Polymers for Engineering Applications PDF
Document Details
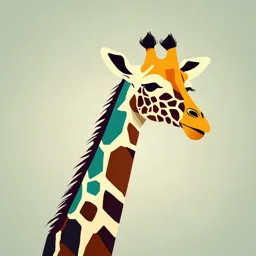
Uploaded by BetterDirac
Tags
Summary
This document provides an overview of polymers, including their types, monomers, polymerization, and applications. It details the properties and characteristics of different types of polymers, such as their structures, molecular weights, and functionality.
Full Transcript
UNIT 3 Polymers for engineering applications Polymers It is defined as a giant or macromolecule formed by the repeated union of several simple molecules called monomers. Mainly there are two types of polymers: 1) Natural polymers: Ru...
UNIT 3 Polymers for engineering applications Polymers It is defined as a giant or macromolecule formed by the repeated union of several simple molecules called monomers. Mainly there are two types of polymers: 1) Natural polymers: Rubber, resins, cellulose etc. 2) Synthetic polymers: Nylon, PVC, Bakelite etc. Monomer It is defined as simple molecule with two or more bonding sites through which it forms covalent linkages with other monomer molecules to form the macromolecule. Examples: Note: Monomers are thus building blocks of polymers. All simple molecules cannot behave as monomers but only those with two or more bonding sites can act as monomers. Thus molecules like ammonia, water, ethanol etc. are not monomers alkenes, vinyl chloride, adipic acid, glycol with two bonding sites act as monomers. The repeating chemical units (monomers) are covalently linked to each other in a macromolecule. The properties of a polymer are entirely different from those of monomers from which it is formed. The molar mass of polymers vary from 103 to 107. Polymerization The chemical process by which monomers are converted into polymers is known as polymerization. To convert monomer into polymer, small quantity of chemicals known as initiators are needed. Temperature and pressure should be maintained. Ethylene (ethane) monomer Polyethylene (polythene) 1 Vinyl chloride Polyvinyl chloride (PVC) Degree of polymerization (DP) The number of repeating units present in a polymer is called degree of polymerization. Here ‘n’ is the degree of polymerization Note: when the value of ‘n’ is very large that is in the range of hundreds or thousands the polymers are called high polymers. For low values of ‘n’ i.e.,less than 10, the polymers are called oligomers in general and more specifically as dimers (n=2), trimers (n=3), tetramers (n=4) and so on. The DP can also be used to compute the molecular weight of polymer. The molecular weight of a polymer is the product of the number of repeat units (DP) times the molecular weight of repeat unit (monomer) i.e. Molecular weight of polymer = DP × molecular weight of monomer Ex: If the DP of polystyrene is 1000 its molecular weight is calculated as {styrene CH2=CHC6H5} Molecular weight of polymer = DP × molecular weight of monomer Molecular weight of polystyrene = DP × molecular weight of styrene = 1000 × 104 =1,04,000 Functionality The total number of functional groups or bonding sites present in a monomer molecule is called the functionality of the monomer. Depending on the number of sites the monomers are called bifunctional, trifunctional or polyfunctional. A simple molecule should possess at least bifunctionality which is the basic requirement to qualify as a monomer and to undergo polymerization. All double bonded compounds example CH2= CH2 (ethane), CH3-CH= CH2 (propene), CH2= CHCl (vinyl chloride) have two bonding sites hence their functionality is two and are bifunctional. 2 HOOC-(CH2)4-COOH (adipic acid) and NH2-(CH2)6- NH2 (hexamethylene diamine) functionality is two because each contains two reactive functional groups. Molecular weight of polymers During the formation of polymers, different polymers have different degrees of polymerisation i.e. they have different chain lengths. Thus, molecular masses of individual macromolecules in a particular sample of polymer are different. Hence, an average value of the molecular weight is taken. There are two ways through which average molecular weight can be calculated, 1. Number -average molecular weight: (Mn) 2. Weight -average molecular weight : (Mw) Number-average molecular weight (Mn): It is determined by measuring the colligative propertiessuch as freezing point depression, boiling point elevation, osmotic pressure and lowering of vapour pressure. It is defined as total mass of all the molecules in a polymer sample divided by total number of molecules present. 𝑁1𝑀1+𝑁2𝑀2+𝑁3𝑀3+⋯. Mn = 𝑁1+𝑁2+𝑁3+⋯ Where Ni is the number of molecules of mass Mi. Number average molecular weight assumes that each molecule makes an equal contribution to polymer properties regardless of size or weight. Weight-average molecular mass (Mw): It is determined by light scattering, ultra- centrifugation and sedimentation technique which measures molecular size. M W gives representation to various molecular species in proportion to their weight in the given material. It is defined as mass obtained when sum of the products of total mass of groups of molecules (having different molecular masses) and their respective molecular masses is divided by total mass of all the molecules. MW WiMi Wi Where Wi is the weight fraction of the molecules whose mass is M i. We have Wi = NiMi MW N M M i i i N M i i 2 N M i i N M i i 3 𝑁1 𝑀12 +𝑁2𝑀22 +𝑁3𝑀32 …… Mw = 𝑁1𝑀1+𝑁2𝑀2+𝑁3𝑀3…… It is the averaging process molecular weight of each individual species is multiplied by weight and not by number. Poly Dispersity Index (PDI): It is the ratio of the weight-average molecular weight (MW) to the number- average molecular weight (Mn). 𝑀𝑤 PDI = 𝑀𝑛 Note: In general we have two different types of polymeric substances 1) Polymers whose molecules have same or narrow range of molecular masses. Such polymers are said to be monodisperse. 2) Polymers which have wide range of molecular masses. Such polymers are called polydisperse. Significance of PDI: In order to have an idea about the homogeneity of a polymer, we use the term called PDI. For natural polymers, PDI is usually equal to one showing that they are monodisperse and molecular weight distribution is homogenous. Synthetic polymers generally have PDI>1 showing that they are polydisperse and molecular weight distribution is less homogenous. Note: In polymers, if chains are closely packed in an orderly manner and oriented in a fixed direction then it is said to be a crystalline polymer. If chains are randomly distributed and jumbled then polymer is called as amorphous polymer. But no polymer is purely crystalline or amorphous. Crystalline polymers are hard and structured, like a diamond. Their molecular chains are locked against each other, which means they are more likely to break than they are to bend when placed under pressure. This tight configuration doesn’t really lend itself well to crosslinking. On the other hand the molecular chains of amorphous structures have more room to move around each other, which makes them more likely to crosslink. 4 The physical and chemical properties of a polymer will depend on the degree of crystallinity. Degree of crystallinity varies from polymer to polymer and depends mainly on its structure. All crystalline polymers have high degree of crystallinity and less amorphous areas similarly all amorphous polymers have low degree of crystallinity and high amorphous areas. Although it is possible to create a 100% amorphous structure, it is impossible to create a 100% crystalline structure as crystalline polymers always contain a decent number of amorphous areas. Hence crystalline polymers containing amorphous areas are called as Semi-crystalline polymers. The majority of the polymers are amorphous and semi-crystalline polymers. Glass transition temperature (Tg) and Parameters influencing Tg All amorphous polymers when cooled below a certain temperature become stiff, hard, brittle and glassy but above this temperature they are soft, flexible and rubbery. The temperature at which a polymer abruptly transforms from glassy state to rubbery state is called as Glass transition temperature. 5 Parameters Influencing Tg Value are Flexibility, intermolecular forces, branching & crosslinking, molecular weight, plasticizers and stereoregularity (tacticity). Flexibility A free rotational motion of the polymer chain imparts flexibility to the polymer. The higher the freedom to rotate, the more flexible are the polymer chain segments and hence lower is the Tg value. Linear polymers made up of C-C, C-N or C-O bonds with high degree of freedom for rotation have lower Tg values. The presence of aromatic or cyclic structures or bulky side groups in the chain backbone hinders the free rotation of segments, lowers the flexibility and increases the Tg value. Example: Polyethylene polymer has Tg value -110oC (only single bonds), polybutadiene has -85oC (presence of double bonds), polystyrene has 100oC (bulky side group {aromatic side group}). Intermolecular forces Inter molecular forces determine the strength with which polymer chains are held together in a polymer sample. Non –polar polymer chains are attracted to each other by weak Vander Waal attractions and therefore such polymers will have low Tg. If polar groups are linked to carbon atoms of the polymer chain, the dipole- dipole attractions will affect the segmental mobility resulting in a higher Tg. If polymer chains are linked by hydrogen bonds, intermolecular attractions are very strong and the Tg will be high. For example, in hydrocarbon polymers, only Van der Waals forces hold the polymer chains together and the chain segments can move easily. Thus such polymers have low Tg value. Eg. Polythene – Tg = -110◦ C. Nylon 6 having similar structure as that of polythene has higher Tg value 50 oC because, polymer chains are held together more strongly by dipoles as well as intermolecular hydrogen bonding, and their movement is restricted, thus increasing Tg value of the polymer. Similarly for Nylon 6,6 (Nylon 66) has Tg = 57◦ C. 6 Branching and Cross linking A small amount of branching will reduce the value of Tg, because the free volume increases with branching and thus decreases the Tg. Higher degree of branching put a restriction on segmental motion and hence Tg increases. When the chains are crosslinked through covalent bonds there is almost total immobility of chains and hence high Tg. Examples: Polyethylene Tg= -110oC (no branches), polystyrene Tg =100oC. (aromatic side group) and Poly methyl styrene Tg =170 oC (two side groups). Molecular weight The Tg value of a polymer is influenced by its molecular weight. The Tg, value of all polymers, in general increases with molecular weight up to 20,000 as the attractive force between the polymer chain increases and above which the effect is negligible. Under high molecular mass, long polymeric chains coil and entangle with one another which restricts free mobility of the chain increasing Tg. Addition of plasticizers Plasticizers are low molecular weight non-volatile substances, which when added to polymer improves flexibility, processibility and resilience. Therefore presence of plasticizers reduces the Tg of polymers. Example: Addition of diisooctylphthalate (plasticizer) to PVC reduces its Tg from 80oC to below room temperature. Other plasticizers are camphor, triceryl phosphate, dimethyl phthalate and triphenyl phosphate 7 Note: Plasticizers molecules penetrate into the polymer matrix, occupying the space between the polymer molecules and thereby reducing the intermolecular forces between them. Without plasticizers many of the commercially useful polymers such as PVC and nylon would have been useless as their Tg values are above ambient temperature and are brittle at normal temperature Stereo-regularity (Tacticity) In a polymer based on the position of the substituent group Z, three stereoisomers are possible. Isotactic: If all the substituents lie on one side of the chain, then the configuration is called as isotactic. Syndiotactic : If the substituents alternate from one side to another in a regular manner then the configuration is syndiotactic. Atactic : Random arrangement of substituent group. Isotactic Syndiotactic Atactic Based upon their stereoisomers, the Tg can be related considering following examples POLYMER Tg Isotactic Tg Syndiotactic Tg Atactic PP 0◦ C -8◦ C -20◦ C PMMA 130◦ C 120◦ C 100◦ C Hence it can be concluded that isotactic polymer has higher Tg than syndiotactic whose Tg is in turn greater than atactic isomers (Tg of isotactic > syndiotactic > atactic) Significance of Tg: Tg can be used to evaluate the flexibility of a polymer and predict its response to mechanical stress. Many polymers show an abrupt change in their physical properties at their glass transition temperature. Coefficient of thermal expansion, heat capacity, refractive index, mechanical damping, modulus of elasticity and electrical properties at Tg determine the usefulness of a polymer over a temperature range. Polymeric materials are subjected to different processing operations such as moulding, calendering and extrusion. Knowledge of Tg is useful in choosing appropriate temperature for fabrication of polymer materials. 8 Structure-Property relationship of polymers The structure of a polymer has profound influence on some of the properties of polymers. The properties such as crystallinity, tensile strength, elastic nature and chemical resistance are largely dependent on the structure of the polymers. Crystallinity: Polymers invariably contain both crystalline and amorphous regions. The percentage of crystallinity of a polymer depends on its structure [linear, branched with large pendant groups in polymer chain] and configuration (stereo regularity) Linear polymers, homopolymer and the presence of polar groups increases the crystallinity whereas branched, copolymer and bulky side groups decreases the crystallinity The polymers having high degree of crystallinity exhibit high tensile strength, impact resistance, high density and sharp melting point (Tm) Example: Polyethene (linear) is more crystalline than polystyrene (bulky side groups) or polymethylacrylate (more branched). Nylons are more crystalline due to hydrogen bonding. Polymers like isotactic and syndiotactic polypropylene have a regular structure and are more crystalline than atactic polystyrene where substituents are arranged in a random manner. Tensile strength: Tensile strength is highly influenced by molecular weight of the polymers. Tensile strength and impact strength increase with molecular weight up to ~20,000 and then becomes constant. Every polymer has a threshold molecular weight (~DP=30) value below which it does not possess useful strength. High molecular weight polymers are tough, hard, heat resistant and difficult to process. The melt viscosity of the polymer initially shows a gradual increase with the molecular mass and a steep increase at higher molecular masses. Low melt viscosity and high tensile and impact strengths are desirable properties for a polymer to be commercially useful. Chemical resistivity The chemical attack on polymers involves softening, swelling and loss of strength of material. The resistance to chemical attack of a polymer depends on several factors such as the presence 9 of polar or non-polar groups the degree of crystallinity and molecular mass, degree of crosslinking and also on the nature of the attacking reagents. A polymer is more soluble in a solvent of similar chemical structure. Polymers having polar groups such as –OH or –COOH groups are usually attacked or dissolved by polar liquids such as water or alcohols. (E.g. resol resins swell in alcohols). Polymers with non-polar groups such as –CH3 and –C6H5 are not easily attacked by polar solvents but they easily swell and sometimes dissolve in non-polar solvents such as petrol, benzene and carbon tetrachloride. Polymers with large number of aromatic groups dissolve in aromatic solvents such as benzene. Polymers containing residual unsaturation undergo oxidative degradation when exposed to light (O2, O3 or UV attacks the double bond). For a given polymer, the swelling character decreases with the increase in the molecular mass. In polymers higher the degree of crystallinity higher is the chemical resistance. Greater the degree of cross linking (lesser is the solubility) higher is its chemical resistance. PLASTICS & RESINS Plastics Definition: Plastics are high polymers which can be moulded by the application of heat and pressure. There is no chemical change during the moulding process Examples: Polyethylene, polystyrene, PVC, polyester, Teflon, nylon, plexiglass, Bakelite, melamine, duroplast(thermosetting resin plastic) etc. Resins Resins are basic binding material which form a main part of the plastic and which actually has undergone polymerization reactions during the preparation or moulding of a plastic. Resin is a solid or liquid or semisolid organic low molecular weight, soluble and fusible polymer used as basis for plastics, adhesives, varnishes. Examples: Amino resins (UF resin, MF resin), phenolic resins, epoxy resins, thermosetting polyester resins etc Note: Thermoplastics/thermoplastic resins – These are the polymers which become soft on heating and hard on cooling and the process can be repeated for a number of times. They undergo reversible changes on heating. These are mostly linear polymers and branched structure polymers with flexible chains. Thermosetting plastics/thermosetting plastic resins – These are the polymers which are fusible on initial heating, but on further heating become permanently set insoluble, hard, rigid and three dimensional network product. They undergo irreversible changes on heating. These are strong covalent crosslinked polymers. Thermosetting polymers are generally harder and stronger than thermoplastics and have better dimensional stability. Note: There are four ways chain reaction polymerization are carried out in commercial 10 scale they are 1) Bulk polymerization 2) Suspension polymerization 3) Emulsion polymerization & 4) Solution polymerization Synthesis, properties and applications of PMMA and UF resin Polymethyl methacrylate (PMMA) {Plexi glass or Acrylic glass or transparent glass or Lucite} PMMA is a white transparent thermoplastic polymer. Preparation: Methyl methacrylate is the monomer used in the synthesis of PMMA. PMMA is obtained by subjecting methyl methacrylate to emulsion polymerization at 60-700C in presence of hydrogen peroxide. Properties: It is amorphous in nature due to the presence of bulky group. It has an excellent optical clarity, which is not affected by sunlight, but has poor scratch resistance. It has resistance to water, alkalis, inorganic salts but dissolves in organic solvents. It has good outdoor weathering property and dimensional stability. Applications: Used for making light fixtures, aircraft windows, attractive sign boards etc. used as paints and adhesives used to make durable lenses for automobile lighting, instrument and dust covers for equipments In making artificial eyes and teeth. In making transparent bottles, moulds, tubes plastic jewellery, toys etc. In making TV, computer screens, window screens. Urea-Formaldehyde Resins (UF Resin) Synthesis: It is prepared by condensation of urea with formaldehyde in presence of a base in stainless steel reactor at 50 ⁰C. The initial products monomethylol urea are compounded by addition of filler, plasticizer, pigment or catalyst followed by curing by applying heat and pressure to provide long -C-N-C-N chain polymer. This is called urea- formaldehyde resin and is a cross-linked polymer. 11 Properties: It gives clear, water-white products of good tensile strength, good electrical insulation. Good chemical resistance, greater hardness, good abrasion resistance. Amorphous and transparent plastic. Outstanding shape formation. Resistant to water, alkalis and inorganic salts but dissolves in organic solvents. Applications: It is employed for bonding in grinding wheels, as cation-exchange resins. Binder for glass fibre, rock, wool, plywood, to provide electrical insulation etc. ELASTOMERS (RUBBERS) Definition: They are high molecular weight polymers that possess elastic properties. i.e., they can undergo deformation under stress (to an extent 5-10 times original length) but regain their original shape when the stress is released. This property is called elasticity and it is due to coiled nature of chains in them. Natural rubber is found in several species of rubber trees. The virgin natural rubber is as useless as 24 carat gold. As such it has virtually no practical utility value. Because of many deficiencies of natural rubber, pure rubber has practically no applications. However, the utility value of natural rubber could be immensely improved if vulcanized. Vulcanization: It is a process of heating the natural or raw rubber with sulfur and other agents at 120-150 C for 1-4 hours. This process introduces sulfur cross links (bridge) between polymer chains of raw rubber (which involve residual double bonds of polymer 12 chains) and hence raw rubber gains higher strength, stability, elasticity and chemical resistance. Compounding: It is one more technique of improving the quality of natural rubber, it is the process of mixing of natural rubber with different ingredients to impart certain specific properties to natural rubber. The ingredients generally used are: Plasticizers (to improve flexibility and adhesion, ex: oils, waxes), accelerators (to improve physical properties, ex: amine aldehydes), fillers (to give strength and rigidity, ex: carbon black, zinc oxide), antioxidants (to prevent early deterioration of rubber by oxidation, ex: amines, phenols) and colouring agents (to impart desired colour to rubber products, ex: chromium oxide, ferric oxide). Curing: It is a process refers to the toughening or hardening of a polymer material by cross- linking of polymer chains, brought about by electron beams, heat or chemical additives. When the additives are activated by UV radiation, the process is called UV cure. In rubber, the curing process is also called vulcanization. Advantages of Synthetic Rubber - (a) resistance to heat, light (b) water repellent and solvent resistance (c) high chemical resistance - alkali/acid (d) high temperaturestability (e) high abrasion resistant. Butyl Rubber Synthesis: Butyl Rubber is synthesized by mixing isobutylene with up to 5% of isoprene monomer using methyl chloride as solvent using anhydrous aluminium chloride as catalyst at -80 oC. The product was vulcanized and dried by mixing with antioxidant. Properties: It is less sensitive to oxidative aging. It has very low gas permeability and shows good solvent resistance. It has excellent resistance to heat, abrasion, ageing, chemicals, polar solvents like alcohol and acetone. It has good electrical insulation properties. 13 Applications: (a) inner tube for tyres, automobile parts, hoses, tank-linings (b) insulating materials for high voltage transmission lines and cables. Nitrile Rubber (Buna-N or Europrene) Synthesis: Nitrile Rubber is synthesized by Emulsion Polymerization of butadiene and acrylonitrile monomers in a steel vessel at 30-40 oC using water as solvent in presence of H2O2 as free radical initiator. The resulting polymer latex was coagulated followed by vulcanization to provide Nitrile rubber. Properties: Nitrile rubber co-polymer has high tensile strength, excellent resistance to heat, sunlight, oils, fats, organic solvents, dilute acids but is less resistant to alkalis due to presence of base labile -CN group. Lower acrylonitrile composition in the rubber leadto decrease in glass transition temperature and show greater resistance to solvents upon increasing nitrile composition. This rubber can withstand a temperature of -40 oC to 100oC. Applications: (a) Nuclear, medical and aeronautical industry applications since it can withstand low and high temperatures (b) Used as sealant, expanded foam, floor mats, non-latex gloves, automotive transmission belts, gaskets, oil seals (c) as pigment binder, fuel and oil handling hoses. POLYMER COMPOSITES An ideal structural material (air craft industry) should have properties such as: (a) Low density (b) High strength and stiffness (c) Corrosion resistance and (d) Abrasion and impact resistance. No single metal, alloy, ceramic or polymeric materials are known that can offer combination of aforesaid properties. This is because a strong material is relatively dense and an increase in stiffness generally results in a decrease in impact strength. The search for materials possessing above properties led to the development of composite materials. They are initially developed for military and aerospace applications in 1940’s and are currently used for house decoration, bridge repair, mooring cables, structural strengthening and other applications. “A combination of two or more distinct components to form a new class of material suitable for structural applications is referred as composite materials”. In composites if one of the materials is polymer it is called polymer composite. Polymer composites are generally made of two components, namely (i) matrix and (ii) fibre. The fibre is generally embedded in the matrix in order to make the matrixstronger. The matrix is usually a thermoset material such as epoxy resin and it holds the fibre together. Fibre is most often glass; other synthetic fibres include carbon fibre, Kevlar etc. 14 Naturally occurring fibres such as wood - lignin, cellulose can also be employed. Importance as structure material Matrix binds the fibres together and can transmit and distribute any externally applied stress to the fibres. Matrix protects individual fibre from surface damage due to mechanical abrasion or any chemical reaction with the surroundings. The strength of the composite material is primarily due to the bonding forces between fibers and matrix. Kevlar (Aromatic polyamide) [-NH-C6H4-NH-CO-C6H4-CO-]n Kevlar is an aromatic polyamide or aramid. It was the first organic fibre with sufficient tensile strength and structural rigidity to be used in advanced composites. Kevlar is synthesized in solution of N-methyl-pyrrolidone and calcium chloride from the monomers 1,4-phenylene-diamine (para-phenylenediamine) and terephthaloyl chloride through a condensation reaction with the liberation of HCl. Properties: High tensile strength (five times stronger per unit weight than steel) High modulus of elasticity Very low elongation up to breaking point Low weight 15 High chemical inertness Very low coefficient of thermal expansion High fracture toughness (impact resistance) High cut resistance Flame resistance Uses: Used as reinforcement in tyre and rubber mechanical goods. Industrial applications are as cables, in asbestos replacements, brake linings, and body armor. Used in plastic reinforcements for boat hulls, airplanes, and bicycles. Kevlar is well known component of personal armor such as combat helmets, ballistic face masks, ec. Used as inner lining for some tyres to prevent punctures. The Kevlar fibre is used in woven rope and in cables. Aramid fibres are widely used for reinforcing composite materials, often in combination with carbon fibre and glass fibre. The matrix for high performance composite is usually epoxy resin. Typical applications include bodies for F1 racing cars, helicopter rotor blades, tennis, table tennis, badminton and squash rackets, cricket bats, and hockey sticks. Carbon fiber: Carbon fibers are a new breed of high strength materials. Carbon fiber containing at least 90% carbon obtained by the controlled pyrolysis of appropriate fibers. Generally, it’s formed as a composites with a light weight matrix. It has high strength, stiffness, light weight and outstanding properties. Various metal organic frame precursor materials are used to synthesis carbon fibre of different morphologies and various characteristics. The most prefer precursor is Polyacrylonitrile (PAN) cellulosic fibers, and phenolic fibers. Synthesis of Carbon fibre from PAN: Three stages are in the conversion of PAN in to high performance carbon fibre. Oxidative Stabilization: The polyacrylonitrile is first stretched and simultaneously oxidized in a temperature range of 200-300oC. This treatment converts thermoplastic PAN to a non-plastic cyclic compound. Carbonization: After the oxidative, the fibres are carbonized at about 1000C with nitrogen atmosphere for 2 hours. While this process non-carbon elements are removed and yield of about 60% of the mass of original PAN. Graphitization: After the carbonization the fibres are treated at 1500-3000oC, which improves the ordering and orientation of the crystallites in the direction of the fibre axis. 16 Preparation Applications: It is used in making parts of aeroplanes, space shuttles and modern motor bikes. It is used in tennis rackets, guitar strings and golf clubs. It is used in tripods, fishing rods and archery equipment. CONDUCTING POLYMERS “An organic polymer with highly delocalized pi-electrons system, which can exhibit electrical conductivity similar to a conductor upon doping”. Doping creates charge carriers like holes or excess electrons (similar to doped silicon) and thereby, an organic polymer becomes a conductor. Conducting polymers are also called as synthetic metals. The electrical conductivity of polyacetylene can be increased to 13 folds by doping with 17 electron acceptors and donors. Tremendous applications would exist as they are flexible, ease offabrication, stability, ease of processability, etc. Due to these reasons conducting polymers are considered to be better than conventional conducting inorganic materials. Examples are below are some of the conducting polymers Note: Synthesis of conducting polymers An organic polymer can be converted into a conducting polymer if it has Linear structure Extensive conjugation in polymeric back bone (pi-back bone) The conducting polymers are synthesized by doping, in which charged species are introduced in organic polymers having pi-backbone. The important doping reactions are 1. Oxidative doping (p-doping) 2. Reductive doping (n-doping) 3. Protonic acid doping (p-doping) The electrical conductivity of doped polyacetylene (105 sm-1) is remarkable to Teflon (10-8 sm-1) but is marginally lower than copper (108 sm-1). Oxidative doping (p-doping) – In this process, pi-backbone of a polymer is partially oxidized using a suitable oxidizing agent like iodine vapour, iodine in CCl 4, HBF4, perchloric acid, bezoquinone, aluminium chloride etc. Oxidizing agent takes away electron from pi-backbone creating positively a positively charged sites (holes) on polymer backbone. Thus polymer becomes p-type conductor by the movement of holes which act as current carriers for conduction. Reductive doping (n-doping) – In this process, pi backbone of a polymer is partially reduced by suitable reducing agent such as sodium or lithium naphthalide in trtra hydro furan. Reducing agent supplies electrons to pi backbone of the chain. This creates negatively charged sites on polymer backbone. These negatively charged sites are responsible for the conduction (polymer becomes n-type semiconductor as it conducts by free mobile electrons). 18 Protonic acid doping – The synthesis of conducting polyaniline (PANI) is a typical example of this type of doping technique. In this process, current carrying charged species (-ve/+ve) are created by the protonation of imine nitrogen. Synthesis of polyacetylene and its conduction mechanism Synthesis: Polyacetylene are synthesized using Zeigler-Natta catalyst. The catalyst tetrabutoxytitanium and triethylaluminum suspended in silicone oil through which monomer acetylene is passed and is stirred for two hours at 120 o C and then cooled slowly to room temperature resulting in thin sheets of polyacetylene. Mechanism of conduction in polyacetylene on oxidative doping The polymer chain is partially oxidized by using a mild oxidizing agent such as I 2 in CC4. In the first step, one electron is removed from pi-backbone of the chain leaving a positive charge on one of the carbon atoms. One more electron left out on another carbon makes it a delocalized free radical ion. The free radical cation thus formed is called polaron. Similarly, on second oxidation of chain containing polaron, another free radical-cation is formed which is called as bipolaron (radical recombination yields two charge carriers on each chain). The radicals migrate towards each other and combine to form one double bond. The chain now have two positive charges (holes). Positive charges are compensated by I 3 ions formed by the oxidizing agent during doping and thus the polymer is electrically neutral. As delocalized positive charges on the polymer chain are mobile (not the dopant anions, thus these delocalized positive charges are current carriers for conduction, these charges must move from chain to chain as well as along the chain for bulk conduction)) on applying potential they move and thus making a conductive polymer. On doping polyacetylene using iodine in CCl4, for partial oxidation, the conductivity increases from 10-5 S.cm-1 to 103 - 105 S.cm-1. 19 Applications Doped polyacetylene offers particularly high electrical conductivity therefore it can be used in electric wiring or electrode material in lightweight rechargeable batteries (ex. For higher power to weight ratio like, in coin type batteries. As conductive tracks in PCBs As sensors – humidity sensor, gas sensor, radiation sensor and biosensor (to measure glucose, galactose concentrations) etc. As film membrane for gas separations In LEDs 20 BIODEGRADABLE POLYMERS Polymers that get decomposed under aerobic or anaerobic conditions due to the action of microorganisms or enzymes are called biodegradable polymers. Note: Biodegradation generally takes place through the action of enzymes or chemicals associated with living organisms. Biodegradable polymers contain polymer chains that are hydrolytically or enzymatically cleaved, resulting in soluble degradation products. In biodegradation of polymers first step involves fragmentation of polymers in to low molecular weight species either by oxiadation, photolysis, and hydrolysis or by enzymatic action of microorganisms. For degradation of polymers, C-C bonds need to be broken. As these bonds are inert to enzymes, certain functional groups are added such as ester group (-COOR) to make them biodegradable. Biodegradability is particularly desired in biomedical applications, in which degradation of the polymer ensures clearance from the body and eliminates the need for retrieval or explant. Biodegradable polymers have applications in controlled/sustained release drug delivery approaches like Tissue engineering scaffolds and Temporary prosthetic implants. Polgycolic acid (PGA) or Polyglycolide Synthesis: Polyglycolide can be obtained through ring-opening polymerization of glycolide, the cyclic diester of glycolic acid. Ring-opening polymerization of glycolide can be catalyzed using aluminium isopropoxide as catalyst. In the presence of a catalyst glycolide heated to a temperature of 195 °C. Biodegradation of PGA: 1) Polyglycolide is characterized by hydrolytic instability owing to the presence of 21 the ester linkage in its backbone. The degradation process is erosive and appears to take place in two steps during which the polymer is converted back to its monomer glycolide first water diffuses into the amorphous (non-crystalline) regions of the polymer matrix, cleaving the ester bonds; the second step starts after the amorphous regions have been eroded, leaving the crystalline portion of the polymer susceptible to hydrolytic attack. Upon collapse of the crystalline regions the polymer chain dissolves. 2) When exposed to physiological conditions, polyglycolide is degraded by random hydrolysis, and apparently it is also broken down by certain enzymes, especially those with esterase activity. The degradation product, glycolic acid, is nontoxic, and it can enter the tricarboxylic acid cycle, after which it is excreted as water and carbon dioxide. A part of the glycolic acid is also excreted by urine. Applications: Biodegradable polymers are used commercially in both the tissue engineering and drug delivery field of biomedicine. Specific applications include: Sutures Dental devices (PLGA) Orthopaedic fixation devices Tissue engineering scaffolds Biodegradable vascular stents Biodegradable soft tissue anchor 22 23 24 25