered by a variety of influences, frequently reducing the quality or adequacy of the repar ative process. Variables that modify healing may be extrin 105 106 CHAPTER 3 Inflammation and Repair sic (e.g., infection) or intrinsic to the injured tissue, and systemic or local: • • • • • • • • • Infection is clinically one of the most important causes of delay in healing; it prolongs inflammation and poten tially increases the local tissue injury. Diabetes is a metabolic disease that compromises tissue repair for many reasons (Chapter 24), and is one of the most important systemic causes of abnormal wound healing. Nutritional status has profound effects on repair; protein deficiency, for example, and particularly vitamin C deficiency, inhibits collagen synthesis and retards healing. Glucocorticoids (steroids) have well-documented anti inflammatory effects, and their administration may result in weakness of the scar due to inhibition of TGF-β production and diminished fibrosis. In some instances, however, the anti-inflammatory effects of glucocorti coids are desirable. For example, in corneal infections, glucocorticoids are sometimes prescribed (along with antibiotics) to reduce the likelihood of opacity that may result from collagen deposition. Mechanical factors such as increased local pressure or torsion may cause wounds to pull apart, or dehisce. Poor perfusion, due either to arteriosclerosis and diabe tes or to obstructed venous drainage (e.g., in varicose veins), also impairs healing. Foreign bodies such as fragments of steel, glass, or even bone impede healing. The type and extent of tissue injury affects the subse quent repair. Complete restoration can occur only in tissues composed of stable and labile cells; even then, extensive injury will probably result in incomplete tissue regeneration and at least partial loss of function. Injury to tissues composed of permanent cells must inevitably result in scarring with, at most, attempts at functional compensation by the remaining viable ele ments. Such is the case with healing of a myocardial infarct. The location of the injury and the character of the tissue in which the injury occurs are also important. For example, inflammation arising in tissue spaces (e.g., pleural, peritoneal, synovial cavities) develops exten sive exudates. Subsequent repair may occur by diges tion of the exudate, initiated by the proteolytic enzymes of leukocytes and resorption of the liquefied exudate. This is called resolution, and in the absence of cellular necrosis, normal tissue architecture is generally restored. However, in the setting of larger accumulations, the exudate undergoes organization: granulation tissue grows into the exudate, and a fibrous scar ultimately forms. Selected Clinical Examples of Tissue Repair and Fibrosis So far, we have discussed the general principles and mech anisms of repair by regeneration and scar formation. In this section we describe two clinically significant types of repair—the healing of skin wounds (cutaneous wound healing) and fibrosis in injured parenchymal organs. Healing of Skin Wounds This is a process that involves both epithelial regeneration and the formation of connective tissue scar and is thus illustrative of the general principles that apply to healing in all tissues. Based on the nature and size of the wound, the healing of skin wounds is said to occur by first or second intention. Healing by First Intention When the injury involves only the epithelial layer, the principal mechanism of repair is epithelial regeneration, also called primary union or healing by first intention. One of the simplest examples of this type of wound repair is the healing of a clean, uninfected surgical incision approxi mated by surgical sutures (Fig. 3-29). The incision causes only focal disruption of epithelial basement membrane continuity and death of relatively few epithelial and con nective tissue cells. The repair consists of three connected processes: inflammation, proliferation of epithelial and other cells, and maturation of the connective tissue scar. • • • • Wounding causes the rapid activation of coagulation pathways, which results in the formation of a blood clot on the wound surface (Chapter 4). In addition to entrapped red cells, the clot contains fibrin, fibronectin, and complement proteins. The clot serves to stop bleeding and acts as a scaffold for migrating cells, which are attracted by growth factors, cytokines, and chemokines released into the area. Release of VEGF leads to increased vessel permeability and edema. As dehydration occurs at the external surface of the clot, a scab covering the wound is formed. Within 24 hours, neutrophils are seen at the incision margin, migrating toward the fibrin clot. They release proteolytic enzymes that begin to clear the debris. Basal cells at the cut edge of the epidermis begin to show increased mitotic activity. Within 24 to 48 hours, epithe lial cells from both edges have begun to migrate and proliferate along the dermis, depositing basement mem brane components as they progress. The cells meet in the midline beneath the surface scab, yielding a thin but continuous epithelial layer that closes the wound. By day 3, neutrophils have been largely replaced by macrophages, and granulation tissue progressively invades the incision space. As mentioned earlier, mac rophages are key cellular constituents of tissue repair, clearing extracellular debris, fibrin, and other foreign material, and promoting angiogenesis and ECM deposi tion. Collagen fibers are now evident at the incision margins. Epithelial cell proliferation continues, forming a covering approaching the normal thickness of the epidermis. By day 5, neovascularization reaches its peak as granu lation tissue fills the incisional space. These new vessels are leaky, allowing the passage of plasma proteins and fluid into the extravascular space. Thus, new granula tion tissue is often edematous. Migration of fibroblasts to the site of injury is driven by chemokines, TNF, PDGF, TGF-β, and FGF. Their subsequent proliferation is triggered by multiple growth factors, including PDGF, EGF, TGF-β, and FGF, and the cytokines IL-1 and Tissue repair HEALING BY FIRST INTENTION HEALING BY SECOND INTENTION Scab Neutrophils 24 hours Clot Mitoses Granulation tissue Macrophage 3 to 7 days Weeks Fibroblast New capillary Wound contraction Fibrous union Figure 3-29 Steps in wound healing by first intention (left) and second intention (right). In the latter, note the large amount of granulation tissue and wound contraction. • TNF. Macrophages are the main source for these factors, although other inflammatory cells and platelets may also produce them. The fibroblasts produce ECM pro teins, and collagen fibrils become more abundant and begin to bridge the incision. The epidermis recovers its normal thickness as differentiation of surface cells yields a mature epidermal architecture with surface keratinization. During the second week, there is continued collagen accumulation and fibroblast proliferation. The leuko cyte infiltrate, edema, and increased vascularity are substantially diminished. The process of “blanching” begins, accomplished by increasing collagen deposition within the incisional scar and the regression of vascular channels. • By the end of the first month, the scar comprises a cellular connective tissue largely devoid of inflamma tory cells and covered by an essentially normal epider mis. However, the dermal appendages destroyed in the line of the incision are permanently lost. The tensile strength of the wound increases with time, as described later. Healing by Second Intention When cell or tissue loss is more extensive, such as in large wounds, abscesses, ulceration, and ischemic necrosis (infarction) in parenchymal organs, the repair process involves a combination of regeneration and scarring. In healing of skin wounds by second intention, also known as healing by secondary union (Figs. 3-29 and 3-30), the 107 108 CHAPTER 3 Inflammation and Repair A B C D Figure 3-30 Healing of skin ulcers. A, Pressure ulcer of the skin, commonly found in diabetic patients. The histologic slides show a skin ulcer with a large gap between the edges of the lesion (B), a thin layer of epidermal reepithelialization and extensive granulation tissue formation in the dermis (C), and continuing reepithelialization of the epidermis and wound contraction (D). (Courtesy Z. Argenyi, MD, University of Washington, Seattle, Wash.) inflammatory reaction is more intense, there is develop ment of abundant granulation tissue, accumulation of ECM and formation of a large scar, and wound contraction by the action of myofibroblasts. Secondary healing differs from primary healing in several respects: • • • In wounds causing large tissue deficits, the fibrin clot is larger, and there is more exudate and necrotic debris in the wounded area. Inflammation is more intense because large tissue defects have a greater volume of necrotic debris, exudate, and fibrin that must be removed. Consequently, large defects have a greater potential for secondary, inflammation-mediated, injury. Much larger amounts of granulation tissue are formed. Larger defects require a greater volume of granulation tissue to fill in the gaps and provide the underlying framework for the regrowth of tissue epithelium. A greater volume of granulation tissue generally results in a greater mass of scar tissue. At first a provisional matrix containing fibrin, plasma fibronectin, and type III collagen is formed, but in about 2 weeks this is replaced by a matrix composed primarily of type I collagen. Ultimately, the original granulation tissue scaffold is converted into a pale, avascular scar, composed of spindle-shaped fibroblasts, dense collagen, fragments of elastic tissue, and other ECM components. The dermal appendages that have been • destroyed in the line of the incision are permanently lost. The epidermis recovers its normal thickness and architecture. By the end of the first month, the scar is made up of acellular connective tissue devoid of inflam matory infiltrate, covered by intact epidermis. Wound contraction generally occurs in large surface wounds. The contraction helps to close the wound by decreasing the gap between its dermal edges and by reducing the wound surface area. Hence, it is an impor tant feature in healing by secondary union. The initial steps of wound contraction involve the formation, at the edge of the wound, of a network of myofibroblasts, which are modified fibroblasts exhibiting many of the ultra structural and functional features of contractile smooth muscle cells. Within 6 weeks, large skin defects may be reduced to 5% to 10% of their original size, largely by contraction. Wound Strength Carefully sutured wounds have approximately 70% of the strength of normal skin, largely because of the placement of sutures. When sutures are removed, usually at 1 week, wound strength is approximately 10% of that of unwounded skin, but this increases rapidly over the next 4 weeks. The recovery of tensile strength results from the excess of col lagen synthesis over collagen degradation during the first 2 months of healing, and, at later times, from structural Tissue repair modifications of collagen fibers (cross-linking, increased fiber size) after collagen synthesis ceases. Wound strength reaches approximately 70% to 80% of normal by 3 months but usually does not substantially improve beyond that point. Fibrosis in Parenchymal Organs Deposition of collagen is part of normal wound healing. The term fibrosis is used to denote the excessive deposition of collagen and other ECM components in a tissue. As already mentioned, the terms scar and fibrosis are used interchangeably, but fibrosis most often refers to the abnor mal deposition of collagen that occurs in internal organs in chronic diseases. The basic mechanisms of fibrosis are the same as those of scar formation in the skin during tissue repair. Fibrosis is a pathologic process induced by persistent injurious stimuli such as chronic infections and immunologic reactions, and is typically associated with loss of tissue (Fig. 3-31). It may be responsible for substan tial organ dysfunction and even organ failure. As discussed earlier, the major cytokine involved in fibrosis is TGF-β. The mechanisms that lead to the activa tion of TGF-β in fibrosis are not precisely known, but cell death by necrosis or apoptosis and the production of reac tive oxygen species seem to be important triggers of the activation, regardless of the tissue. Similarly, the cells that produce collagen under TGF-β stimulation may vary depending on the tissue. In most organs, such as in lung and kidney, myofibroblasts are the main source of colla gen, but stellate cells are the major collagen producers in liver cirrhosis. Fibrotic disorders include diverse chronic and debilitat ing diseases such as liver cirrhosis, systemic sclerosis (scleroderma), fibrosing diseases of the lung (idiopathic pulmonary fibrosis, pneumoconioses, and drug-, radiationinduced pulmonary fibrosis), end-stage kidney disease, and constrictive pericarditis. These conditions are dis cussed in the appropriate chapters throughout the book. Because of the tremendous functional impairment caused by fibrosis in these conditions, there is great interest in the development of antifibrotic drugs. Abnormalities in Tissue Repair Complications in tissue repair can arise from abnormalities in any of the basic components of the process, including deficient scar formation, excessive formation of the repair components, and formation of contractures. Epithelium Tissue injury, loss of epithelial integrity • REGENERATION Limited injury Repeated or severe injury Inflammation Macrophages T lymphocytes and other lymphoid cells • Cytokines (e.g., IL-13) TGFβ MMPs Fibroblast recruitment and differentiation Myofibroblasts Extracellular matrix FIBROSIS Figure 3-31 Mechanisms of fibrosis. Persistent tissue injury leads to chronic inflammation and loss of tissue architecture. Cytokines produced by macrophages and other leukocytes stimulate the migration and proliferation of fibroblasts and myofibroblasts and the deposition of collagen and other extracellular matrix proteins. The net result is replacement of normal tissue by fibrosis. • Inadequate formation of granulation tissue or formation of a scar can lead to two types of complications: wound dehiscence and ulceration. Dehiscence or rupture of a wound, although not common, occurs most frequently after abdominal surgery and is due to increased abdominal pressure. Vomiting, coughing, or ileus can generate mechanical stress on the abdominal wound. Wounds can ulcerate because of inadequate vascularization during healing. For example, lower extremity wounds in individuals with atherosclerotic peripheral vascular disease typically ulcerate (Chapter 11). Nonhealing wounds also form in areas devoid of sensation. These neuropathic ulcers are occasionally seen in patients with diabetic peripheral neuropathy (Chapters 24 and 27). Excessive formation of the components of the repair process can give rise to hypertrophic scars and keloids. The accumulation of excessive amounts of collagen may give rise to a raised scar known as a hypertrophic scar; if the scar tissue grows beyond the boundaries of the original wound and does not regress, it is called a keloid (Fig. 3-32). Keloid formation seems to be an individual predisposition, and for unknown reasons this aberra tion is somewhat more common in African Americans. Hypertrophic scars generally develop after thermal or traumatic injury that involves the deep layers of the dermis. Exuberant granulation is another deviation in wound healing consisting of the formation of excessive amounts of granulation tissue, which protrudes above the level of the surrounding skin and blocks reepithelialization (this process has been called, with more literary fervor, proud flesh). Excessive granulation must be removed by cautery or surgical excision to permit restoration of the 109