Respiratory Physiology PDF
Document Details
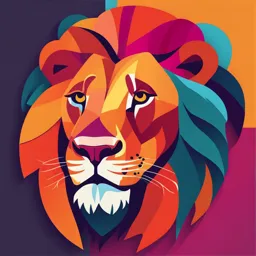
Uploaded by SuccessfulJuniper
University of Adelaide
Tags
Summary
Respiratory Physiology lecture notes. This document covers fundamental concepts in respiratory physiology, including systemic vs pulmonary circulation, the relationship between alveoli and lung capillaries, O2 delivery variables, the oxyhemoglobin dissociation curve, and V/Q mismatch.
Full Transcript
Foundations of Critical Care Respiratory Physiology Objectives Understand systemic vs pulmonary circulation Review the relationship between alveoli and lung capillaries Understand the variables of O2 delivery Understand the Oxyhaemoglobin Dissociation Curve Discuss V/Q misma...
Foundations of Critical Care Respiratory Physiology Objectives Understand systemic vs pulmonary circulation Review the relationship between alveoli and lung capillaries Understand the variables of O2 delivery Understand the Oxyhaemoglobin Dissociation Curve Discuss V/Q mismatch - shunt and dead space University of Adelaide 2 Systemic vs Pulmonary Circulation White board University of Adelaide 4 Lung Capillaries University of Adelaide 5 University of Adelaide 6 University of Adelaide 8 University of Adelaide 9 Composition of atmospheric air Can you guess the composition of the air we breathe? University of Adelaide 10 Quick Review What is Cardiac Output? Pre Load Stroke Volume Contractility After Load Ejection Fraction?? University of Adelaide 11 Still unpacking… Difference between SaO2 and PaO2? Normal Hb? When do we top it up? How should we treat low SpO2? FiO2 – fraction of inspired Oxygen. Room Air = 21% FiO2 – 0.21% 10- 15L NRB = 60-80% FiO2 (depends on leak) University of Adelaide 12 Oxygen delivery variables DO2 CO x (SaO2 x Hb x 1.34) + 0.003(PaO2) x 10 University of Adelaide 13 Variables CO SaO2 Hb PaO2 Is giving more Oxygen the answer? University of Adelaide 14 Steps of Oxygen Delivery O2 Available to breathe Airway - Into the alveoli Transmembrane diffusion – A-a gradient Vascular – Red cells available for binding Cardiac Output – Blood moving to take O2 to the tissues Hb releasing O2 adequately – Tissue uptake Use of O2 by tissue University of Adelaide 15 Hypoxia vs Hypoxaemia from Greek hupo ‘under’ 1940s Hypo + Oxy = Hypoxia Haima (Greek) turned into hemia (Latin) = ‘blood’ What is Hypoxaemia? What is Hypoxia? University of Adelaide 16 Why is Hypoxia so bad? What happens in the tissues when there is no O2? What do they use? Do the cells die? University of Adelaide 17 Cellular Respiration Aerobic Metabolism Anaerobic Metabolism University of Adelaide 18 Aerobic Metabolism Glucose + Pyruvic acid + O2 = 38 ATP + CO2 + H2O University of Adelaide 19 Anaerobic Metabolism Glucose + Pyruvic Acid = 2 lactic acid + 2 ATP University of Adelaide 20 University of Adelaide 21 The Lactate Dilemma - Lactate is produced in the presence of anaerobic metabolism; - The problem with that is that for a cell to go into anaerobic metabolism it takes a very low Delivery of Oxygen; - Lactate is also produced in the presence of Oxygen, for fuel (glycolysis – pyruvic acid – lactate); - It is a marker of physiological stress; released when there is an adrenergic response, with or without tissue hypoxia; - What we know: a high lactate is associated with increased mortality. Why? It is not the lactate’s fault. It is just a marker of stress. University of Adelaide 22 More… - The decrease of lactate shows that the stress is going down. The patient is improving; - A high lactate shows a problem – always; University of Adelaide 23 West Zones PA Alveolar pressure Pa Arterial pressure PV Venous pressure University of Adelaide 24 Zones of Pulmonary Blood Flow Three functional zones of pulmonary blood flow can be defined based on the relevant pressure gradient actuating flow of blood. In Zone 1 where no blood flows the alveolar pressure (PA) is greater than both the arterial (Pa) and venous pressures (Pv). In Zone 2 where moderate blood flow occurs the relevant pressure gradient is between the arterial pressure and the alveolar pressure. In Zone 3 where the greatest blood flow occurs the relevant pressure gradient is between the arterial and venous pressures. University of Adelaide 25 University of Adelaide 26 VQ mismatch - Shunt and dead space https://ars.els-cdn.com/content/image/1-s2.0- S2049080122005805-gr5_lrg.jpg https://ars.els-cdn.com/content/image/1-s2.0-S2049080122005805- gr5_lrg.jpg University of Adelaide 27 Oxyhaemoglobin Dissociation Curve The same way it binds, now it needs to unbind; Oxygen has an affinity for Hb, it doesn’t want to unbind… In comes 2,3 DPG (Diphosphoglycerate) – by-product of glycolysis in the red cell. Helps regulate the affinity between Hb and O2 – repellent; The tissues have a very low concentration of O2, because it uses so much. The difference in concentration from SaO2 to the intracellular will weaken the affinity, promoting unloading. University of Adelaide 28 University of Adelaide 29 University of Adelaide 30 It is not pathological to shift to the right or to the left Muscles/placenta: Increased temperature (more blood flow) Higher level of CO2 (more acidic environment) High requirement of O2 (Exercise) Less affinity- needs to unload More 2,3 DPG Shift to the Right Lungs: Lower temperature High level of O2 More affinity - holds onto it Less 2,3 DPG Shift to the left University of Adelaide 31 It becomes pathological when it shifts out of balance… University of Adelaide 32 Questions? Thank you University of Adelaide 33 Respiratory Monitoring Foundations of Critical Care 2024 D.Freer Learning objectives Review essentials of respiratory monitoring Answer the questions What is respiratory monitoring? Different forms of monitoring available Who do we monitor? Why do we monitor? Monitoring Monitor – Verb “to watch and check a situation carefully for a period of time in order to discover something about it” “to watch closely for purposes of control, surveillance, etc.; keep track of; check continually” Latin: Monit - warned Case Study Mr Peter Rabbit Age: 35 Presentation: Elective laparoscopic bariatric surgery PMHx: ▪ Obstructive Sleep Apnoea – home CPAP ▪ Previous Respiratory arrest following sedation for endoscopy ▪ BMI 40 ▪ Ischemic Heart Disease Progress In OT: ▪Bleeding from liver Laceration and splenic hilum; ▪No record of respiratory monitoring for last 1h 30 min prior to discharge to Recovery?? In Recovery: ▪SpO2 drop 2x below 90%; ▪Decision for pt’s own CPAP; ▪Pt’s own CPAP does not fit supplemental O2; ▪CPAP idea abandoned, continues with a CIG/Hudson mask; ▪Mr Rabbit is in severe pain; ▪Morphine pain protocol; ▪Over recovery stay SpO2 80-95%. In HDU ▪Mr Rabbit is admitted to HDU at 19:40h; ▪No Cardiac monitoring is connected as it is only used for cardiac patients (there was equipment available); ▪From 19:40 – 20:20 Mr Rabbit’s monitor alarmed 33 times for low SpO2. ▪Mrs Rabbit, while visiting her husband says that he sounds like he is sleeping without his CPAP machine but much worse. She goes home at 19:55; ▪2 x the RNs in HDU reposition the SpO2 finger probe, attempt to rouse the patient and observe his SpO2 climb slowly to 90% Conclusion ▪At 20:22 Mr Rabbit’s monitor beeps inoperative and there is a question mark after a flat plethysmographic waveform. ▪At 20:38 the RN comes to review him; ▪A Code Blue is called… Unfortunately, Mr. Rabbit died in 2013 as a result of ischemic encephalopathy following laparoscopic bariatric surgery with iatrogenic spleen and liver damage (? Artery lacerated?) (Coroner’s report) Are we satisfied with the respiratory monitoring that Mr. Rabbit received? What would you have done differently? What respiratory monitoring is available? Nurse initiated Other Inspection Imaging WOB X-ray RR CT Palpation VQ Auscultation Ultrasound Pulse Oximetry MRI Capnography Bronchoscopy VBG Vent feedback Peak Flow ABG Spirometry Let’s review Why do we breathe? Oxygen in – oxygenation CO2 out – ventilation We use various forms of monitoring to assess both oxygenation and ventilation How do we breathe normally ? Voluntary OR Cellular metabolism generates CO2 High levels of CO2 detected centrally Phrenic nerve innervated Diaphragm moves down Intercostals move out Mixed gases come in 21% Oxygen 1% CO2, Argon, water vapour, and other gases 78% Nitrogen Gas exchange occurs Then everything relaxes and CO2 is exhaled Inspection Look at your patient – make sure you undress them in the ED!! - Equal expansion - Signs of trauma Work of breathing – assessing oxygenation or ventilation? - Ventilation - Combination of RR and TV – think minute ventilation Respiratory Rate – Count it! Why is it the first vital sign to go off? Palpation Palpate equal expansion Tenderness Subcutaneous emphysema Auscultation How many of you own a stethoscope? How many of you use it? What can you tell with a stethoscope? Pulse Oximetry How many Red cells in the body? How many Hb in one red cell? Pulse Oximetry is a non-invasive method of measuring the percentage of Haemoglobin (Hb) saturated with Oxygen (O2) in arterial blood PaO2 vs Hb carrying capacity SpO2 SaO2 Can also measure pulse amplitude and pulse rate Continuous or intermittent Pulse Oximetry Limitations Only as good as the operator Positioning of the probe Intravascular dyes – methylene blue Nail varnish will give falsely low readings Anaemia Vasoconstriction / decreased perfusion (low flow states) Cannot distinguish between different forms of Hb Carboxyharmoglobinaemia Methheamoglobinaemia Accurate in general above 80%, below CHECK with ABG Oxygen-Haemoglobin Dissociation Curve Describes the sigmoidal relationship between the partial pressure of oxygen (PaO2) and the oxygen saturation of haemoglobin Describes the changing affinity of haemoglobin for oxygen which occurs with increasing PaO2 It can shift - Physiologically - Pathologically Effect of the shift on SpO2 The same SpO2 can have different relationships to PaO2: - With a right shift have higher PaO2 (acidosis, high CO2, high temp, more 2- 3DPG) Oxygen has a greater affinity to offload from the Hb - With a left shift have lower PaO2 (alkalosis, low CO2, hypothermia, less 2- 3DPG) Oxygen doesn’t want to leave the Hb Always interpret SpO2 in light of underlying pathology that could affect the oxygen- haemoglobin dissociation curve Capnography The measurement of carbon dioxide (CO2) in expired air Continuous, non-invasive Capnometer - device that measures and displays a numerical value of carbon dioxide Capnograph - also displays the carbon dioxide waveform Infrared spectroscopy (absorption) ▪A beam of infrared light is passed through a gas sample containing CO2 ▪CO2 molecules absorb specific wavelengths of infrared light energy ▪Light emerging from the sample is analysed ▪A ratio of the CO2 affected wavelengths to the non-affected wavelengths is reported as ETCO2 Indications Confirmation of tracheal intubation Real-time surrogate CO2 monitoring: Patients with CO2 retention Head injured patients Ongoing intubation and ventilation Ventilator weaning Assessment of ROSC Limitations Nasal probe placement – normally not enough gas flow Sensor is susceptible to blockage by secretions or condensation May erroneously read Nitrous Oxide or helium as CO2 Who do we monitor? So, we have discussed what respiratory monitoring is. Who do we monitor? What do we base our monitoring on? Why do we monitor? Looking for those warning signs If you see a warning sign, do something about it. Better to not monitor than to monitor without action. Case Study 1 Identity: Jack Black, 24 Y.O M Situation: Brought into ED on a Friday night by friends. Was out on the town with friends and “took some odd pills” before becoming unrousable. Nil evidence of trauma Background: Nil PmHx, no regular medications Case Study 1 A: What monitoring do you want to inform your Assessment? Airway Inspection – look, listen, feel. Maintaining own. Sonorous respirations. Dried vomit around mouth. C-spine – immobilise or not? Breathing What monitoring? Breathing spontaneously, RR – 8bpm. SpO2 92% RA. Nasal ETCO2 50mmHg, equal chest wall expansion, nil trauma Circulation Warm, well perfused peripheries. HR 130 sinus, BP 100/50 Disability: What assessment / monitoring? Rousable to pain, GCS 11 (E2, V4, M5). BSL 5.4 Exposure Nil external evidence of trauma, t- 35.0 Case Study 1 Still on Assessment What ongoing respiratory monitoring is essential for Jack? Why? What shift will his SpO2 curve have? Why? What does this shift mean for his PaO2 and oxygen reserve? What are your recommendations for Jack’s care? Case study 2 Identity Thelma, 60 YO Female Situation: Presented to ED with acute SOB. Commenced on CPAP & GTN infusion for APO. Unable to wean off CPAP – just transferred to CCU. Background IHD, HTN, heart failure (1 litre fluid restriction), recurrent UTIs Case study 2 Assessment: What monitoring do you want to inform your Assessment? Airway Inspection – look, listen, feel. Maintaining own. Risk of NIV? Staffing ratio? Breathing What monitoring? Breathing spontaneously, RR – 28bpm SpO2 95% FiO2 0.4. Circulation Warm, well perfused peripheries. HR 130 sinus, BP 100/50 ACUTE RESPIRATORY FAILURE Learning Objectives ▪Define acute respiratory failure (ARF) ▪Classification of ARF ▪Causes & clinical features ARF ▪Mechanisms & features of hypoxaemia ▪Identify the causes of tissue hypoxia ▪ABG assessment of hypoxaemia and hypoxia ▪Mechanisms & features of hypercarbia ▪General treatment of ARF Review of A & P How do we breathe? University of Adelaide 4 University of Adelaide 5 University of Adelaide 6 University of Adelaide 7 University of Adelaide 8 University of Adelaide 9 University of Adelaide 10 University of Adelaide 11 University of Adelaide 12 Recap Definitions ▪Hypoxaemia ▪Deficient oxygenation of arterial blood ▪PaO2 < 80 mmHg ▪Hypoxia ▪Deficient oxygenation of the tissues ABG Analysis PaO2 70 mmHg PH 7.25 PaCO2 51 mmHg HCO3- 24 mEq/L Oxygen delivery- 50% through Invasive ventilation ED presentation- 1st gas PaO2 60 mmHg PH 7.29 PaCO2 32mmHg HCO3- 19mEq/L Lactate 3 Oxygen delivery- Non rebreather Nil urine output since?? ABG’s One more practice- PaO2 200 mmHg PH 7.50 PaCO2 28 mmHg HCO3- 23 mEq/L Oxygen delivery- Nasal Specs 4L Variations VBG Normal Values pH: 7.32-7.43 PvO2: 30-40 PvC02: 41-50 HC03: 19-25 BE: -3-+3 ABG Normal Values pH: 7.35-7.45 PaO2: 80-100 PCO2: 35-45 HCO3: 22-28 BE: -2 to +2 Respiratory Failure - Definition ▪A condition in which the respiratory system fails in one or both of its gas-exchange functions ▪Oxygenation ▪Elimination of Carbon Dioxide (CO2) Acute Respiratory Failure Conventionally defined as: ▪PaO2 < 60 mmHg and/or ▪PaCO2 > 45 mmHg & pH < 7.35, or both. Respiratory Failure The respiratory system consists of two parts: The lungs (gas exchange) The ventilatory pump Failure of one or the other leads to respiratory failure Respiratory Failure Lung failure- Type I Pump failure – Type II Failure to oxygenate Failure to ventilate manifest by manifest by hypoxemia hypercarbia Classification of Acute Respiratory Failure Failure To Oxygenate Failure To Ventilate ▪Hypoxemic (type I) ▪Hypercapneic (type II) respiratory failure respiratory failure ▪Characterised by ▪Characterised by hypoxaemia hypercapnia ▪Normocapnia or hypocapnia Causes of Acute Respiratory Failure Intrapulmonary Extra pulmonary Airway Disease Central Nervous System (CNS) Disorders Lung Disease Disorders of the Peripheral Nervous System & Spinal cord Pulmonary Circulation Neuromuscular Disorders Pulmonary Oedema Diseases/Disorders of the Pleura or Chest Wall Clinical Features of Acute Respiratory Failure Variable ▪Dependant on the underlying cause ▪Dependant on the degree of ▪ hypoxaemia, ▪ hypercarbia ▪ acidosis Clinical Features Dyspnoea, tachypnoea, orthopnoea Inability to speak Use of accessory muscles Intercostal retractions Paradoxical abdominal movement Noisy breathing - crackles, wheezes, stridor Anxiety, restlessness, confusion Lethargy, coma Type 1 Respiratory Failure: Failure to Oxygenate ▪Most common type of respiratory failure ▪Mechanisms of hypoxaemia ▪ Low Fraction of inspired oxygen (FiO2) ▪ V/Q mismatch ▪Diffusion defect Ventilation Perfusion Mismatch (V/Q mismatch) ▪Most common cause of hypoxaemia ▪Normally ventilation equals perfusion ▪As V/Q ratios deviate from normal- gas exchange is impaired Causes of V/Q Mismatch ▪Shunt - Ventilation reduced / perfusion normal ▪Obstructed airways ▪Pneumonia ▪Pulmonary oedema ▪Dead space - Ventilation normal / perfusion reduced ▪Pulmonary embolism ▪Low cardiac output http://emedicine.medscape.com/article/167981-overview Diffusion Defect Impaired diffusion of gases ▪Due to conditions that affect the alveolar-capillary (AC) membrane: ▪Loss of alveolar surface area ▪COPD, pneumonectomy, tumour ▪Thickening of the AC membrane ▪Pulmonary fibrosis & pulmonary oedema ▪Response to O2 depends on the severity of the disease Determinates of Gas Exchange The thickness of the membrane e.g., pulmonary fibrosis The surface area of the membrane e.g., atelectasis The diffusion coefficient of the gas (O2 has a lower molecular weight than CO2 and therefore can be expected to diffuse across the respiratory membrane faster) e.g., temperature The pressure difference between the two sides of the membrane (alveoli and capillary) e.g. The partial pressure of oxygen in the alveoli is about 104 mm Hg, whereas its partial pressure in the blood of the capillary is about 40 mm Hg. Hypoxaemia versus hypoxia ▪Hypoxaemia does not necessarily cause tissue hypoxia ▪Depends on the severity of hypoxaemia ▪A precise PaO2 that will cause hypoxia cannot be identified ▪Affected by various factors ▪Cardiac Output, Haemoglobin, shift in Oxy Haemoglobin Dissociation curve ▪Tissue hypoxia is likely with severe hypoxaemia ▪PaO2 < 45 Markers of Tissue Hypoxia ▪ Increased Lactate ▪ Reduced SVO2 < 65% or ScVO2 < 70% ▪ How do you recognise tissue hypoxia ? Other indicators ? Causes of Hypoxia ▪Hypoxaemic hypoxia ▪Inadequate oxygenation of arterial blood ▪Stagnant hypoxia ▪Inadequate blood flow ▪Anaemic hypoxia ▪Insufficient functional haemoglobin ▪Histotoxic hypoxia ▪Inadequate tissue utilisation of oxygen Hypoxaemic hypoxia ▪Inadequate oxygenation of arterial blood ▪V/Q mismatch, right-to-left shunt, diffusion impairment, hypoventilation and low inspired O2. Stagnant hypoxia ▪Inadequate blood flow ▪May affect the entire body ▪Heart failure, cardiogenic shock ▪May be localised ▪Vascular disease Anaemic Hypoxia ▪Insufficient amount of functional Hb ▪Decrease in concentration of Hb ▪Defect in the ability of Hb to carry O2 Histotoxic Hypoxia ▪Impaired tissue utilisation of oxygen ▪Increased metabolic demands ▪Systemic inflammation, sepsis, shivering, burns ▪Impaired tissue utilisation ▪Sepsis, carbon monoxide & cyanide poisoning Clinical Features of Hypoxia ▪Respiratory ▪Early - Dyspnoea, tachypnoea, hyperpnoea ▪Late - Bradypnoea or agonal breathing ▪Cardiovascular ▪Early - Tachycardia, mild hypertension ▪Late - Bradycardia & hypotension, arrhythmias and Angina Clinical Features of Hypoxia ▪Neurological ▪Headache, restlessness, agitation, confusion, paranoia, combative behaviour ▪Loss of coordination, CNS depression, coma ▪Skin ▪Early - Cool, clammy, diaphoresis ▪Late – Cyanosis Peripheral/Central Other effects of Hypoxia ▪Hypoxic pulmonary vasoconstriction ▪Increased pulmonary vascular resistance ▪Cor pulmonale (right heart failure) ▪Myocardial dyfunction ▪Diaphragmatic fatigue Type 2 Respiratory Failure: Failure to Ventilate ▪Hypercapneic respiratory failure ▪PaO2 > 45 mmHg ▪Usually* hypoxaemia as well ▪Usually caused by hypoventilation Clinical Manifestations of Hypercarbia PaCO2 60 -75mmHg PaCO2 80-100mmHg PaCO2 120-150mmHg Tachypnoea/ Asterixis Anaesthesia Dyspnoea Peripheral Confusion/ DEATH Vasodilation Lethargy Warm peripheries Headache Bounding pulse Coma Other effects of Hypercarbia ▪Displaces alveolar O2 & reduces PaO2 ▪Shifts the Oxy-Haemoglobin Dissociation (OHD) curve to the right ▪Decreases affinity of Hb for O2 ▪Increases unloading O2 to the tissues Who is at risk of CO2 retention? ▪Patients with: ▪ COPD ▪ Severe chronic asthma ▪ Cystic fibrosis ▪ Kyphoscoliosis ▪ Neuromuscular disease ▪ Obesity hypoventilation ▪Most patients with COPD have normal PaCO2 ▪Most admissions with an acute exacerbation of COPD have acute not chronic respiratory failure ▪Distinction has important prognostic & therapeutic implications- consider baseline CO2 levels. Investigations (Type 1 and 2) ▪ABG ▪SaO2, PaO2, PaCO2 ▪A-a gradient, SvO2 ▪pH, bicarbonate, lactate ▪Chest X-Ray Extra investigations (for underlying cause): Electrocardiograph (ECG), haemodynamic monitoring, & echocardiography The Alveolar-arterial gradient (A-a) ▪The difference between the alveolar concentration of oxygen and the arterial concentration of oxygen ▪Normal is approx. 5 - 10mmHg (Young adult, non-smoker) ▪One use of the A-a gradient is in patients with hypoxemia & hypercarbia ▪A-a gradient normal : ▪hypoxaemia is caused by hypoventilation alone (e.g., head injury) ▪A-a gradient abnormal (Raised): ▪ hypoxaemia is complicated by an intrapulmonary disorder e.g., pneumonia affecting transfer of oxygen across alveolar membrane. ▪A-a gradient is calculated as PAO2 – PaO2 https://www.nursingcenter.com/ncblog/august- 2020/calculate-a-a-gradient go to calculator and practice For every decade a person has lived, their A–a gradient is expected to increase by 1 mmHg – a conservative estimate of normal A–a gradient is < [age in years/4] + 4 or age x 0.3 Management of Respiratory Failure ▪Failure to oxygenate ▪Oxygen therapy ▪Maintaining lung volumes using alveolar recruitments strategies ▪PEEP / CPAP / *NHF ▪Failure to ventilate ▪NIV / BiPAP / IPPV General Goals of Therapy ▪Maintain airway patency ▪Optimise oxygen delivery ▪Oxygen therapy, NIV, IPPV ▪Patient positioning, C&DB, pain relief ▪Optimise CO & ensure adequate Hb ▪Minimise oxygen demand ▪Identify & treat cause ▪Prevention of complications Take home messages ▪There are two types of respiratory failure ▪Type 1 - Failure to Oxygenate ▪Type 2 - Failure to Ventilate ▪Hypoxia will kill before hypercarbia ▪Administer oxygen ▪Consider the underlying cause and (if possible) treat/intervene. Type I and Type II RF Type I Type II - Hypoxia – low paO2 -Hypoxaemia AND Hypercapnia -pCO2 is normal or low -Low paO2 and high pCo2 -How is the patient -How is the patient looking? looking? University of Adelaide 52 Case study Maggie is a 25 yo female with no relevant PmHx. She takes no medications besides an oral contraceptive. She presents to ED with mild SOB and chest pain. Her cardiac troponin level is negative. Please see her ECG: University of Adelaide 53 University of Adelaide 54 Please see her CXR University of Adelaide 55 Her SOB worsens. She is now on Nasal Specs 4L (FiO2? ) RR: what do you think? SpO2 94% HR: 110bpm BP: 110/70mmHg (usually low) The team decides to obtain an ABG. University of Adelaide 56 a b c FiO2 36% pH 7.48 paO2 110 pCO2 25 HCO3 24 Interpretation How is the patient? Magic Numbers 35-45! What do we do? pH: 7.35-7.45 PaO2: 80-100 PCO2: 35-45 HCO3: 22-28 University of Adelaide 57 a b c FiO2 35% 50% pH 7.48 7.40 paO2 110 100 pCO2 25 38 HCO3 24 24 Interpretation How is the patient? What do we do? Magic Numbers 35-45! pH: 7.35-7.45 PaO2: 80-100 PCO2: 35-45 HCO3: 22-28 University of Adelaide 58 a b c FiO2 50% 50% 60% pH 7.48 7.40 7.2 paO2 110 100 61 pCO2 25 38 57 HCO3 24 24 24 Interpretation How is the patient? What do we do now? Magic Numbers 35-45! pH: 7.35-7.45 PaO2: 80-100 PCO2: 35-45 HCO3: 22-28 University of Adelaide 59 Maggie does not tolerate NIV as she is very SOB and drowsy. She is quickly intubated. A bedside ECHO is performed where severe RV dysfunction is identified. After stable she is taken to a CTPA where multiple PE are identified. University of Adelaide 60 Treatment - Haemodynamic and respiratory support - Anticoagulation (Heparin, NOACs or warfarin) - Reperfusion treatment - systemic thrombolysis (alteplase/tenecteplase) University of Adelaide 61 Questions? Common Respiratory Disorders Foundations of Critical Care 2024 D.Freer Learning Outcomes Identify the aetiology, pathophysiology, clinical and diagnostic features and management of the following acute respiratory disorders: COPD Pulmonary Embolism Pneumonia Asthma APO will be discussed with Heart Failure Causes of respiratory distress What commonly causes respiratory distress in your patients? Ventilatory problems Obstructive / restrictive Acute / Chronic OR impaired pulmonary ventilation or perfusion Infectious processes Impaired CNS drive Heart failure Metabolic problems Normal during increased activity Take a good history Important in every area, not just the ED Length of time? Onset (gradual vs abrupt)? Position of comfort? Orthopnea? Coughing? Productive? Character? Haemoptysis? Pain? Onset, quality, radiation, severity, duration ? PMHx? Occupational Hx? Current medications & allergies? Focussed assessment Don’t forget the ABCs Signs & manifestations of shortness of breath Cough – recent vs chronic Sputum – colour & amount Fever Chest pain – localised, worse on Haemoptysis – distinguish from haematemesis, epistaxis, maxillofacial Children – head bobbing, nasal flaring, Dyspnoea Causes Cardiovascular… Respiratory… Metabolic… Mechanical… Extra-pulmonary… Acute dyspnoea with no previous heart or lung disease Chronic Lung Disease Obstructive – cannot get the air out Asthma Bronchiectasis Chronic Bronchitis Emphysema Chronic obstructive pulmonary disease (COPD) Chronic obstructive airways disease (COAD) Chronic airway limitation (CAL) Restrictive – cannot get the air in -osis (interstitial pulmonary fibrosis, asbestosis, cystic fibrosis) COPD 5% of the population May present as life threatening respiratory failure Common co-morbidity Often have a combination of chronic bronchitis and emphysema +/- asthma Chronic Bronchitis Clinical diagnosis: “Chronic cough with sputum production for at least 3 months per year for 2 consecutive years” Emphysema Pathological diagnosis: “Destruction of lung units distal to terminal bronchioles leading to abnormal permanent airspace enlargement” Chronic Bronchitis Long-standing inflammation of the lower airways Mucus +++ Infections Wheeze Coughing and mucus for at least 3 months a year for 2 years in a row Chronic Bronchitis Increased mucus secreting cells Productive cough Vital capacity decreased Tidal volume is normal or decreased Alveoli walls not severely affected and diffusion is relatively normal Alveolar ventilation reduced Emphysema Destroys the alveoli Lungs lose elasticity Alveoli become enlarged Emphysema Destruction of the alveolar walls Decreased alveolar surface membrance Pulmonary capillaries decrease in number Increased dead-space ventilation (V>Q) Weakens walls of small bronchioles, causes: Loss of recoil Air trapping Increased residual volume Hypoxia, inflammation, loss of capillaries, and hypercarbia Leads to pulmonary hypertension & cor pulmonale (R heart failure) Alveolar destruction in emphysema Attraction of Smoking inflammatory cells Release of elastase Decreases Inherited Action inhibited by a1-antitrypsin a1-antitrypsin a1-antitrypsin activity deficiency Destruction of elastic fibres No Damage in lung Emphysema Emphysema Smoker history Barrel-chested, AP ratio 1:1 Unable to fully exhale ABG normally shows low PaO2, high PaCO2 and high HCO3 (chronic) COPD Treatment Relieve hypoxia – hypoxia kills faster than hypercarbia Titrate oxygen to SpO2 88-92% Positioning – seated / semi-seated Bronchodilators – salbutamol & Ipratropium – MDI / neb NIV – BiPAP for ventilatory support & alveolar recruitment Corticosteroids Antibiotics ? When? Oxygen & CO2 retention Excessive oxygen administration can lead to hypercapnic respiratory failure in some COPD patients COPD patients with more severe hypoxemia are at higher risk of CO2 retention from uncontrolled O2 administration The same phenomenon has also been described in Severe asthma Community-acquired pneumonia Obesity hypoventilation syndrome Neuromuscular conditions Any patient with chronic respiratory failure may be at risk Oxygen and CO2 retention The traditional theory is that oxygen administration to CO2 retainers causes loss of hypoxic drive, resulting in hypoventilation and type 2 respiratory failure. This is a myth. Patients suffering from COPD exacerbations, regardless of whether they have CO2 retention, generally have HIGH respiratory drive (unless there is impending hypercapnic coma) The real explanation involves: Increased V/Q mismatch (most important) The Haldane effect How oxygen causes CO2 retention V/Q mismatch In COPD, over time patients optimise their gas exchange by hypoxic vasoconstriction (i.e. they reduce blood supply to areas where gas exchange does not occur well). Administering a high level of oxygen will increase oxygen tension, reducing hypoxic pulmonary vasoconstriction. Results in increased perfusion to areas where ventilation is poor Increased dead space ventilation & higher PaCO2 due to overall lack of ventilation at the alveolar level How oxygen causes CO2 retention The Haldane effect: Describes the difference in the quantity of carbon dioxide carried in oxygenated and deoxygenated blood. CO2 has a higher affinity for deoxygenated Hb than oxygenated Hb Giving a patient high levels of oxygen will decrease the affinity of CO2 for Hb. Carbon dioxide will be displaced by oxygen, resulting in a higher PaCO2. For patients who cannot increase their minute ventilation and blow off this CO2 (i.e. those with COPD), the Haldane effect accounts for about 25% of their total PaCO2 increase. What do we do? In patients with COPD, hypoxic pulmonary vasoconstriction is the most efficient way to optimise the V/Q ratio to improve gas exchange. This physiological mechanism is counteracted by oxygen therapy and accounts for the largest increase of oxygen-induced hypercapnia. A titrated oxygen therapy to achieve saturations of 88%-92% is recommended in patients with an acute exacerbation of COPD. “permissive hypoxemia” to avoid hypercarbia In extremist, always give oxygen Hypoxia kills faster than hypercarbia Pneumonia – ‘Old mans best friend’ Definition: Acute inflammation of the lung parenchyma 10% mortality rate in Australia 2nd most common hospital-acquired infection Highest mortality rate for HAI Common cause of death in elderly Pneumonia Classification Classified into groupings. Community Acquired pneumonia Hospital Acquired (nosocomial) pneumonia Pneumonia in the immuno-compromised patient Aspiration pneumonia Community – acquired pneumonia Large majority of presentations to ED Typical (common bacteria) / atypical (uncommon bacteria) Mostly managed in the community Hospital acquired pneumonia (nosocomial) Pneumonia acquired during a hospital stay (in hospital after 72 hours) The leading cause of death among hospital acquired infections Broad range of causative organisms some being antibiotic resistant Pneumonia Risk Factors Advanced Age >65years Smoking history Stroke / swallowing difficulty URTI Tracheal intubation Prolonged immobility Immunosuppressive therapy Non-functional immune system Malnutrition Dehydration Co-Morbidities I.e; CAL, Diabetes, CHD Pneumonia Pathophysiology Inflammatory response Lung defence mechanisms overwhelmed (COUGH/ CILIARY ACTION) Infective agent penetrate the lower respiratory tract Inflammation and colonization Inflamed alveoli fill with exudate Increased capillary permeability Diffusion of O2 and CO2 altered A-a gradient increased or decreased? Pneumonia Pathophysiology Bacterial pneumonia can lead to significant V/Q mismatch with significant increase in pulmonary shunting. Leads to hypoxia ARF / pleural effusions can develop Causes of Pneumonia Bacteria Viruses Mycoplasma’s Fungal agents Protozoan Aspiration of food, fluids, vomitus Inhalation of toxic or caustic chemicals, dust, smoke, or gases Pneumonia - Typical (Bacterial) Gram positive Strep. Pneumoniae (most common) Staph. Aureus Gram negative (highest mortality) e.g. haemophilus influenza bacillus, klebsiella frequent cause of nosocomial pneumonia Esp. in the critically ill due to altered defences, intubation, poor oral hygiene, suctioning… Pneumonia - Atypical Influenza virus strains Can rapidly progress to picture of ARDS. Mycoplasma (younger adults, teenagers) PCP ( severely immuno-suppressed patients) Pneumocystis carinii pneumonia – CXR (diffuse bilateral interstitial shadowing), Pneumonia Clinical Signs Fever / rigors / sweats Dyspnoea, headache, fatigue Tachycardia Low saturations, cyanosis (severe , late) Dull percussion over area of consolidation Crackles, bronchial breathing Altered mental state Sputum Pneumococcal – bloody or rust coloured Pseudomonas – green Haemophilus – green Klebsiella – currant jelly Pneumonia Management Antibiotic therapies commence as soon as possible broad spectrum until the specific organism is isolated Respiratory support a needed Oxygen to correct hypoxia Enable expectoration – Chest Physiotherapy Bronchodilator therapies – open airways/loosen consolidation Fluids and Electrolyte management Nutritional support Prevention – Tracheal suction IPPV pt Handwashing Pulmonary Embolism Blockage of the pulmonary artery by: Air Blood clot Other foreign material Thrombus can be loosened by: Trauma Clot dissolution Sudden muscle spasm Intravascular pressure changes or alterations in peripheral blood flow Most common is dislodgement of emboli from deep veins in the thigh and pelvis Pulmonary embolism Risk Factors: Immobility Venous injury Hypercoagulability Pregnancy / post-partum Oral contraceptive tablet Multi-trauma (long bone #s) IVDU Symptomatic PE occurs in approximately 50% of untreated DVT. Pulmonary embolism Consider a PE in any patient who has cardio-respiratory problems that cannot be otherwise explained; look for risk factors Wells’ criteria for PE: - Clinical signs - Probability of PE - HR>100 - Immobilisation (3 days) or surgery in past 4 weeks - Previous PE - Hemoptysis - Malignancy with treatment in last 6 months or palliative Pathophysiology of PE Venous stasis Vessel Injury Hypercaogulability Thrombus Formation Dislodgement of Portion of Thrombus Occlusion of pulmonary circulation Increase in dead-space ventilation Hypoxic vasoconstriction Decreased surfactant Release of neuro-hormonal inflammatory substances Pulmonary oedema Atelectasis Pathophysiology of PE Tachypnoea Dyspnoea – most common Chest Pain Increased Dead Space V/Q imbalances Decreased PaO2 Pulmonary infarction Decreased cardiac output Systemic hypotension Shock Pulmonary Embolism Treatment Closely observe – continuous Sp02 and cardiac monitoring. Oxygen Fluid loading Anticoagulation Thrombolytics (massive PE) ETT & IPPV Inotropes Surgery Asthma Inflammatory disease Episodic periods of reversible bronchospasm, mucous plugging and air trapping Epidemiology 1 in 10 Australians have asthma – approx. 2.8 million people More common in females after adolescence Approximately 500 deaths per year ‘Thunderstorm Asthma’ 2016 unusual ‘cluster of allergic asthma flare-ups’ with springtime thunderstorms High grass pollen count for first 30 min of storm Thousands of emergency calls 9 deaths, state of emergency in Victoria Asthma Pathophysiology Chronic inflammatory disorder of the lower airways Mast cells, eosinophils, & T lymphocytes mobilised Bronchospasm to cause inflammation Many and varied triggers Inflammation leads to increase in airway responsiveness: Bronchial hyper-responsiveness Airway remodelling occurs secondary to the inflammation: Thickening and Fibrosis of brochioles Bronchial Edema Increased Mucus Production Asthma Pathophysiology Mediated by immune system Mast cells activated by allergens Bronchoconstrictive substances (e.g. histamine) released Eosinophils release protein that damages the epithelium T cells stimulate IgE Acute broncho constriction (contraction of smooth 2 muscle) 1 Swelling of airway wall Secondary to inflammation 3 Chronic mucous plug formation (mucous hyper secretion & inflammatory exudate) Direct & indirect airway Hyper responsiveness Asthma triggers Extrinsic Type 1 – allergic (e.g. pollen) Type II – prolonged exposure (e.g. mites) Commonly accompanied by other allergies (eczema & allergic rhinitis) Intrinsic attacks (no known cause in 30% - ? Exercise) E.g. infection, emotional stress, anxiety, coughing or laughing Most episodes occur following respiratory infection Asthma patient history Recent viral infection? Exposure to known allergen? Activity prior to presentation? High risk History Childhood onset Steroid dependence Previous ICU admissions Signs of severe asthma Classic triad of cough, wheeze, and dyspnea Difficulty speaking – Single words Rib retractions Pulse > 130, Resps > 30 SaO2 < 90% on air Altered mental status – Lethargy/confusion? Signs of life-threatening asthma Inability to speak Silent chest Sweating & vomiting Panic SaO2 < 90% with O2 Golden Rule All that wheezes is not asthma Pulmonary Edema Allergic reactions Pneumonia Foreign body aspiration Asthma Treatment Bronchodilators 12x salbutamol & 8 Atrovent via MDI with spacer Can give 5mg salbutamol & 500mcg ipratropium neb Repeat / continuous bronchodilators May need K+ replacement Oxygen – titrate to 93-95% If no response, add IV Magnesium Sulfate Systemic corticosteroids – 100mg Hydrocortisone Ventilate………. NIV or invasive…….Difficult Zeep & permissive hypercapnea Increased I:E ratio, longer expiratory time Continue to treat underlying cause Questions? We are sitting in the shade today because someone planted a tree a long time ago Oxygen Therapy Foundations of Critical Care 2024 D.Freer Learning Objectives Understand the properties of oxygen Safety aspects related to oxygen Physiological effects of high oxygen administration CO2 retainers Oxygen delivery equipment available and how to determine delivery method When would you administer oxygen and when wouldn’t you? CHECK IT! At the beginning of every shift! And after a critical transport What is all the fuss about Oxygen? Delivery of oxygen is dependent on 4 variables: CO, Hb, SaO2, and PaO2 1. What changes Cardiac Output? HR SV Preload Contractility Afterload 2. What changes functional Hb – oxygen carrying capacity? Blood loss – acute / chronic Haematological conditions, e.g. sickle cell anaemia Iron levels 3. What changes SaO2 – our functional oxygen? Shifting of the oxy-haemaglobin dissociation curve – pH, CO2, Temperature, 2-3 DPG Diffusion across alveolar-capillary membrane FiO2 3. What Changes PaO2 – our oxygen reserve? Diffusion across alveolar-capillary membrane FiO2 Oxygen administration changes FiO2 and only affects two of oxygen delivery variables: SaO2 & PaO2 The Oxygen Cascade from Atmosphere to Mitochondria Atmosphere to Alveoli Atmospheric air: 21% oxygen = PaO2 of 159 mmHg Airway gas mixture: Diluted by water vapour = PaO2 of 149 mmHg Alveolar gas mixture: Diluted by CO2 = PaO2 of 99 mmHg Also, some oxygen is taken up by the capillaries, which decreases the alveolar PaO2 Capillary to Mitochondria Pulmonary endcapillary blood Essentially the same as alveolar gas, in health PaO2 approx. 99mmHg Arterial blood Diluted by venous admixture= PaO2 of 92 mmHg The difference between alveolar and arterial gas is the A-a gradient Normal A-a gradient is 7mmHg in the young, and 14mmHg in the old Tissue oxygen tension Drops due to diffusion distance Varies from tissue to tissue, but is usually around 10-30 mmHg Mitochondrial oxygen tension Drops due to diffusion distance Usually between 1-10 mmHg At sea level Atmospheric pressure = 760mmHg Oxygen makes up 21% of this Therefore, the partial pressure of oxygen at sea level is 760 x 0.21 = 159mmHg That’s why the oxygen cascade start with a PaO2 of 159mmHg (often rounded to 160mmHg) Change in Altitude Higher altitude Decrease in atmospheric PaO2 Affects the whole oxygen cascade Decrease in oxygen dissolved in blood (PaO2) Lower altitude (below sea level & hyperbaric) Increase in atmospheric PaO2 and oxygen dissolved in blood. Does the FiO2 change? No, the molecules are just further apart… Effect of atmospheric pressure Atmospheric pressure ATA (mmHg) FiO2 PatmO2 PAlvO2 0.5atm 18000 ft 380mmHg 0.21 80mmHg ~50mmHg 1 atm Sea Level 760mmHg 0.21 160mmHg ~100mmHg 2 atm 10mbsw 1520mmHg 0.21 320mmHg ~200mmHg Change in Altitude The higher up a mountain we go, the further apart the oxygen molecules are. High altitude places stress on people with underlying cardio / respiratory conditions. If we live in higher areas for long times, develop compensatory mechanisms Increased RBC Increased lung capacity Reason for high altitude training What about in an aeroplane? Oxygen Discovered in 18th Century (1772-1780) Named from the Greek (oxy gene – “acid former”) Oxygen reacts to produce oxides (e.g. sulphur, carbon, aluminium, and phosphorous) Earth’s most plentiful element by weight (46%) Properties of Oxygen Colourless, odourless, tasteless Soluble in solution Essential for life – aerobic metabolism Liquefies at -183 degrees Celsius Vigorously supports combusion Oxygen Cylinders Manufactured in accordance with Australian Standards Colour coded White Body / white shoulder Labelled “Oxygen” No agreed international standard for O2 cylinders Filled to 16,300kPa at 15o Celcius In most critical care areas have plumbed oxygen Care of oxygen cylinders Store in a cool well ventilated area Keep away from ignition sources Heat, flammable liquids & gases Patient’s going for a ciggie Keep free from oil or grease Secure upright with restraints Should be refilled if below 5,000 kPa Know how to replace cylinder Open the valve slowly Indications for Oxygen Administration Correction or prevention of hypoxemia SaO2< 90% & PaO2 < 60mmHg Respiratory Failure Cardio-respiratory arrest Shock Severe Trauma Acute myocardial infarction with low SpO2 Anaesthesia / procedural sedation Indications in the absence of hypoxemia Decreased oxygen carrying capacity Carbon monoxide poisoning Severe anaemia / sickle cell disease Debatable – Promote reabsorption of air in body cavities Air embolism, pneumothorax Decompression sickness Explanation is decrease the partial pressure of nitrogen in the lungs and create a diffusion gradient for nitrogen to be exhaled. Goals of oxygen therapy Reduce or correct hypoxemia Reduce the bodies compensatory mechanisms Patient will be less SOB, reduce the work of breathing Increased WOB can account for up to 40% of total oxygen consumption Reduce tachycardia Reducing myocardial oxygen demand Effectiveness of Oxygen Therapy Depends on the cause of hypoxemia and hypoxia Shunt (VQ) Ventilation is ok Issue with perfusion Physiological in the trachea Pathological – PE Oxygen administration generally more effective as ventilation is ok Oxygen Delivery Systems Low flow / Variable performance systems Oxygen flow rate is less than the patient’s inspiratory flow Do not provide all the gas necessary to meet inspiratory demand Room air entrained to make up the difference Oxygen diluted with room air resulting in variable FiO2 Higher minute ventilation = more entrained room air = lower FiO2 with same O2 flow rate Peak inspiratory Oxygen Entrained air flow flow rate 30 L/min 10 L/min 20 L/min 60 L/min 10 L/min 50 L/min Oxygen Delivery Systems High flow / Fixed performance systems Gas flow rate > patient’s inspiratory flow rate Gas flow sufficient to meet patient’s demand Delivers a fixed FiO2 not affected by the patients ventilatory pattern Low-flow / variable performance systems Nasal specs Hudson mask Tracheostomy mask Reservoir bag masks Manual resuscitators Nasal Cannula Inexpensive, comfortable FiO2 will be less in patients who Oxygen flow rate FiO2 1 L/min 0.24 are SOB 2 L/min 0.28 FiO2 may be reduced in mouth 3 L/min 0.32 breathers 4 L/min 0.36 Simple face mask (Hudson mask) Provides 100-200ml reservoir Higher concentration of oxygen Some rebreathing of CO2 A minimum of 6LPM is required for all face masks to flush expired Oxygen flow rate FiO2 CO2 6 L/min 0.50 FiO2 reduced with increased 8 L/min 0.55 WOB, poor seal, tolerance 10 L/min 0.60 12 L/min 0.65 Unable to eat, dries mucosa 15 L/min 0.70 Tracheostomy mask Similar to a simple face mask Delivery is dependent on the status of the cuff Why? Requires humidification Why? Non-rebreather face mask Contain a 750 ml reservoir bag that stores O2 during expiration On inspiration Valves on the exhalation port seal against the mask The patient draws pure O2 from the reservoir bag Increases O2 concentration On exhalation Valve between mask & reservoir bag prevents expired gases entering the reservoir bag Expired gas exits through exhalation ports One or two valves on exhalation ports of the face mask Non-rebreather masks Theoretically can deliver up to FiO2 1.0 In reality, the FiO2 is nearer 0.6-0.8 Room air entrained as no tight seal Adjust flow rate so reservoir bag does not collapse Temporising measure – reserved for critical care areas / pre-hospital / procedural sedation / anaesthesia Manual resuscitation bag Self-refilling, non-rebreathing resuscitators Normally disposable Can deliver very close to FiO2 of 1.0 Different sizes for adults and children Only inflate approx. 1/3 volume of the ventilation bag If cannot intubate, try at least to ventilate What do you do if you cannot intubate & cannot ventilate? Pocket Mask Expired air – FiO2 16% O2 @ 10l/min – FiO2 0.5 High flow / fixed performance systems Venturi mask Nasal high-flow NIV IPPV These will be discussed more in subsequent presentations Venturi Mask Uses the venturi principle O2 flow rate is supplemented by entraining room air O2 flow rate @ 6-8 l/min total flow 40-60 l/min Delivers a fixed FiO2 How a venturi mask works O2 is forced through a constriction The velocity of gas flow increases This creates a sudden drop in pressure beyond the constriction Air is entrained via holes in the bottom of the mask The size of the orifice & flow rate determine the concentration O2 FiO2 O2 flow Total gas flow 0.24 4 L/min 104 L/min 0.28 6 L/min 66 L/min 0.35 8 L/min 48 L/min 0.40 8 L/min 32 L/min 0.60 12 L/min 24 L/min Benefits of Venturi Mask Accurately delivers a prescribed FiO2 Gas flow is high enough to meet peak inspiratory demands High flow rates eliminate rebreathing of CO2 Since room air is entrained humidification is not essential Best means of managing patients with chronic COPD Delivers predictable FiO2 Minimises rebreathing CO2 Flow & Concentration Flow is not the same as concentration Low-flow masks can deliver high FiO2 (e.g. resuscitation bag) High-flow masks can deliver low FiO2 (e.g. venturi mask) Which Device? Nasal cannula Normal vital signs, slightly low SpO2, long-term O2 therapy Face masks & non-rebreather masks Higher concentration required / acutely ill patients Delivering an accurate FiO2 not necessary High flow devices Controlled O2 therapy required Exacerbation of COPD (Venturi) Humidification o Room air is 20 C with a relative humidity of 50% Medical oxygen is both cold & dry 10-15 º Celsius Relative humidity is zero Potential for? Under humidification Mucus thickens Reduction in Cilia movement Leads to bacterial colonisation / increased risk of infection Atelectasis from mucus plugging small airways Reduced lung compliance and increased work of breathing Respiratory failure Patients at risk Thick tenacious sputum – bronchitis Difficulty expectorating Neuromuscular dysfunction Chest wall abnormalities Smokers Chronic lung disease Dehydration Elderly High FiO2 Humidification & risk infection Humidification Hot water humidifiers – e.g Fisher & Paykel “gold standard” Humidifies air with water vapour The airway naturally uses water vapour Water vapour does not transport bacteria or viruses into the lungs Reduces the likelihood of pathogens entering the lungs More efficient Inspired relative humidity of 100% at 37oC (bypassed airway) Inspired relative humidity of 85% at 34oC (mask) Heat & Moisture Exchangers Exhaled gases pass through HME Nursing Care of HME Change every 24 hrs Heat & moisture retained Care with nebulised Heat & moisture added with next inspired breath medications Less efficient than hot water humidifiers Can saturate with nebulised medication No risk of thermal injury or overhydration Reduction in Small increase in airway resistance & dead space effectiveness Becomes a barrier to Contra-indications gas exchange Copious secretions Small tidal volumes Dead space may compromise ventilation CO2 Large tidal volumes Ability to humidify gases may be compromised Unwelcome effects of oxygen Arterial spasm Oxygen toxicity Pulmonary oxygen toxicity CNS oxygen toxicity Retrolental fibroplasia (blind) Absorption atelectasis Worsening CO2 retention Under humidification Fire hazard… Pulmonary oxygen toxicity Low dose prolonged administration of O2 FiO2 >0.6 for > 24 hours Believed to be due to toxic O2 free radicals Causes Mucociliary function depressed Endothelial cell damage causes interstitial oedema Decreased macrophage activity Reduced surfactant – reduced surface tension & collapse Decreased compliance Decreased diffusion capacity Pulmonary Oxygen toxicity Signs & Symptoms Shortness of breath Substernal pain Deteriorating gas eschange Poor lung compliance Chest x-ray changes Sound familiar? Difficult to differentiate between pulmonary oxygen toxicity and a deterioration in a pre- existing lung condition Absorption Atelectasis Develops in patients with high FiO2 N2 is the most abundant gas in the alveoli The pressure exerted by N2 splints the alveoli open FiO2 1.0 “washes out” all the N2 Leaves only O2 which is rapidly absorbed alveolar collapse Develops in < 1 hr worsening hypoxemia THINK ABOUT THIS IN THE ED!!! Oxygen and CO2 retention The traditional theory is that oxygen administration to CO2 retainers causes loss of hypoxic drive, resulting in hypoventilation and type 2 respiratory failure. This is a myth. Patients suffering from COPD exacerbations, regardless of whether they have CO2 retention, generally have HIGH respiratory drive (unless there is impending hypercapnic coma) The real explanation involves: Increased V/Q mismatch (most important) The Haldane effect How oxygen causes CO2 retention V/Q mismatch In COPD, over time patients optimise their gas exchange by hypoxic vasoconstriction (i.e. they reduce blood supply to areas where gas exchange does not occur well). Administering a high level of oxygen will increase oxygen tension, reducing hypoxic pulmonary vasoconstriction. Results in increased perfusion to areas where ventilation is poor Increased dead space ventilation (V>Q) and higher PaCO2 due to overall lack of gas exchange at the alveolar level How oxygen causes CO2 retention The Haldane effect: Describes the difference in the quantity of carbon dioxide carried in oxygenated and deoxygenated blood. CO2 has a higher affinity for deoxygenated Hb than oxygenated Hb Giving a patient high levels of oxygen will decrease the affinity of CO2 for Hb. Carbon dioxide will be displaced by oxygen, resulting in a higher PaCO2. For patients who cannot increase their minute ventilation and blow off this CO2 (i.e. those with COPD), the Haldane effect accounts for about 25% of their total PaCO2 increase. What do we do? In patients with COPD, hypoxic pulmonary vasoconstriction is the most efficient way to optimise the V/Q ratio to improve gas exchange. This physiological mechanism is counteracted by oxygen therapy and accounts for the largest increase of oxygen-induced hypercapnia. A titrated oxygen therapy to achieve saturations of 88%-92% is recommended in patients with an acute exacerbation of COPD. “permissive hypoxemia” to avoid hypercarbia In extremist, always give oxygen Hypoxia kills faster than hypercarbia Oxygen delivery O2 delivery depends on more than just O2 therapy Ventilation Diffusion of O2 into the blood Binding with haemoglobin (Enough carriers, ability to bind) Adequate cardiac output (HR, preload, contractility, & afterload) Diffusion into the cell Nursing Care Mouth care/ nasal care Pressure area care – observe ears and nares Ensure treatment/ medication order unless it is an emergency Oxygen after all is a medication….. And just like other medications has positive and negative effects. Take home messages Overall goal of oxygen therapy is to avoid tissue hypoxia The devices are different and terms are used interchangeably Faced with a hypoxic patient ALWAYS think Oxygen first Long term oxygen therapy is not without side effects Consider different approaches for the chronic CO2 retainer when planning oxygen therapy Questions? Foundations of Critical Care – Krystle Halls NIPPV NIPPV – Non-Invasive Positive Pressure Ventilation Indications Contra- Indications Principles Methods Complications Nursing Care University of Adelaide 2 Indications Type 1 (CPAP) and 2 (BIPAP) respiratory failure Exacerbation of COPD Atelectasis Acute Pulmonary Oedema (Cardiogenic / non cardiogenic) Pneumonia Ventilatory (pump) failure – Chest wall deformity – Neuromuscular disease Weaning from Invasive Mechanical Ventilation University of Adelaide 3 Contraindications Life-threatening hypoxemia (PaO2