Pipe Stress Engineering PDF
Document Details
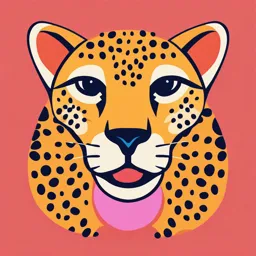
Uploaded by SpotlessCosine
Liang-Chuan (L.C.) Peng and Tsen-Loong (Alvin) Peng
Tags
Summary
This book covers pipe stress engineering, including analysis, component details, failure modes, and industry practices. It details strength of materials, thermal expansion, and flexibility. The book targets professionals in mechanical engineering and related fields.
Full Transcript
Pipe Stress Engineering by Liang-Chuan (L.C.) Peng and Tsen-Loong (Alvin) Peng Peng Engineering, Houston, Texas, USA CONTENTS Acknowledgments..................................................................................................................
Pipe Stress Engineering by Liang-Chuan (L.C.) Peng and Tsen-Loong (Alvin) Peng Peng Engineering, Houston, Texas, USA CONTENTS Acknowledgments........................................................................................................................................ xi Preface............................................................................................................................................. xiii Nomenclature................................................................................................................................... xv Chapter 1 Introduction....................................................................................................................................... 1 1.1 Scope of Pipe Stress Analysis................................................................................................... 2 1.2 Piping Components and Connecting Equipment..................................................................... 4 1.3 Modes of Failure...................................................................................................................... 9 1.3.1 Static Stress Rupture..................................................................................................... 9 1.3.2 Fatigue Failure............................................................................................................ 12 1.3.3 Creep Rupture............................................................................................................ 14 1.3.4 Stability Failure........................................................................................................... 17 1.3.5 Miscellaneous Modes of Failure................................................................................. 18 1.4 Piping Codes.......................................................................................................................... 19 1.5 Industry Practice.................................................................................................................... 22 1.5.1 Load Cases.................................................................................................................. 23 1.5.2 Local Support Stresses................................................................................................ 24 1.5.3 Local Thermal Stresses............................................................................................... 24 1.5.4 Pressure Effect on Flexibility...................................................................................... 25 1.5.5 Stress Intensification for Sustained Loads.................................................................. 25 1.5.6 Support Friction......................................................................................................... 25 1.5.7 Guide and Stop Gaps.................................................................................................. 26 1.5.8 Anchor and Restraint Stiffness................................................................................... 26 1.5.9 Small Piping................................................................................................................ 26 1.6 Design Specification.............................................................................................................. 26 1.6.1 Owner’s Design Specification..................................................................................... 26 1.6.2 Project Specification................................................................................................... 28 1.7 Plant Walk-down................................................................................................................... 30 Chapter 2 Strength of Materials Basics............................................................................................................. 33 2.1 Tensile Strength..................................................................................................................... 33 2.1.1 Modulus of Elasticity.................................................................................................. 34 2.1.2 Proportional Limit...................................................................................................... 34 2.1.3 Yield Strength, Sy....................................................................................................... 35 2.1.4 Ultimate Strength, Su.................................................................................................. 35 2.1.5 Stresses at Skewed Plane............................................................................................ 35 2.1.6 Maximum Shear Stress, Ss,max..................................................................................... 36 2.1.7 Principal Stresses........................................................................................................ 36 2.2 Elastic Relationship of Stress and Strain............................................................................... 36 2.2.1 Poisson’s Ratio........................................................................................................... 37 2.2.2 Shear Strain and Modulus of Rigidity......................................................................... 38 iv Contents 2.3 Static Equilibrium.................................................................................................................. 38 2.3.1 Free-Body Diagram.................................................................................................... 39 2.3.2 Static Equilibrium....................................................................................................... 40 2.4 Stresses due to Moments........................................................................................................ 40 2.4.1 Stresses due to Bending Moments.............................................................................. 40 2.4.2 Moment of Inertia....................................................................................................... 42 2.4.3 Polar Moment of Inertia............................................................................................. 42 2.4.4 Moment of Inertia for Circular Cross-Sections.......................................................... 43 2.4.5 Stresses due to Torsion Moment................................................................................ 43 2.5 Stresses in Pipes..................................................................................................................... 45 2.5.1 Stresses due to Internal Pressure................................................................................ 45 2.5.2 Stresses due to Forces and Moments.......................................................................... 47 2.6 Evaluation of Multi-Dimensional Stresses............................................................................. 49 2.6.1 General Two-Dimensional Stress Field...................................................................... 49 2.6.2 Mohr’s Circle for Combined Stresses......................................................................... 51 2.6.3 Theories of Failure...................................................................................................... 52 2.6.4 Stress Intensity (Tresca Stress)................................................................................... 52 2.6.5 Effective Stress (von Mises Stress).............................................................................. 53 2.7 Basic Beam Formulas............................................................................................................ 53 2.7.1 Guided Cantilever....................................................................................................... 55 2.8 Analysis of Piping Assembly.................................................................................................. 55 2.8.1 Finite Element............................................................................................................ 56 2.8.2 Data Points and Node Points..................................................................................... 57 2.8.3 Piping Assembly......................................................................................................... 58 Chapter 3 Thermal Expansion and Piping Flexibility....................................................................................... 61 3.1 Thermal Expansion Force and Stress.................................................................................... 61 3.1.1 Ideal Anchor Evaluation............................................................................................. 61 3.1.2 The Real Anchor......................................................................................................... 62 3.2 Methods of Providing Flexibility........................................................................................... 63 3.2.1 Estimating Leg Length Required................................................................................ 63 3.2.2 Inherent Flexibility..................................................................................................... 65 3.2.3 Caution Regarding Quick Check Formulas................................................................ 65 3.2.4 Wall Thickness and Thermal Expansion Stress.......................................................... 66 3.3 Self-Limiting Stress................................................................................................................ 66 3.3.1 Elastic Equivalent Stress............................................................................................. 67 3.4 Stress Intensification and Flexibility Factors......................................................................... 67 3.4.1 Ovalization of Curved Pipes....................................................................................... 68 3.4.2 Code SIFs................................................................................................................... 69 3.5 Allowable Thermal Expansion Stress Range......................................................................... 71 3.6 Cold Spring............................................................................................................................ 76 3.6.1 Cold Spring Gap......................................................................................................... 77 3.6.2 Location of Cold Spring Gap...................................................................................... 78 3.6.3 Cold Spring Procedure................................................................................................ 78 3.6.4 Multi-Branched System.............................................................................................. 79 3.6.5 Analysis of Cold Sprung Piping System...................................................................... 79 3.7 Pressure Effects on Piping Flexibility.................................................................................... 80 3.7.1 Pressure Elongation.................................................................................................... 80 3.7.2 Potential Twisting at Bends........................................................................................ 81 Contents 3.7.3 Pressure Elongation Is Self-Limiting Load................................................................. 82 3.7.4 Pressure Effect on Bend Flexibility and SIFs.............................................................. 82 3.8 General Procedure of Piping Flexibility Analysis.................................................................. 83 3.8.1 Operating Modes........................................................................................................ 83 3.8.2 Anchor Movements.................................................................................................... 84 3.8.3 Assignments of Operating Values............................................................................... 84 3.8.4 Handling of Piping Components................................................................................. 85 3.8.5 The Analysis............................................................................................................... 86 3.9 Problems With Excessive Flexibility...................................................................................... 86 3.9.1 Problems Associated With Excessive Flexibility........................................................ 88 3.10 Field Proven Systems............................................................................................................. 88 Chapter 4 Code Stress Requirements................................................................................................................ 91 4.1 “Design” Chapter of the Piping Codes.................................................................................. 91 4.2 Loadings to be Considered.................................................................................................... 92 4.2.1 Pressure...................................................................................................................... 93 4.2.2 Temperature............................................................................................................... 93 4.2.3 Weight Effects............................................................................................................. 95 4.2.4 Wind Load.................................................................................................................. 95 4.2.5 Earthquake.................................................................................................................. 96 4.2.6 Dynamic Fluid Loads................................................................................................. 96 4.2.7 Harmonic Anchor Displacement Loads..................................................................... 98 4.2.8 Passive Loads.............................................................................................................. 98 4.3 Basic Allowable Stresses........................................................................................................ 98 4.3.1 Bases for Establishing Allowable Stresses................................................................... 98 4.3.2 Code Allowable Stress Tables..................................................................................... 99 4.3.3 Weld Strength Reduction Factor.............................................................................. 100 4.4 Pressure Design................................................................................................................... 101 4.4.1 Straight Pipe............................................................................................................. 102 4.4.2 Curved Segment of Pipe............................................................................................ 104 4.4.3 Miter Bends.............................................................................................................. 106 4.4.4 Branch Connections................................................................................................. 109 4.4.5 Pressure Design for Other Components................................................................... 113 4.5 Stresses of Piping Components............................................................................................ 113 4.5.1 Calculations of Component Stresses......................................................................... 113 4.5.2 Sustained Stresses..................................................................................................... 117 4.5.3 Occasional Stresses................................................................................................... 119 4.5.4 Thermal Expansion and Displacement Stress Range................................................ 121 4.5.5 Code Stress Compliance Report............................................................................... 124 4.6 Class 1 Nuclear Piping......................................................................................................... 125 Chapter 5 Discontinuity Stresses..................................................................................................................... 133 5.1 Differential Equation of the Beam Deflection Curve........................................................... 133 5.2 Infinite Beam on Elastic Foundation With Concentrated Load........................................... 135 5.3 Semi-Infinite Beam on Elastic Foundation.......................................................................... 138 5.4 Application of Beam on Elastic Foundation to Cylindrical Shells....................................... 139 5.5 Effective Widths................................................................................................................... 141 5.6 Choking Model.................................................................................................................... 142 vi Contents 5.7 Stresses at Junctions Between Dissimilar Materials............................................................ 143 5.7.1 Uniform Pipes With Similar Modulus of Elasticity................................................... 144 5.7.2 A Pipe Connected to a Rigid Section........................................................................ 146 5.8 Vessel Shell Rotation........................................................................................................... 147 Chapter 6 Pipe Supports and Restraints......................................................................................................... 151 6.1 Device Terminology and Basic Functions........................................................................... 151 6.2 Support Spacing................................................................................................................... 157 6.3 Analysis of Piping Systems Resting on Supports................................................................. 159 6.4 Variable Spring and Constant Effort Supports..................................................................... 162 6.4.1 Variable Spring Hanger Selection Procedure............................................................ 163 6.4.2 Constant-Effort Supports.......................................................................................... 165 6.4.3 Spring Support Types and Installations.................................................................... 166 6.4.4 Setting of Loads — Hot Balance and Cold Balance................................................. 168 6.5 Support of Long Risers........................................................................................................ 170 6.5.1 Support Schemes...................................................................................................... 170 6.5.2 Support Loads.......................................................................................................... 171 6.5.3 Analysis Method....................................................................................................... 172 6.6 Significance of Support Friction.......................................................................................... 172 6.6.1 Effects of Support Friction....................................................................................... 172 6.6.2 Method of Including Friction in the Analysis........................................................... 174 6.6.3 Application of Friction Force................................................................................... 176 6.6.4 Methods of Reducing Friction Force........................................................................ 176 6.7 Support of Large Pipes......................................................................................................... 178 6.7.1 Saddle Supports Using Roark’s Formula.................................................................. 179 6.7.2 Ring Girder Supports................................................................................................ 180 6.7.3 Saddle Supports by Zick’s Method........................................................................... 185 6.7.4 Support Types........................................................................................................... 190 6.8 Pipe Stresses at Integral Support Attachments.................................................................... 194 6.8.1 Power Boiler Formulas for Lug Stresses................................................................... 194 6.8.2 Kellogg’s Choking Model......................................................................................... 198 6.8.3 WRC-107 Stress Evaluation...................................................................................... 202 6.9 Treatment of Support Stiffness and Displacement............................................................... 205 Chapter 7 Flexible Connections....................................................................................................................... 209 7.1 Basic Flexible Joint Elements and Analytical Tools............................................................ 211 7.1.1 Generic Flexible Connections................................................................................... 211 7.1.2 Bellow Elements....................................................................................................... 213 7.2 Using Catalog Data.............................................................................................................. 218 7.2.1 Background of Catalog Data.................................................................................... 218 7.2.2 Using the Catalog..................................................................................................... 219 7.2.3 Calculating Operational Movements........................................................................ 223 7.2.4 Cold Spring of Expansion Joint................................................................................ 224 7.3 Applications of Bellow Expansion Joints............................................................................. 225 7.3.1 Application of Axial Deformation............................................................................ 225 7.3.2 Lateral Movement and Angular Rotation................................................................. 230 7.3.3 Hinges and Gimbals.................................................................................................. 232 7.4 Slip Joints............................................................................................................................ 235 Contents vii 7.5 Flexible Hoses...................................................................................................................... 237 7.5.1 Types of Metallic Hoses............................................................................................ 237 7.5.2 Application and Analysis of Flexible Hoses............................................................. 238 7.5.3 Analysis of Hose Assembly....................................................................................... 241 7.6 Examples of Improper Installation of Expansion Joints...................................................... 241 7.6.1 Direction of Anchor Force....................................................................................... 241 7.6.2 Tie-Rods and Limit Rods......................................................................................... 242 7.6.3 Improperly Installed Anchors................................................................................... 244 Chapter 8 Interface with Stationary Equipment............................................................................................. 247 8.1 Flange Leakage Concern...................................................................................................... 247 8.1.1 Standard Flange Design Procedure.......................................................................... 248 8.1.2 Unofficial Position of B31.3...................................................................................... 250 8.1.3 Equivalent Pressure Method..................................................................................... 251 8.1.4 Class 2 Nuclear Piping Rules.................................................................................... 254 8.2 Sensitive Valves.................................................................................................................... 255 8.3 Pressure Vessel Connections............................................................................................... 256 8.3.1 Loadings Imposed to Piping from Vessel................................................................. 257 8.3.2 Vessel Shell Flexibility.............................................................................................. 258 8.3.3 Allowable Piping Load at Vessel Connections......................................................... 264 8.3.4 Heat Exchanger Connections................................................................................... 265 8.4 Power Boiler and Process Heater Connections................................................................... 265 8.5 Air-Cooled Heat Exchanger Connections............................................................................ 268 8.6 Low-Type Tank Connections.............................................................................................. 270 8.6.1 Displacement and Rotation of Tank Connection..................................................... 270 8.6.2 Stiffness Coefficients of Tank Nozzle Connection.................................................... 272 8.6.3 Allowable Piping Loads at Tank Connections.......................................................... 274 8.6.4 Practical Considerations of Tank Piping.................................................................. 280 Chapter 9 Interface with Rotating Equipment................................................................................................ 285 9.1 Brief Background of Allowable Piping Load on Rotating Equipment................................. 286 9.1.1 When Nobody Knew What to Do............................................................................. 286 9.1.2 First Official Set of Allowable Piping Loads............................................................. 287 9.1.3 Factors Behind the Low Allowable Piping Load...................................................... 287 9.2 Evaluation of Piping Load on Rotating Equipment............................................................. 288 9.2.1 Effect of Piping Loads............................................................................................... 288 9.2.2 Movements of Nozzle Connection Point.................................................................. 289 9.2.3 Analysis Approach.................................................................................................... 290 9.2.4 Selecting the Spring Hangers to Minimize the Weight Load.................................... 292 9.2.5 Multi-Unit Installation.............................................................................................. 293 9.2.6 Fit-up the Connection............................................................................................... 294 9.3 Steam Power Turbine.......................................................................................................... 295 9.4 Mechanical Drive Steam Turbines....................................................................................... 296 9.4.1 Allowable Loads at Individual Connection.............................................................. 296 9.4.2 Allowable for Combined Resultant Loads................................................................ 297 9.4.3 Basic Piping Layout Strategy.................................................................................... 298 9.5 Centrifugal Pumps............................................................................................................... 300 9.5.1 Characteristics Related to Piping Interface.............................................................. 300 viii Contents 9.5.2 Basic Piping Support Schemes.................................................................................. 302 9.5.3 Non-API Pumps....................................................................................................... 303 9.5.4 API Standard 610 Pumps......................................................................................... 304 9.6 Centrifugal Compressors...................................................................................................... 308 9.7 Reciprocating Compressors and Pumps.............................................................................. 313 9.7.1 Pulsating Flow.......................................................................................................... 313 9.7.2 Pulsation Pressure..................................................................................................... 315 9.7.3 Pulsation Dampener for Reciprocating Pumps......................................................... 316 9.7.4 Some Notes on Piping Connected to Reciprocating Machine.................................. 319 9.8 Problems Associated With Some Techniques Used in Reducing Piping Loads.................. 321 9.8.1 Excessive Flexibility.................................................................................................. 321 9.8.2 Improper Expansion Joint Installations.................................................................... 322 9.8.3 Theoretical Restraints............................................................................................... 322 9.9 Example Procedure for Designing Rotation Equipment Piping........................................... 324 Chapter 10 Transportation Pipeline and Buried Piping.................................................................................... 329 10.1 Governing Codes and General Design Requirements.......................................................... 330 10.1.1 B31.4 Liquid Petroleum Pipeline............................................................................ 331 10.1.2 B31.8 Gas Transmission Pipeline........................................................................... 333 10.2 Behavior of Long Pipeline.................................................................................................... 335 10.2.1 Pressure Elongation................................................................................................ 335 10.2.2 Anchor Force.......................................................................................................... 335 10.2.3 Potential Movement of Free Ends.......................................................................... 336 10.2.4 Movement of Restrained Ends................................................................................ 337 10.2.5 Stresses at Fully Restrained Section........................................................................ 337 10.3 Pipeline Bends..................................................................................................................... 339 10.4 Basic Elements of Soil Mechanics....................................................................................... 340 10.4.1 Types of Soils.......................................................................................................... 340 10.4.2 Friction Angle......................................................................................................... 340 10.4.3 Shearing Stress........................................................................................................ 341 10.4.4 Soil Resistance Against Axial Pipe Movement....................................................... 341 10.4.5 Lateral Soil Force................................................................................................... 343 10.4.6 Soil-Pipe Interaction............................................................................................... 344 10.5 Example Calculations of Basic Pipeline Behaviors.............................................................. 346 10.5.1 Basic Calculations................................................................................................... 346 10.5.2 Soil-Pipe Interaction............................................................................................... 347 10.6 Simulation of Soil Resistance............................................................................................... 348 10.7 Behavior of Large Bends...................................................................................................... 349 10.8 Construction of Analytical Model........................................................................................ 351 10.9 Anchor and Drag Anchor.................................................................................................... 353 Chapter 11 Special Thermal Problems.............................................................................................................. 357 11.1 Thermal Bowing................................................................................................................... 357 11.1.1 Displacement and Stress Produced by Thermal Bowing......................................... 357 11.1.2 Internal Thermal Stresses Generated by Bowing Temperature............................... 359 11.1.3 Occurrences of Thermal Bowing............................................................................. 360 11.1.4 The Problem Created by a Tiny Line...................................................................... 364 11.2 Refractory Lined Pipe.......................................................................................................... 365 Contents ix 11.2.1 Equivalent Modulus of Elasticity............................................................................ 365 11.2.2 Hot-Cold Pipe Junction.......................................................................................... 366 11.3 Un-Insulated Flange Connections....................................................................................... 369 11.4 Unmatched Small Branch Connections............................................................................... 369 11.5 Socket-Welded Connections................................................................................................ 370 Chapter 12 Dynamic Analysis — Part 1: SDOF Systems and Basics................................................................. 373 12.1 Impact and Dynamic Load Factor....................................................................................... 373 12.2 SDOF Structures.................................................................................................................. 375 12.2.1 Working Formula for SDOF Systems..................................................................... 376 12.2.2 Un-Damped SDOF Systems................................................................................... 376 12.2.3 Damped SDOF Systems......................................................................................... 382 12.2.4 Summary of the Characteristics of SDOF Vibration............................................... 386 12.3 Damping.............................................................................................................................. 387 12.4 Sonic Velocity Versus Flow Velocity................................................................................... 389 12.4.1 Sonic Velocity......................................................................................................... 390 12.4.2 Flow Velocity.......................................................................................................... 392 12.5 Shaking Forces due to Fluid Flow....................................................................................... 395 12.6 Safety Valve Relieving Forces.............................................................................................. 397 12.6.1 Open Discharge System.......................................................................................... 397 12.6.2 Closed Discharge System........................................................................................ 401 12.7 Steam Turbine Trip Load.................................................................................................... 403 Chapter 13 Dynamic Analysis — Part 2: MDOF Systems and Applications..................................................... 409 13.1 Lumped-Mass Multi-Degree of Freedom Systems............................................................... 409 13.1.1 Mass Lumping........................................................................................................ 410 13.1.2 Free Vibration and Modal Superposition............................................................... 412 13.2 Piping Subject to Ground Motion........................................................................................ 413 13.2.1 Response Spectra Method...................................................................................... 415 13.2.2 Combination of Response Spectra Analysis Results............................................... 417 13.2.3 Comparison of Modal Combination Methods........................................................ 419 13.2.4 Puzzles of Absolute Closely Spaced Modal Combination...................................... 421 13.2.5 Compensation for the Higher Modes Truncated.................................................... 422 13.2.6 Design Response Spectra........................................................................................ 423 13.3 Account for Uncertainties.................................................................................................... 426 13.4 Steady-State Vibration and Harmonic Analysis................................................................... 428 13.4.1 Basic Vibration Patterns......................................................................................... 428 13.4.2 Allowable Vibration Displacement and Velocity.................................................... 429 13.4.3 Formulation of Harmonic Analysis......................................................................... 437 13.4.4 Evaluation of Vibration Stress................................................................................ 444 13.5 Time-History Analysis......................................................................................................... 446 13.5.1 Treatment of Damping............................................................................................ 446 13.5.2 Integration Schemes................................................................................................ 448 13.5.3 Time Step, Stability, and Accuracy......................................................................... 450 13.5.4 Example Time-History Analysis............................................................................. 451 Contents Appendix A................................................................................................................................... 459 Appendix B................................................................................................................................... 461 Appendix C................................................................................................................................... 462 Appendix D................................................................................................................................... 464 Appendix E................................................................................................................................... 466 Appendix F.................................................................................................................................... 474 INDEX............................................................................................................................................ 475 ACKNOWLEDGMENTS This book is essentially the summary of the knowledge accumulated by the authors through 40 years of practice as piping mechanical engineers. I, the senior author, would like to use this opportunity to express my appreciation and gratitude to many friends, colleagues, and supervisors for providing those learning opportunities and environments. First, I would like to thank Ron Hollmeier of Pioneer Service & Engineering in Chicago for offering me my first pipe stress job developing a computer pro- gram for pipe stress analysis in 1967. This job allowed me to stay in the United States and led to a long, interesting career. I am grateful to Bechtel’s Bill Doble and Joe Gilchrist, who did not hesitate to send me to English classes and put me on interesting jobs such as the Trans-Alaskan pipeline and Black. Mesa coal slurry pipeline projects. My most memorable work was done at Nuclear Services Cor- poration in San Jose. Working as part of a team that included Bob Keever, Randy Broman, Doug Munson and myself, and with help from Professor Gram Powell of University of California-Berkeley and valuable inputs from Mel Pedell and Dane Shave of Stone and Webster, we created the NUPIPE pipe stress software, which became a very powerful tool in the design of nuclear piping. I am greatly indebted to Don Mckeehan and Ed Bissaillon of M. W. Kellogg for their encouragement and imple- mentation of the SIMFLEX software. As a result of the authors’ long association with M. W. Kellogg, this book is noticeably influenced by Kellogg’s philosophy and approaches mentioned in the second edition of the Kellogg’s book — Design of Piping Systems (1956, John Wiley and Sons). Suggestions from Ray Chao and David Osage of Exxon Research were very helpful during the development of the PENGS program. The estimated 1000 engineers, who came to my training classes conducted in a dozen countries, have greatly widened my perspective on piping mechanical work. The authors are very grateful to ASME Press for valuable comments and excellent editing. We would also like to thank the twin sisters Lina and Linda for reading the manuscript. The fact that these two non-technical sis- ters have read through the entire draft of the manuscript has greatly encouraged us. Liang-Chuan (L.C.) Peng Tsen-Loong (Alvin) Peng xi PREFACE Pipe stress analysis calculates the stress in a piping system subject to normal operating loads such as pressure, weight, and thermal expansion, and occasional loads such as wind, earthquake, and water hammer. Because all piping systems are connected to equipment such as vessels, tanks, pumps, tur- bines, and compressors, the piping stress analysis also involves evaluation of the effect of the piping forces and moments to the connecting equipment. As the piping stress is controlled by the arrange- ment of the supports and restraints, the scope of piping stress includes also pipe supports. The whole scope of this work is generally referred to as piping mechanical. Before the advent of the electronic computer, pipe stress analysis was handled by very specialized engineers. Only large corporations and specialized firms had the personnel to do the job. It normally took a specialist to use the calculator non-stop for a couple of weeks just to analyze the flexibility of a moderately complex piping system to absorb the thermal expansion of the pipe. Because only very few of these engineers knew how to analyze piping stress, most engineers treated it as some type of a mysterious subject. Engineers saw there were expansion loops, offsets, and special supports such as spring hangers and constant effort supports, but did not really know why they were there. The limited scope of pipe stress analysis dealing with the piping flexibility for absorbing thermal expansion was called piping flexibility analysis. With the arrival of the electronic computer in the 1970s, and especially the personal computer in the 1980s, suddenly everybody knew how to analyze pipe stress. This has generated even more mystery about the field. Nowadays, we occasionally see an electrical engineer, although discouraged, conduct- ing the analysis just as proficiently as a mechanical or a structural engineer. This partly stems from the fact that colleges and universities normally do not offer any course on pipe stress. This leaves the knowledge and skill of pipe stress and piping engineering to be learned by self-study and actual prac- tice. Practitioners who obtain the best computer program and comprehend the manual most will do the better job. With the rapid advancement in computer technology, a piping flexibility analysis nowadays takes only few minutes via an appropriate computer software. Therefore, the task of the stress engineer has been shifted from the traditional stress calculation to stress engineering. The emphasis is not on how to calculate the stress, but rather on how to utilize the analysis tool to design a better plant. However, just because it is easy to get the stress calculated, engineers often depend too much on the computer and forget about the fundamentals and engineering common sense. Without the fundamentals and common sense, one may not even be aware of the unreasonable results produced by the computer, to say the least about good engineering. This book emphasizes engineering common sense as well as the basic principles. The following are some examples of piping problems that might have been solved by just good engineering common sense: A plant operated smoothly for the first 10 years, and then experienced a leakage at the main process piping about every 4 months after a major revamping. Experts were con- sulted, sophisticated analyses were performed, and expensive modifications were made to no avail. Had the engineers used the basic thermal stress common sense, the problem would have been solved with very little effort (see Section 11.2.2). The pressure thrust force is very critical at a bellow expansion joint. Anchors are often needed at bellow expansion joint installations to resist the pressure thrust force, but xiii xiv Preface sometimes an anchor placed at the wrong location may just be the cause of the problem. The wrong anchor had been contributing to severe vibration at some rotating equipment. Had we known that the pressure thrust force at an expansion joint is generally much higher than the force that can be tolerated by the rotating equipment, the problem would not have occurred (Chapters 7 and 9). A small steam purge line in a large process pipe had caused the plant piping to twist wildly, breaking many connecting leads. Several major re-routing of the piping systems were made, but the problem persisted. Had the involved engineers had some idea about thermal bowing, the problem could have been easily corrected (see Section 11.1.4). Providing the knowledge for solving the problems such as the ones listed above is the primary goal of the book. Chapter 1 summarizes the scopes and requirements related to piping mechanical activities, and the subsequent chapters discuss how to deal with them. Chapter 1 contains some of the authors’ inside views, which we hope will help the readers progress more confidently and comfortably into the piping mechanical field. Nowadays, making a calculation with a computer is so fast that we often hear about the “what-if” approach in engineering. What all this “what-if” approach accomplishes is making numerous random trials and the wish that one of these trials will hit the mark sooner or later. The problem is that after a few trials, most people lose the ability to make sense of the trials. The more they try, the more they get confused. In contrast, this book puts emphasis on the “what, why, and how” to guide the readers into this 3-W approach — that is, to be aware of the problem, understand the cause of the problem, and to solve the problem or prevent it from happening. The authors will try to explain all the necessary tasks of pipe stress engineering with basic funda- mentals. Although only a few very fundamental equations are introduced, theoretical backgrounds will be covered in as much detail as possible. The book is titled Pipe Stress Engineering to distinguish it from a regular pipe stress analysis book, which normally lacks the coverage of the engineering aspects. Although this book is intended for piping mechanical engineers, it is also a suitable reference book for piping designers, plant engineers, and civil-mechanical engineers. The book can be used as the textbook for a one- or two-semester elective course given at the senior or graduate level. NOMENCLATURE (All are in consistent units. Special usages and non-consistent units are noted in the main text. Ab- breviations are listed at the end) A Cross-section area A Thickness allowance including corrosion, thread, etc. A Flow area Ab Total net cross-section area of flange bolts Ac Corrosion allowance of pipe thickness Am Manufacturing under-tolerance of pipe thickness Ap Pressure area encircled by the bolt circle of flange AT Nozzle throat area or valve orifice area a Bellow effective pressure thrust area a Sonic speed a Tank nozzle radius B Flange inside diameter B Tank bottom plate thermal expansion factor b Flange effective gasket width b Thickness of support saddle, in pipe axial direction b Width of the beam cross-section b Width of a small element or strip C Cold spring factor C Flange bolt circle diameter C Thermal bowing local stress factor, defined in Figure 11.2 [C] Damping matrix of the structural system C1, C2 integration constants to be determined by boundary conditions C2, K2 ASME Class-1 nuclear piping stress indices for displacement loading CED End coefficient with respect to vibration displacement, defined in Eq. (13.43) CEV End coefficient with respect to vibration velocity, defined in Eq. (13.52) CK Kármán force coefficient on vortex shedding force Cw Ratio of total pipe weight, including pipe metal weight, content, and insulation, to pipe metal weight CX Vibration stress amplification factor due to concentrated weight c Soil cohesion stress c Viscous damping cp Specific heat of gas under constant pressure condition cv specific heat of gas under constant volume condition D Diameter of pipe or circular cross-section D Diameter of vessel shell or run pipe {D} Displacement vector in global coordinates, for both element and overall structures {D¢} Element displacement vector in local coordinates Dc Combined equivalent diameter of all the nozzles at rotating equipment De Equivalent diameter of an individual nozzle at rotating equipment xv xvi Nomenclature DLF Dynamic load factor d Displacement d Diameter d Diameter of branch pipe de Elastic displacement limit of an elastic-plastic restraint dp Pitch diameter of bellow E Modulus of elasticity E Longitudinal joint efficiency, normally expressed with allowable stress as (SE) Ee Equivalent modulus of elasticity for composite pipe e Strain ecom Combined total equivalent axial bellow deformation per convolution ex Axial bellow deformation per convolution ey Equivalent axial bellow deformation per convolution due to lateral displacement y eθ Equivalent axial bellow deformation per convolution due to rotation θ F Force F Gas pipeline design factor (defined in Table 10.2) F Pipeline anchor force, or potential expansion force {F} Force vector in global coordinates, for both element and overall structures {F¢} Element force vector in local coordinates |F| Absolute value of force F F1 Force equivalent to total impulse function = ρAV 2 + PA {FC} Vector of cosine components of the harmonic force FK Kármán force or vortex shedding force Fmax Net maximum peak shaking force of a given piping leg Fn Normal force FnS Pressure force at point n due to standing pressure wave FnT Pressure force at point n due to traveling pressure wave FP Tank nozzle pressure force defined in Eq. (8.30) FR Radial force FR Resultant force Fs Shear force {FS} Vector of sine components of the harmonic force F(t) Force as function of time FRX Rotation spring constant about x axis for a generic flexible joint FRY Rotation spring constant about y axis for a generic flexible joint FRZ Rotation spring constant about z axis for a generic flexible joint FTX Translation spring constant in x axis for a generic flexible joint FTY Translation spring constant in y- axis for a generic flexible joint FTZ Translation spring constant in z- axis for a generic flexible joint f Line force per unit length of active line in an attachment f Natural frequency of the structural system, or frequency of a vibration f Pipeline friction force per unit length of pipe f Stress range factor for calculating allowable expansion stress range f Support friction force f1(βx) Beam on elastic foundation function defined by Eq. (5.17a) f2(βx) Beam on elastic foundation function defined by Eq. (5.17b) f3(βx) Beam on elastic foundation function defined by Eq. (5.17c) f4(βx) Beam on elastic foundation function defined by Eq. (5.17d) fw Working (nominal) axial spring constant per bellow convolution G Flange gasket load reaction circle diameter G Shear modulus of elasticity, modulus of rigidity Nomenclature xvii g1 Flange hub thickness at back of flange gx, gy, gz Cold spring gap in x, y, z directions, respectively H Depth of soil cover, from top of pipe to soil surface H Height of the beam cross-section H Ring force per unit circumferential breadth h Flexibility characteristic of piping component, defined in Table 3.1 h Safety valve height, defined in Fig. 12.17 h Soil depth at an arbitrary point I Moment of inertia of a pipe or beam cross-section [I ]D Identity matrix, or unit diagonal matrix, with 1 on diagonal and 0 at elsewhere Ip Polar moment of inertia i Stress intensification factor i Imaginary number, square root of -1 iC Stress intensification factor for circumferential stress iL Stress intensification factor for longitudinal stress K Bulk modulus of the liquid = -dp/(dv/v) K Stiffness or spring constant of support structure [K ] Stiffness matrix in global coordinates, for both element and overall structures [K¢] Element stiffness matrix in local coordinates KA Coefficient of active lateral soil pressure Kh Spring constant per unit pipe length for horizontal soil resistance KL EJMA bellow lateral spring rate = KV KN Napier constant for steam flow KR EJMA bellow rotational spring rate = KRθ KRy Bellow rotational spring rate due to lateral end deflection KRθ Bellow rotational spring rate due to end rotation, when free lateral deflection is. allowed KV Bellow lateral spring rate due to lateral end deflection. End moment also created Kv Spring constant per unit pipe length for vertical soil resistance Kx Bellow axial spring rate k Flexibility factor of piping component k Ratio of specific heats = cp/cv k Spring constant and directional stiffness k Spring constant of foundation per unit length of beam L Distance from the center of tank nozzle to tank bottom L Length L Pipeline active length L Support spacing [L] Transformation matrix between local coordinates and global coordinates LMP Larson-Miller parameter defined in Eq. (1.2) ℓ Elongation ℓ Support lug length ℓ Length of element M Mass M Moment [M ] Mass matrix of the structural system Mmass Concentrated mass MC Circumferential moment ML Longitudinal moment MR Resultant moment Mt Torsion moment xviii Nomenclature Mw Molecular weight My Moment produced by bellow lateral displacement without free end rotation Mθ Moment produced by bellow rotation with free lateral displacement M(θ + D) Moment produced by bellow rotation without free lateral displacement m Flange gasket factor m Mass per unit length of pipe ṁ Mass flow rate N Circumferential force per unit length of pipe N Number of bellow convolutions N Number of operating cycles NS Strouhal number on vortex shedding frequency; NS = fD/V n Number of stiffening rings at each support saddle P Pressure Pd Flange design pressure Pe Equivalent pressure due to force and moment acting on a flange PS Pressure amplitude of a standing wave PT Pressure amplitude of a traveling wave p Pressure p* Critical pressure at sonic velocity state Q Pipeline end resistance force Q Total support load Q Volumetric flow rate [Q] Influence or relation matrix relating ground motion to every part of the structure q Distributed external force per unit length of beam q Pitch of bellow convolution qa Deformed pitch at centerline of bellow qc Compressed pitch at pitch diameter of bellow qe Extended pitch at pitch diameter of bellow qh Horizontal soil resistance force per unit length of pipe qv Vertical soil resistance force per unit length of pipe R Bend radius R Gas constant = R/Mw R Radius of curvature R Radius of circular cross-section R Radius of vessel shell R Reaction force R Universal gas constant Ra Acceleration response spectra Raj Acceleration response spectra of jth independent support motion RC Radius of the crown on a vessel head Rd Displacement response spectra Rv Velocity response spectra r Radius of an arbitrary circular ring r Radius of pipe cross-section r Radius of round attachment r Rotation rm Mean radius of pipe cross-section S Basic allowable stress for pipeline S Stress SA Basic allowable thermal expansion stress range Sb Flange bolt stress Nomenclature xix Sc Allowable stress of pipe material at cold ambient condition SE Expansion stress SEB Benchmark expansion stress range Sel Endurance strength of pipe material SelA Allowable endurance strength SF Flange gasket stress due to axial force {Sg} Vector of independent support motion components Sh Allowable stress of pipe material at hot operating condition Sh Pressure hoop stress Shp Hoop pressure stress Si Stress intensity (=twice of the maximum shear stress) Slp Longitudinal pressure stress SM Flange gasket stress due to bending moment SMYS Specified Minimum Yield Strength SP Flange gasket stress due to pressure SPW Longitudinal pipe stress due to pressure and weight ST Local thermal stress due to thermal bowing Su Ultimate strength Sy Yield strength Syc Yield strength at cold condition Syhx The lesser of yield strength at hot condition and 160% of the stress producing 0.01% creep in 1000 hours at the operating temperature T Absolute temperature, K (=273 + °C) or R (=460 + °F) T Period of vibration T Temperature T Vessel thickness or run pipe thickness T* Critical absolute temperature at sonic velocity state t Thickness of bellow t Thickness of branch pipe t Thickness of pipe, generic t Thickness of tank shell at nozzle location t Time td Time duration of an impulse loading to Effective valve opening time V Bellow lateral force V Velocity V Volume V Tangential shear force per unit circumferential breadth v Shear force v Specific volume W Total flange bolt load W Total shear force at pipe cross-section W Weight load in force unit W Weight of the free body, or weight load W Weld strength reduction factor Wp Weight of pipe per unit length of pipe Ws Weight of soil cover per unit length of pipe w Weight per unit length {X } Displacement vector of the structural system XA Distance between the top of nozzle and tank bottom plate XB Distance between the bottom of nozzle and tank bottom plate xx Nomenclature XC Distance between the center of nozzle and tank bottom plate {X}g Ground motion displacement vector x Axial displacement of beam or bellow x, y, z Coordinates in x, y, z directions, respectively YC Allowable coefficient, Fig. 8.25, for circumferential moment on tank nozzle YF Allowable coefficient, Fig. 8.25, for axial force on tank nozzle YL Allowable coefficient, Fig. 8.25, for longitudinal moment on tank nozzle y Adjustment coefficient (see Table 4.1) for pipe thickness calculation y Flange gasket seating stress y Local lateral displacement of beam or bellow y Pipeline end axial movement y Radial displacement of pipe shell Z Section modulus of a pipe or beam cross-section Zp Polar section modulus ZPA Zero period acceleration of the response spectra curve z Compressibility of real gas = pv/(RT ) Greek symbols a Constant defining participation of mass in damping, see Eq. (13.76) a Vibration allowable stress reduction factor, 1.3 for carbon and low alloy steels and 1.0 for austenitic stainless and high alloy steels a Thermal expansion rate, as expansion per unit length per unit temperature β Angle from top of pipe to edge of saddle β Angle of branch intersection β Branch/run diameter ratio β Characteristic parameter of beam on elastic foundation, defined by Eq. (5.26) β Constant defining participation of stiffness in damping, see Eq. (13.76) β Frequency ratio of the applied frequency to the natural frequency of the system γ Rotational deformation D Clearance between tube and tube sheet hole D Deflection D.. Difference of.. DA Amplitude decay due to step-by-step time-history analysis DT Period elongation due to step-by-step time-history analysis Dt Integration time step for time-history analysis ζ Damping ratio, the ratio of damping to critical damping η Nozzle flow efficiency θ Angle of circular wedge θ Angle of miter θ Angle of the inclining plane θ Rotation θ Support saddle angle l Tank geometrical parameter defined in Eq. (8.30) µ Friction coefficient ν Poisson ratio ξ Coordinate or coefficient of normal mode space, i.e., {X} = [F]{x} {ξC} Cosine component of modal coordinate vector of harmonic response {ξS} Sine component of modal coordinate vector of harmonic response π 3.141592 ρ Angle of the maximum bending moment location at a support ring ρ Density, mass per unit volume Nomenclature xxi å Summation of t Shear stress υ Flow velocity {Φ} Eigenvector, or natural vibration shape [Φ] Eigenvector matrix with eigenvectors as columns φ Angle from top of pipe φ Bellow rotation per convolution φ Soil internal friction angle ω Circular frequency, or rotational speed ωd Circular natural frequency for damped system ωn Circular natural frequency for un-damped (and also damped) system Subscripts 0 At origin 0 initial state 0, 1, 2, … At location 0, 1, 2,…, etc., or at condition 0, 1, 2,…, etc. a Allowable allow Allowable a, b, … at point a, b, … , etc. b bending b branch pipe c circumferential direction c cold or ambient temperature c critical condition D discharge side e equivalent f friction H hoop direction h hub of flange h hot or operating temperature hp hoop pressure i in-plane i inside surface of the pipe L longitudinal direction l longitudinal direction lp longitudinal pressure m mean value max maximum value n natural vibration n nominal n normal o out-plane o outside surface of the pipe p pressure R radial direction R resultant R rigid body response R run pipe r ring stiffener r run pipe xxii Nomenclature s suction side s shear st static T test condition t tangential t torsion xy on x plane and in y direction x, y, z components in x, y, and z directions, respectively y yield condition y at y distance away Abbreviations ABS absolute ANSI American National Standards Institute API American Petroleum Institute ASME American Society of Mechanical Engineers ASTM American Society of Testing and Materials AWWA American Water Work Works Association B&PV Boiler and Pressure Vessel CEN Comité Européen de Normalisation (European Standard) cps cycles per second DLF dynamic load factor ft foot or feet in. inch or inches Hz Hertz = cycles per second K Kelvin = 273 + °C ksi kilo pounds per square inch = 1000 psi lb pound (weight or force) lbf pound force m meter MDOF multi degrees of freedom MSS Manufacturer Standardization Society of the Valve and Fitting Industry N Newton NRC Nuclear Regulatory Commission Pa Pascal = N/m2 psi pounds per squire inch R Rankin = 460 + °F RPM revolution per minute SDOF single degree of freedom SIF stress intensification factor SRSS square root sum of the squares WRC Welding Research Council chapter 1 INTRODUCTION A piping system is the most efficient and common means of transporting fluids from one point to another. Within a petrochemical complex, acres and acres of piping can be seen running in every di- rection and at many different levels. Piping constitutes 25% to 35% of the material of a process plant, requires 30% to 40% of the erection labor, and consumes 40% to 48% of the engineering man-hours. The actual importance of piping, however, can far exceed these percentages. An entire piping sys- tem is composed of a large number of components. The failure of just one single component has the potential to shut down the entire plant or, worse yet, cause serious public safety problems. In spite of this, piping is generally considered a low-technology subject in the academia. Very few colleges teach the subject, leaving engineers to gain this knowledge only through actual practice in the field. To find out exactly where pipe stress fits in the piping design process, let us first find out what pro- cedures are involved in designing a piping system. A piping system is designed in the following steps by different engineering disciplines: (1) Process engineers, basing on process requirements and plant capacity, determine, among other things, the flow path, the flow medium and quantity, and operating conditions. They then put all this information into process flow diagrams. (2) Material specification engineers assign suitable categories of specifications for the piping sys- tem based on the process flow and reactivity of the contained fluid. Each specification is appli- cable to certain combinations of fluid types, temperature ranges, and pressure ranges. Material specifications normally include pipe material, pipe wall thickness for each pipe size, the corro- sion and erosion allowances, flange class, valve types, fitting and branch connection type, bolt material, gasket type, etc. (3) System engineers combine process flow diagrams, material specifications, and equipment data sheets to create operational piping diagrams. They select the applicable material specification and determine the size for each line based on flow quantity, allowable pressure drop, and flow stability. Piping diagrams are generally combined with the necessary instrument and control circuits to become piping and instrument diagrams (P&IDs). Special items such as potential two-phase flow and slug-flow zones are also identified on these diagrams for special consider- ation in design and analysis. In addition to the P&IDs, a line list covering all pipe spools is also constructed. This line list contains most of the design, upset, and operating parameters to be used in the layout, analysis, and fabrication of the piping system. (4) Piping designers, in coordination with other disciplines, conceive an overall plant layout, per- form a piping routing study, determine the pipe rack locations, and place the actual piping that connects to designated points. They lay out and support the piping by following the rules and procedures set up by each individual company. In general, three sets of drawings are prepared. The first set is the schematic planning drawings, used as a communication board between different departments. Actions and comments from related disciplines are all resolved and recorded in these drawings. Pipe supports are also recorded in this set of drawings. The second set is the composite drawings, consisting of to-scale drawings of all pipes and equip- ment in the area. These drawings, to be used in the construction, are evolved from the planning Chapter 1 drawings. The third set of drawings is the isometrics of the piping, used for stress checks and shop fabrications. (5) Piping mechanical engineers check the stresses and supports of the systems. Using the P&IDs, they develop operating modes so that all the expected operating conditions are properly ana- lyzed. Proper supports and restraints are selected and placed to optimize the overall cost and performance of the systems. They also design or specify piping specialty items, such as expan- sion joints, flue heads, special connections, spring hangers, vibration supports, and so forth. It may be a bit surprising that designing a piping system is so involved. Indeed, on a large project, not only is every discipline required, but the effort also engages quite a few people. Piping design and pip- ing mechanical are the two disciplines that require the most number of personnel. However, for a small project handled by a small outfit, generally only one or two people are assigned to take care of all the work of various disciplines. In such cases, pipe stress activity is often neglected. Due to the resilient na- ture of ductile piping systems, the piping will work most of the time, even without going through proper stress checks. This may be acceptable for small non-hazardous piping, but not for most of public and industrial piping systems, which require the piping system to be safe and operational all the time. Item (5) is the main subject of this book. The task of a piping mechanical engineer is generally called pipe stress and support. The scope of the pipe stress and support activity has increased exponentially in the past three decades. This is due to the stringent requirements of the modern plant. For instance, in the 1960s, the pipe stress and support manpower used for a petrochemical plant was about 4000 man-hours. A nuclear power plant in that era would have used about the same amount of pipe stress man-hours. Nowadays, the pipe stress and support manpower required for a petrochemical plant has increased by ten times to about 50,000 man-hours. The effort required by a nuclear power plant has grown 1000-fold to reach as high as 2 million man-hours. With this exponential growth in the man- hours involved, the probability of getting sub-standard output from some of the engineers is very high. A time-saving tool, such as an efficient pipe stress computer program, not only significantly reduces the cost of designing a plant, but also greatly improves the quality of the plant. Good work starts with a good grasp of the scope of the jobs that need to be done. In this chapter, the non-mathematical con- cepts of pipe stress activities will be discussed. 1.1 SCOPE OF PIPE STRESS ANALYSIS Pipe stress analysis is, of course, the analysis of the stress in the pipe. However, if we ask what is to be analyzed, many will hesitate to answer. In the 1950s and 1960s, when engineers started analyzing piping systems, they had only one thing in mind: calculating the stress due to thermal expansion. In other words, they checked the piping layout to see if the piping system was flexible enough to absorb the thermal expansion due to temperature change. The analysis was referred to as piping flexibility analysis. Books [3–6] and articles [7–9] written during this period dealt mainly with flexibility analysis. Later on, as technology progressed, pipe stress analysis encompassed much more than just checking flexibility; yet nowadays many engineers still refer to pipe stress analysis as flexibility analysis. This slight mix-up in terms is not important. However, the concept that flexibility is the only consideration in piping stress analyses can lead to an expensive, and unsafe, sub-standard design. For instance, many engineers tend to consider that providing additional flexibility in the piping is a conservative ap- proach. In reality, additional flexibility not only increases the material cost and pressure drop, it also makes the piping prone to vibration, the biggest problem area of the piping in operation. Since the publication of the 1955 piping code and Kellogg’s book, failures due to insufficient flexibility have become very rare. Nowadays, most failures are caused by vibration, thermal bowing, creep, thermal fatigue not related to flexibility, steam/water hammer, expansion joints, and so forth [11, 12]. These facts should serve as clues toward designing better piping systems. Consider, for example, the piping installed to move the process fluid from the storage tank to the process unit as shown in Fig. 1.1. First, we have to deal with tank shell displacement and rotation Introduction Fig. 1.1 Tasks of pipe stress analysis due to the hydrostatic bulge of the shell. This temperature-independent displacement and rotation will exert a great influence on the connecting piping. Furthermore, the tank nozzle connection is far from rigid. Its flexibility has to be estimated and included in the analysis. Then, after the pipe forces and moments at the connection are calculated, they have to be evaluated for their acceptance. There Chapter 1 are many items like these, which are not normally called piping flexibility, but are required to be considered in piping stress analysis. These items, using Fig. 1.1, shall be identified one by one starting from this tank connection. The next items we come across are flanges and valves. Can they maintain the tightness under piping forces and moments? Can valves operate properly under pipe forces and moments? These need to be checked even though the pipe itself is strong enough for the same forces and moments. We know the support friction can also have a significant effect on piping movement. The situations and methods to include the friction effect also need to be considered. In addition to the average pipe temperature, the pipe might also have a temperature gradient across the pipe cross-section due to stratified flow or blow off of low-temperature fluid. Even radiant energy from the sun on empty un-insulated pipe can cause this type of temperature difference. This type of bowing phenomena can create a great problem in the piping and needs to be considered. For piping connecting to the rotating equipment such as pumps, the pipe load has to be maintained to within the manufacturer’s allowable range to prevent the equipment from excessive vibration, wear, and overheating. The piping connected to rotating equipment also needs the consideration of poten- tial water hammer, pulsation, and other dynamic phenomena. Proper spring hangers will need to be selected and placed to ensure that the piping is properly sup- ported under all operating conditions. At the vessel connection, again, the flexibility and displacement at the connection have to be in- cluded in the analysis. After pipe forces and moments at the connection are calculated, vessel local stresses have to be evaluated to see if they are acceptable. Then, of course, if the structure is located in an earthquake or hurricane zone, earthquake and wind loading have to be considered when designing the piping system. In general, the purpose of pipe stress analysis can be summarized into two broad categories: (a) Ensure structural integrity: This involves the calculation of stresses in the pipe due to all design loads. Necessary procedures are taken to keep the stress within the code allowable limits. This code stress check is the basic assurance that failures from breaks or cracks will not occur in the piping. (b) Maintain system operability: A piping itself can be very strong, yet the system may not be oper- able due to problems in the connecting equipment. Flange leakage, valve sticking, high stress in the vessel nozzle, and excessive piping load on rotating equipment are some of these prob- lems. The work required in maintaining the system operability is generally much greater than that required in ensuring the structural integrity. This is mainly attributable to the lack of coordination between engineers of different disciplines. Rotating equipment manufacturers, for instance, design non-pressure parts, such as support and base plate, based mainly on the weight and the torque of the shaft. Then they specify the allowable piping load with that de- sign, disregarding the fact that some practical piping load always exists and needs to be ac- commodated. The allowable loads they provide are generally much too small to be practical. Unfortunately, these allowable values go unchallenged, mainly because the industry as a whole gives no incentives to manufacturers to produce equipment that can resist the extra piping load. If more and more engineers would request the extra strength or give preferential treat- ment to manufacturers that produce stronger equipment, an optimal solution might eventually be reached. Until such time comes, piping mechanical engineers should be prepared to spend three times as many man-hours in stress engineering the piping system connected to rotating equipment. 1.2 PIPING COMPONENTS AND CONNECTING EQUIPMENT In this section, we will deal with three categories of hardware: pressure parts of the piping proper, support and restraint elements, and connecting equipment. The functions of support and restraint ele- Introduction ments are apparent. And because a separate chapter is dedicated to pipe supports and restraints, we shall not include them in this discussion. In the following, we use Fig. 1.2 as a guide to summarize the types of piping components and arrays of connecting equipment typically encountered in piping stress engineering. Fig. 1.2 Piping components and interface equipment Chapter 1 (a) Main pipe. Starting with the main pipe, we have to know the pipe material, which is gener- ally given by the American Society of Testing and Materials (ASTM) specification number. The pipe is generally identified by its nominal diameter and nominal thickness. For pipes 12 in. (300 mm) or smaller, the nominal diameter is very close to the inside diameter of the pipe with standard wall thickness. However, for sizes 14 in. (350 mm) and larger, the nominal pipe diameter is exactly the same as the outside diameter of the pipe. For each pipe size, the outside diameter is fixed for a given nominal diameter. This is done so that all pipes with the same nominal size can use the same pipe sup- port attachments such as clamps, and insulation blocks. Each pipe size also has several commercially available thicknesses called nominal thickness. The available nominal thicknesses are standardized in related standards such as ASTM A-106 and American Society of Mechanical Engineers (ASME) B36.10. In the United States, these thicknesses are also represented by weight grades as standard (Std), extra strong (XS), and double extra strong (XXS), and by schedule numbers ranging from schedule 10 (Sch-10) to schedule 160 (Sch-160). Stainless steel pipes have separate schedule numbers appended with the letter “S” such as Sch-5S, Sch-10S, etc. For instance, a 6-in., Std pipe means that the pipe has a 6.625-in. outside diameter and a 0.280-in. wall thickness, whereas a 14-in., Sch-40 pipe corresponds to a pipe with a 14-in. outside diameter and 0.438-in. wall thickness. The schedule number itself dose not represent any thickness; it has thickness assigned only when tagged with a pipe diameter. See Appendix A for standard nominal pipe wall thicknesses. The pipe material involves different manufacturing processes that might implicate some stress ris- ers and tolerances. The pipe can be broadly classified via the method in which it was manufactured: seamless or welded. Welded pipes, which are somewhat weaker than seamless pipes due to the weld, are further classified into several categories. From the seam position, we have longitudinally welded and spirally welded (two main types). From the welding process, we have furnace butt welded, electric resistance welded, and electric fusion welded (three methods). Each type of welding has its unique joint efficiency that needs to be included in the pressure design. For tolerances, we are mostly con- cerned with thickness under-tolerance, which can reduce the pressure resisting capability of the pipe. Under-tolerance is the allowable amount of thickness in which a manufactured pipe can be made thin- ner than the nominal thickness. In general, seamless pipes and electric resistance welded pipes have a higher under-tolerance of about 12.5% of the nominal wall thickness. Electric fusion welded pipes have a somewhat different under-tolerance determined by the plate used; however, API 5L suggest 10% to 12.5% for the fusion welded pipe also. This under-tolerance needs to be included in the stress design of the pipe. (b) Welds. To connect piping components together, we use either circumferential weld or flange connections. The circumferential weld, also called girth weld, is for a permanent connection, whereas the flange is used for locations requiring occasional separation. The girth weld also has a joint efficiency that works against longitudinal stress. This joint efficiency does not affect pressure design, which is controlled by circumferential hoop stress. For loadings other than pressure, this girth weld joint efficiency is implied in the stress intensification factor. Therefore, in most piping codes, circumferential weld joint efficiency is seldom mentioned. It is also often overlooked by stress engineers. (c) Weld strength reduction factor. In addition to the joint efficiency that affects the general strength of the piping, the weld also hastens creep failure at creep temperature. The additional reduc- tion of creep strength over the non-weld-affected body is called the weld strength reduction factor. This is the factor applied, over the joint efficiency, at high temperature ranges. The same factor is applied at both longitudinal welds and circumferential welds. However, longitudinal weld affects only the calculation of wall thickness, which is governed by the circumferential hoop stress. On the other hand, circumferential weld affects only the sustained longitudinal stress due to pressure, weight, and other mechanical loads. The weld strength reduction factor is not applicable to occasional stress due to the generally short duration of the stress. It also does not affect thermal expansion and displace- ment stress range due to the self-limiting nature of the stress. Generally, the temperature that requires the application of the weld strength reduction factor starts from 950°F (510°C). However, B31.1 and Introduction B31.3 treat it slightly differently. Details of the application are given in Chapter 4, which deals with code stress requirements. (d) Flanges. Flanges are available in several different types. From the structural construction point of view, it can be classified as welding neck, slip-on, or lap joint (three types). Each type has its length, weight, and stress intensification factor, all of which have to be identified and considered in the stress analysis. Flanges are also identified by classes. Each class has its set of pressure-temperature rat- ings, relating allowable pressures with operating temperatures. At a given operating temperature, the selected flange class shall offer an allowable pressure that is either equal to or greater than the design pressure. Table 1.1 shows the pressure-temperature rating for forged A-105 carbon steel flanges. The table also shows the flange thickness required for each class, for 6-in. and 12-in. flanges. The data, taken from ASME B16.5 , is presented mainly to show the general trend of the allowable pressure versus temperature and class. It also gives some idea about the magnitude of flange thickness, exclud- ing hub. For Class 2500 flanges, flange thickness equals roughly 2/3 of the pipe diameter. It is also interesting to note that the flange classes were originally called pounds. Class 300 flanges were called 300-pound flanges, and so forth. This was because, originally, Class 300 flanges were rated for 300 pounds per square inch (psi) pressure at a benchmark temperature. Pound (lb) meant psi, and had nothing to do with the weight of the flange. The benchmark temperatures used were different for different materials. For A-105 carbon steel, the benchmark temperature was 500°F for Class 150, and 850°F for all other classes. However, as more accurate material data became available and more accurate stress calculations became possible, the original ratings also appeared less accurate. Cur- rently, for A-105 flanges, Class 150 has a rating pressure of 170 psi at 500°F benchmark temperature, and Class 300 has a rating pressure of 270 psi at 850°F benchmark temperature. It is obvious that cur- rent pressure-temperature ratings no longer correspond to the original benchmark idea. Although the pound classification is not very meaningful right now, it is still used by many engineers. See Appendix E for layout dimension and weight of valves and flanges. Table 1.1 Pressure-temperature rating for A-105 carbon steel flanges Chapter 1 (e) Bends. The turning of the pipe is accomplished by bends. Bends have several general types. The most common bend is the so-called long-radius elbow, which has a bend radius equal to 1.5 times the nominal pipe diameter. This is a fitting manufactured by the code-approved standard ASME B16.9 and others. The same standard also includes a short-radius elbow, which has a bend radius equal to the nominal pipe diameter. Short-radius elbows are used in tight spots where available space is not enough for long-radius elbow. The cited factory-made forged elbows are quite expensive and also incur high flow friction loss due to the small bend radius. One alternative is to make the bend di- rectly by bending the pipe. To avoid excessive thinning and potential wrinkling, the bend radius of this type is generally bigger than three nominal pipe diameters. The one shown in the figure is a 5-D bend. Another alternative, mainly to save cost, is to cut the pipe into angled miters and bring them together to form the bend. This type of bend is called a miter bend. All these different bends have different wall thickness requirements, flexibility factors, and stress intensification factors to be considered in the design and analysis. ( f ) Branches. Branch connections are used to form the branches of the piping. The most com- mon full-size branch connection is the forged welding tee, which is made according to ASME B16.9 and other standards. Generally, the welding tee is quite expensive, but provides the smoothest flow passages and least stress intensification factor among all branch connections. Besides the weld- ing tee, the most economical and readily available branch connection is the un-reinforced fabricated tee. Generally called a stub-in connection, this type of connection is made simply by cutting a hole on the run pipe and welding the branch pipe to it. A stub-in connection is cheap and easy to make, but can handle only about one-half of the pressure that the pipe can. It also has a very high stress intensi