Pharmacology Handouts PDF
Document Details
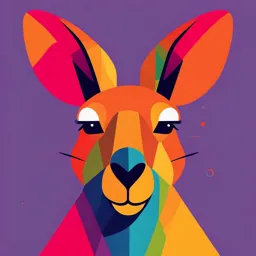
Uploaded by IntricateDidactic
Systems Plus College Foundation
2024
Tags
Summary
These handouts cover fundamental pharmacology concepts, including drug naming, creation, administration routes, pharmacokinetics, pharmacodynamics, and pharmacotherapeutics for Nursing Care Management 106 students at SYSTEMS PLUS COLLEGE FOUNDATION in the Philippines. It details various drug sources and administration methods.
Full Transcript
SYSTEMS PLUS COLLEGE FOUNDATION Angeles City COLLEGE OF NURSING Nursing Care Management 106: PHARMACOLOGY 1st Semester, S.Y. 2024-2025 TABLE OF CONTENTS Page No. TITLE PAGE...
SYSTEMS PLUS COLLEGE FOUNDATION Angeles City COLLEGE OF NURSING Nursing Care Management 106: PHARMACOLOGY 1st Semester, S.Y. 2024-2025 TABLE OF CONTENTS Page No. TITLE PAGE 1 TABLE OF CONTENTS 2 SCHOOL’S MISSION & VISION 3 COURSE CONTENT & COURSE 4 REQUIREMENT 9 HANDOUT No.1: FUNDAMENTAL CONCEPTS OF PHARMACOLOGY PART: A 14 HANDOUT No.2: FUNDAMENTAL CONCEPTS OF PHARMACOLOGY PART: B HANDOUT No.3: DRUGS AFFECTING THE BODY 24 SYSTEM AGENTS AFFECTING CENTRAL NERVOUS SYSTEM (CHOLINERGIC and ANTICHOLINERGIC DRUGS) HANDOUT No.4: DRUGS AFFECTING THE 30 RESPIRATORY SYSTEM HANDOUT No. 5 CARDIOVASCULAR SYSTEM 42 PART A HANDOUT No. 6 ANTIHYPERTENSIVE DRUGS 57 HANDOUT No. 7 CARDIOVASCULAR SYSTEM 63 PART B 78 HANDOUT No.8 DRUGS AFFECTING THE GASTROINTESTINAL SYSTEM HANDOUT No.9 ANTI-INFECTIVE DRUGS 87 HANDOUT No. 10 DRUGS AFFECTING THE RENAL 95 SYSTEM 102 REFERENCES 103 APPENDIX A: LIST OF PRESCRIPTION ABBREVIATION HANDOUT No.1: FUNDAMENTAL CONCEPTS OF PHARMACOLOGY I. LEARNING OUTCOMES At the end of this lecture, the students should be able to: 1. This module focuses on the fundamental principles of pharmacology. It discusses basic information, such as how drugs are named and how they’re created. It also discusses the different routes by which drugs can be administered. 2. This module also discusses what happens when a drug enters the body. This involves three main areas: pharmacokinetics (the absorption, distribution, metabolism, and excretion of a drug) pharmacodynamics (the biochemical and physical effects of drugs and the mechanisms of drug actions) pharmacotherapeutics (the use of drugs to prevent and treat diseases) 3. In addition, the module provides an introduction to drug interactions and adverse drug reactions. II. CONTENT Drugs have a specific kind of nomenclature—that is, a drug can go by three different names: The chemical name is a scientific name that precisely describes its atomic and molecular structure. The generic, or nonproprietary, name is an abbreviation of the chemical name. The trade name (also known as the brand name or proprietary name) is selected by the drug company selling the product. Trade names are protected by copyright. The symbol after the trade name indicates that the name is registered by and restricted to the drug manufacturer. To avoid confusion, it’s best to use a drug’s generic name because any one drug can have a number of trade names. In 1962, the federal government mandated the use of official names so that only one official name would represent each drug. The official names are listed in the United States Pharmacopeia and National Formulary. Family ties Drugs that share similar characteristics are grouped together as a pharmacologic class (or family). Beta-adrenergic blockers are an example of a pharmacologic class. The therapeutic class groups drugs by therapeutic use. Antihypertensives are an example of a therapeutic class. Where drugs come from Traditionally, drugs were derived from natural sources, such as: Plants Animals Minerals Today, however, laboratory researchers use traditional knowledge, along with chemical science, to develop synthetic drug sources. One advantage of chemically developed drugs is that they’re free from the impurities found in natural substances. In addition, researchers and drug developers can manipulate the molecular structure of substances such as antibiotics so that a slight change in the chemical structure makes the drug effective against different organisms. The first-, second-, third-, and fourth generation cephalosporins are an example. The earliest drug concoctions from plants used everything: the leaves, roots, bulb, stem, seeds, buds, and blossoms. Subsequently, harmful substances often found their way into the mixture. As the understanding of plants as drug sources became more sophisticated, researchers sought to isolate and intensify active components while avoiding harmful ones. The active components consist of several types and vary in character and effect: Alkaloids, the most active component in plants, react with acids to form a salt that can dissolve more readily in body fluids. The names of alkaloids and their salts usually end in “-ine.” Examples include atropine, caffeine, and nicotine. Glycosides are also active components found in plants. Names of glycosides usually end in “-in” such as digoxin. Gums constitute another group of active components. Gums give products the ability to attract and hold water. Examples include seaweed extractions and seeds with starch. Resins, of which the chief source is pine tree sap, commonly act as local irritants or as laxatives. Oils, thick and sometimes greasy liquids, are classified as volatile or fixed. Examples of volatile oils, which readily evaporate, include peppermint, spearmint, and juniper. Fixed oils, which aren’t easily evaporated, include castor oil and olive oil. Animal magnetism: The body fluids or glands of animals can also be drug sources. The drugs obtained from animal sources include: Hormones such as insulin Oils and fats (usually fixed) such as cod-liver oil Enzymes, which are produced by living cells and act as catalysts, such as pancreatin and pepsin Vaccines, which are suspensions of killed, modified, or attenuated microorganisms. Mineral springs Metallic and nonmetallic minerals provide various inorganic materials not available from plants or animals. The mineral sources are used as they occur in nature or are combined with other ingredients. Examples of drugs that contain minerals are iron, iodine, and Epsom salts. Down to DNA Today, most drugs are produced in laboratories. Today, most drugs are produced in laboratories and can be: natural (from animal, plant, or mineral sources) synthetic. Examples of drugs produced in the laboratory include thyroid hormone (natural) and ranitidine (synthetic). Recombinant deoxyribonucleic acid research has led to other chemical sources of organic compounds. For example, the reordering of genetic information has enabled scientists to develop bacteria that produce insulin for humans. How drugs are administered? A drug’s administration route influences the quantity given and the rate at which the drug is absorbed and distributed. These variables affect the drug’s action and the patient’s response. Routes of administration include: Buccal, sublingual, translingual: certain drugs are given buccally (in the pouch between the cheek and gum). sublingually (under the tongue), or translingually (on the tongue) to speed their absorption or to prevent their destruction or transformation in the stomach or small intestine gastric: this route allows direct instillation of medication into the GI system of patients who can’t ingest the drug orally Intradermal: substances are injected into the skin (dermis); this route is used mainly for diagnostic purposes when testing for allergies or tuberculosis intramuscular: this route allows drugs to be injected directly into various muscle groups at varying tissue depths; it’s used to give aqueous suspensions and solutions in oil, immunizations, and medications that aren’t available in oral form intravenous: the I.V. route allows injection of substances (drugs, fluids, blood or blood products, and diagnostic contrast agents) directly into the bloodstream through a vein; administration can range from a single dose to an ongoing infusion delivered with great precision Oral: this is usually the safest, most convenient, and least expensive route; drugs are administered to patients who are conscious and can swallow Rectal and vaginal: suppositories, ointments, creams, gels, and tablets may be instilled into the rectum or vagina to treat local irritation or infection; some drugs applied to the mucosa of the rectum or vagina can be absorbed systemically Respiratory: drugs that are available as gases can be administered into the respiratory system; drugs given by inhalation are rapidly absorbed, and medications given by such devices as the metered-dose inhaler can be self- administered, or drugs can be administered directly into the lungs through an endotracheal tube in emergency situations Subcutaneous (subQ): with the subQ route, small amounts of a drug are injected beneath the dermis and into the subcutaneous tissue, usually in the patient’s upper arm, thigh, or abdomen Topical: this route is used to deliver a drug through the skin or a mucous membrane; it’s used for most dermatologic, ophthalmic, otic, and nasal preparations. Drugs may also be given as specialized infusions injected directly into a specific site in the patient’s body, such as an epidural infusion (into the epidural space), intrathecal infusion (into the cerebrospinal fluid), intrapleural infusion (into the pleural cavity), intraperitoneal infusion (into the peritoneal cavity), intraosseous infusion (into the rich vascular network of a long bone), and intraarticular infusion (into a joint). New drug development In the past, drugs were found by trial and error. Now they’re developed primarily by systematic scientific research. The Food and Drug Administration (FDA) carefully monitors new drug development, which can take many years to complete. Only after reviewing extensive animal studies and data on the safety and effectiveness of the proposed drug will the FDA approve an application for an investigational new drug (IND). Phases of new drug development When the Food and Drug Administration (FDA) approves the application for an investigational new drug, the drug must undergo clinical evaluation involving human subjects. This clinical evaluation is divided into four phases: Phase I The drug is tested on healthy volunteers in phase I. Phase II Involves trials with people who have the disease for which the drug is thought to be effective. Phase III Large numbers of patients in medical research centers receive the drug in phase III. This larger sampling provides information about infrequent or rare adverse effects. The FDA will approve a new drug application if phase III studies are satisfactory. Phase IV Is voluntary and involves postmarked surveillance of the drug’s therapeutic effects at the completion of phase III. The pharmaceutical company receives reports from doctors and other health care professionals about the therapeutic results and adverse effects of the drug. Some medications, for example, have been found to be toxic and have been removed from the market after their initial release. III. KEY POINTS FOR REVIEW (This is the highlight or summary of your discussions to this handout) Drugs have a specific kind of nomenclature—that is, a drug can go by three different names: The chemical name is a scientific name that precisely describes its atomic and molecular structure. The trade name (also known as the brand name or proprietary name) is selected by the drug company selling the product. Traditionally, drugs were derived from natural sources, such as: plants, animals and minerals Alkaloids, the most active component in plants, react with acids to form a salt that can dissolve more readily in body fluids. Vaccines, which are suspensions of killed, modified, or attenuated microorganisms. Buccal, sublingual, translingual: certain drugs are given buccally (in the pouch between the cheek and gum) Sublingually (under the tongue), or translingually (on the tongue) intradermal: substances are injected into the skin (dermis) I.V. route allows injection of substances (drugs, fluids, blood or blood products, and diagnostic contrast agents) directly into the bloodstream through a vein. Oral: this is usually the safest, most convenient, and least expensive route. Subcutaneous (subQ): with the subQ route, small amounts of a drug are injected beneath the dermis and into the subcutaneous tissue, usually in the patient’s upper arm, thigh, or abdomen Topical: this route is used to deliver a drug through the skin or a mucous membrane. The Food and Drug Administration (FDA) approves the application for an investigational new drug, the drug must undergo clinical evaluation involving human subjects. This clinical evaluation is divided into four phases: Phase I, Phase II, Phase II, Phase I IV. TEST YOUR KNOWLEDGE 1. While teaching a patient about drug therapy for diabetes, you review the absorption, distribution, metabolism, and excretion of insulin and oral antidiabetic agents. Which principle of pharmacology are you describing? A. Pharmacokinetics B. Pharmacodynamics C. Pharmacotherapeutics D. Drug potency 2. Which type of drug therapy is used for a patient who has a chronic condition that can’t be cured? A. Empiric therapy B. Acute therapy C. Maintenance therapy D. Supplemental therapy 3. Which branch of pharmacology studies the way drugs work in living organisms? A. Pharmacotherapeutics B. Pharmacokinetics C. Drug interactions D. Pharmacodynamics 4. This route allows injection of substances directly into the blood stream through vein. A. Intravenous B. Intraosseous C. Intrathecal D. Subcutaneous 5. Isordil dinitrate was given sublingually to a client suffering from angina as the first dose. This means: A. The medication was put under the tongue B. The medication was put above the tongue C. Administered vaginally D. Administered on the eye HANDOUT NO.2: FUNDAMENTAL CONCEPTS IN PHARMACOLOGY I. LEARNING OUTCOMES At the end of this lecture, the students should be able to: 1. Learn and understand the key concepts of pharmacokinetics 2. Learn the key concepts of pharmacodynamics 3. Analyze the key concepts of pharmacotherapeutics 4. Familiarize the key types of drug interactions and adverse reactions. II. CONTENT Pharmacokinetics Kinetics - refers to movement. Pharmacokinetics deals with a drug’s actions as it moves through the body. Therefore, pharmacokinetics discusses how a drug is: Absorbed (taken into the body) Distributed (moved into various tissues) Metabolized (changed into a form that can be excreted) Excreted (removed from the body). Pharmacokinetics - This branch of pharmacology is also concerned with a drug’s onset of action, peak concentration level, and duration of action. Absorption Drug absorption covers a drug’s progress from the time it’s administered, through its passage to the tissues, until it reaches systemic circulation. On a cellular level, drugs are absorbed by several means—primarily through active or passive transport. Passive transport requires no cellular energy because diffusion allows the drug to move from an area of higher concentration to one of lower concentration. Passive transport occurs when small molecules diffuse across membranes and stops when drug concentration on both sides of the membrane is equal. Active transport requires cellular energy to move the drug from an area of lower concentration to one of higher concentration. Active transport is used to absorb electrolytes, such as sodium and potassium, as well as some drugs such as levodopa. Pinocytosis is a unique form of active transport that occurs when a cell engulfs a drug particle. Pinocytosis is commonly employed to transport fat-soluble vitamins (vitamins A, D, E, and K). If only a few cells separate the active drug from the systemic circulation, absorption will occur rapidly and the drug will quickly reach therapeutic levels in the body. Typically, absorption occurs within seconds or minutes when a drug is administered sublingually, I.V., or by inhalation. Absorption occurs at a slower rate when drugs are administered by the oral, I.M., or subQ routes because the complex membrane systems of GI mucosal layers, muscle, and skin delay drug passage. At the slowest absorption rates, drugs can take several hours or days to reach peak concentration levels. A slow rate usually occurs with rectally administered or sustained-release drugs. At the slowest absorption rates, drugs can take several hours or days to reach peak concentration levels. A slow rate usually occurs with rectally administered or sustained-release drugs. Other factors can affect how quickly a drug is absorbed. For example, most absorption of oral drugs occurs in the small intestine. If a patient has had large sections of the small intestine surgically removed, drug absorption decreases because of the reduced surface area and the reduced time that the drug is in the intestine. Drugs absorbed by the small intestine are transported to the liver before being circulated to the rest of the body. The liver may metabolize much of the drug before it enters the circulation. This mechanism is referred to as the first-pass effect. Liver metabolism may inactivate the drug; if so, the first-pass effect lowers the amount of active drug released into the systemic circulation. Therefore, higher drug dosages must be administered to achieve the desired effect. Increased blood flow to an absorption site improves drug absorption, whereas reduced blood flow decreases absorption. More rapid absorption leads to a quicker onset of drug action. o For example: The muscle area selected for I.M. administration can make a difference in the drug absorption rate. Blood flows faster through the deltoid muscle (in the upper arm) than through the gluteal muscle (in the buttocks). The gluteal muscle, however, can accommodate a larger volume of drug than the deltoid muscle. Pain and stress can decrease the amount of drug absorbed. This may be due to a change in blood flow, reduced movement through the GI tract, or gastric retention triggered by the autonomic nervous system response to pain. High-fat meals and solid foods slow the rate at which contents leave the stomach and enter the intestines, delaying intestinal absorption of a drug. Drug formulation (such as tablets, capsules, liquids, sustainedrelease formulas, inactive ingredients, and coatings) affects the drug absorption rate and the time needed to reach peak blood concentration levels. Combining one drug with another drug, or with food, can cause interactions that increase or decrease drug absorption, depending on the substances involved. Distribution Drug distribution is the process by which the drug is delivered from the systemic circulation to body tissues and fluids. Distribution of an absorbed drug within the body depends on several factors: Blood flow Solubility Protein binding. After a drug has reached the bloodstream, its distribution in the body depends on blood flow. The drug is quickly distributed to organs with a large supply of blood. These organs include the: Heart Liver Kidneys Distribution to other internal organs, skin, fat, and muscle is slower. Distribution The ability of a drug to cross a cell membrane depends on whether the drug is water or lipid (fat) soluble. Lipid-soluble drugs easily cross through cell membranes; water-soluble drugs can’t. Lipid-soluble drugs can also cross the blood-brain barrier and enter the brain. As a drug travels through the body, it comes in contact with proteins such as the plasma protein albumin. The drug can remain free or bind to the protein. The portion of a drug that’s bound to a protein is inactive and can’t exert a therapeutic effect. Only the free, or unbound, portion remains active. A drug is said to be highly protein-bound if more than 80% of the drug is bound to protein. Metabolism Drug metabolism, or biotransformation, is the process by which the body changes a drug from its dosage form to a more water-soluble form that can then be excreted. Drugs can be metabolized in several ways: Most drugs are metabolized into inactive metabolites (products of metabolism), which are then excreted. Other drugs are converted to active metabolites, which are capable of exerting their own pharmacologic action. Active metabolites may undergo further metabolism or may be excreted from the body unchanged. Some drugs can be administered as inactive drugs, called prodrugs, which don’t become active until they’re metabolized The majority of drugs are metabolized by enzymes in the liver; However, metabolism can also occur in the plasma, kidneys, and membranes of the intestines. In contrast, some drugs inhibit or compete for enzyme metabolism, which can cause the accumulation of drugs when they’re given together. This accumulation increases the potential for an adverse reaction or drug toxicity Certain diseases can reduce metabolism. These include liver diseases such as cirrhosis as well as heart failure, which reduce circulation to the liver. Genetics allows some people to metabolize drugs rapidly and others to metabolize them more slowly. Environment, too, can alter drug metabolism. For example, cigarette smoke may affect the rate of metabolism of some drugs; a stressful situation or event, such as prolonged illness, surgery, or injury, can also change how a person metabolizes drugs. Developmental changes can also affect drug metabolism. For instance, infants have immature livers that reduce the rate of metabolism, and elderly patients experience a decline in liver size, blood flow, and enzyme production that also slows metabolism. Excretion Drug excretion refers to the elimination of drugs from the body. Most drugs are excreted by the kidneys and leave the body through urine. Drugs can also be excreted through the lungs, exocrine (sweat, salivary, or mammary) glands, skin, and intestinal tract. The half-life of a drug is the time it takes for one-half of the drug to be eliminated by the body. Factors that affect a drug’s half-life include its rate of absorption, metabolism, and excretion. Knowing how long a drug remains in the body helps determine how frequently it should be administered. In addition to absorption, distribution, metabolism, and excretion, three other factors play important roles in a drug’s pharmacokinetics: Onset of action Peak concentration Duration of action. The onset of action refers to the time interval from when the drug is administered to when its therapeutic effect actually begins. Rate of onset varies depending on the route of administration and other pharmacokinetic properties. o The duration of action is the length of time the drug produces its therapeutic effect. Pharmacodynamics Pharmacodynamics is the study of the drug mechanisms that produce biochemical or physiologic changes in the body. The interaction at the cellular level between a drug and cellular components, such as the complex proteins that make up the cell membrane, enzymes, or target receptors, represents drug action. The response resulting from this drug action is the drug effect. A drug can modify cell function or rate of function, but it can’t impart a new function to a cell or to target tissue. Therefore, the drug effect depends on what the cell is capable of accomplishing. A drug can alter the target cell’s function by: modifying the cell’s physical or chemical environment interacting with a receptor (a specialized location on a cell membrane or inside a cell). Many drugs work by stimulating or blocking drug receptors. A drug attracted to a receptor displays an affinity for that receptor. When a drug displays an affinity for a receptor and stimulates it, the drug acts as an agonist. An agonist binds to the receptor and produces a response. This ability to initiate a response after binding with the receptor is referred to as intrinsic activity. If a drug has an affinity for a receptor but displays little or no intrinsic activity, it’s called an antagonist. An antagonist prevents a response from occurring. Antagonists can be competitive or noncompetitive. A competitive antagonist competes with the agonist for receptor sites. Because this type of antagonist binds reversibly to the receptor site, administering larger doses of an agonist can overcome the antagonist’s effects. A noncompetitive antagonist binds to receptor sites and blocks the effects of the agonist. Administering larger doses of the agonist can’t reverse the antagonist’s action. Pharmacotherapeutics Pharmacotherapeutics is the use of drugs to treat disease. When choosing a drug to treat a particular condition, health care providers consider not only the drug’s effectiveness but also other factors such as the type of therapy the patient will receive. The type of therapy a patient receives depends on the: severity urgency, and Prognosis of the patient’s condition and can include: o acute therapy, if the patient is critically ill and requires acute intensive therapy o empiric therapy, based on practical experience rather than on pure scientific data o maintenance therapy, for patients with chronic conditions that don’t resolve o supplemental or replacement therapy, to replenish or substitute for missing substances in the body o supportive therapy, which doesn’t treat the cause of the disease but maintains other threatened body systems until the patient’s condition resolves o palliative therapy, used for end-stage or terminal diseases to make the patient as comfortable as possible Drug interactions Drug interactions can occur between drugs or between drugs and foods. They can interfere with the results of a laboratory test or produce physical or chemical incompatibilities. The more drugs a patient receives, the greater the chances that a drug interaction will occur. Potential drug interactions include: Additive effects Potentiation Antagonistic effects Decreased or increased absorption Decreased or increased metabolism and excretion. 1. Additive effects o Can occur when two drugs with similar actions are administered to a patient. The effects are equivalent to the sum of either drug’s effects if it were administered alone in higher doses. o Giving two drugs together, such as two analgesics (pain relievers), has several potential advantages: lower doses of each drug, decreased probability of adverse reactions, and greater pain control than from one drug given alone (most likely because of different mechanisms of action). o There’s a decreased risk of adverse effects when giving two drugs for the same condition because the patient is given lower doses of each drug—the higher the dose, the greater the risk of adverse effects. 2. A synergistic effect, also called potentiation o Occurs when two drugs that produce the same effect are given together and one drug potentiates (enhances the effect of) the other drug. o This produces greater effects than when each drug is taken alone. 3. An antagonistic o Effect occurs when the combined response of two drugs is less than the response produced by either drug alone. o Two drugs given together can change the absorption of one or both of the drugs: o Drugs that change the acidity of the stomach can affect the ability of another drug to dissolve in the stomach. o Some drugs can interact and form an insoluble compound that can’t be absorbed. o Sometimes, an absorption-related drug interaction can be avoided by administering the drugs at least 2 hours apart After a drug is absorbed, the blood distributes it throughout the body as a free drug or one that’s bound to plasma protein. When two drugs are given together, they can compete for protein-binding sites, leading to an increase in the effects of one drug as that drug is displaced from the protein and becomes a free, unbound drug. Toxic drug levels can occur when a drug’s metabolism and excretion are inhibited by another drug. Some drug interactions affect excretion only. Adverse drug reactions A drug’s desired effect is called the expected therapeutic response. An adverse drug reaction (also called a side effect or adverse effect), on the other hand, is a harmful, undesirable response. Adverse drug reactions can range from mild ones that disappear when the drug is discontinued to debilitating diseases that become chronic. Adverse reactions can appear shortly after starting a new medication but may become less severe with time. 14 Rights in medication Administration 1. Right Drug/Medication 2. Right Client/Patient 3. Right Route 4. Right Dose 5. Right Frequency/Time 6. Right Assessment 7. Right Approach 8. Right Education 9. Right Evaluation 10. Right Documentation 10 Rights of Drug Administration 1. Right Drug The first right of drug administration is to check and verify if it’s the right name and form. Beware of look-alike and sound-alike medication names. Misreading medication names that look similar is a common mistake. These look-alike medication names may also sound alike and can lead to errors associated with verbal prescriptions. 2. Right Patient Ask the name of the client and check his/her ID band before giving the medication. Even if you know that patient’s name, you still need to ask just to verify. 3. Right Dose Check the medication sheet and the doctor’s order before medicating. Be aware of the difference between an adult and a pediatric dose. 4. Right Route Check the order if it’s oral, IV, SQ, IM, etc.. 5. Right Time and Frequency Check the order for when it would be given and when was the last time it was given. 6. Right Documentation Make sure to write the time and any remarks on the chart correctly. 7. Right History and Assessment Secure a copy of the client’s history to drug interactions and allergies. 8. Drug approach and Right to Refuse Give the client enough autonomy to refuse the medication after thoroughly explaining the effects. 9. Right Drug-Drug Interaction and Evaluation Review any medications previously given or the diet of the patient that can yield a bad interaction to the drug to be given. Check also the expiry date of the medication being given. 10. Right Education and Information Provide enough knowledge to the patient of what drug he/she would be taking and what are the expected therapeutic and side effects. 3. Common Board Topics a. Pharmacokinetics b. Pharmacotherapeutics c. Pharmacodynamics d. Drug interactions e. Drug interactions III. KEY POINTS FOR REVIEW Pharmacokinetics deals with a drug’s actions as it moves through the body. Drug absorption covers a drug’s progress from the time it’s administered, through its passage to the tissues, until it reaches systemic circulation. Passive transport requires no cellular energy because diffusion allows the drug to move from an area of higher concentration to one of lower concentration Active transport requires cellular energy to move the drug from an area of lower concentration to one of higher concentration. Absorption occurs at a slower rate when drugs are administered by the oral, I.M., or subQ routes because the complex membrane systems of GI mucosal layers, muscle, and skin delay drug passage. Drug distribution is the process by which the drug is delivered from the systemic circulation to body tissues and fluids Drug metabolism, or biotransformation, is the process by which the body changes a drug from its dosage form to a more water-soluble form that can then be excreted Drug excretion refers to the elimination of drugs from the body. Most drugs are excreted by the kidneys and leave the body through urine. Drugs can also be excreted through the lungs, exocrine (sweat, salivary, or mammary) glands, skin, and intestinal tract. Pharmacodynamics is the study of the drug mechanisms that produce biochemical or physiologic changes in the body. Pharmacotherapeutics is the use of drugs to treat disease. Drug interactions can occur between drugs or between drugs and foods. They can interfere with the results of a laboratory test or produce physical or chemical incompatibilities. The more drugs a patient receives, the greater the chances that a drug interaction will occur. IV. TEST YOUR KNOWLEDGE 1. While teaching a patient about drug therapy for diabetes, you review the absorption, distribution, metabolism, and excretion of insulin and oral antidiabetic agents. Which principle of pharmacology are you describing? A. Pharmacokinetics B. Pharmacodynamics C. Pharmacotherapeutics D. Drug potency 2. Which type of drug therapy is used for a patient who has a chronic condition that can’t be cured? A. Empiric therapy B. Acute therapy C. Maintenance therapy D. Supplemental therapy 3. Which branch of pharmacology studies the way drugs work in living organisms? A. Pharmacotherapeutics B. Pharmacokinetics C. Drug interactions D. Pharmacodynamics 4. Is the process by which the drug is delivered from the systemic circulation to body tissues and fluids. A. Drug distribution B. Drug metabolism C. Drug excretion D. Drug pharmacodynamics 5. Some drugs can interact and form an insoluble compound that can’t be absorbed. A. Antagonist B. Agonist C. Pharmacotherapeutics D. pharmacodynamics HANDOUT No.3: DRUGS AFFECTING THE BODY SYSTEM AGENTS AFFECTING CENTRAL NERVOUS SYSTEM (CHOLINERGIC and ANTICHOLINERGIC DRUGS) I. LEARNING OUTCOMES At the end of this lecture, the students should be able to: 5. Familiarize classes of drugs that affect the autonomic nervous system 6. Understand the uses and varying actions of these drugs 7. Understand these drugs are absorbed, distributed, metabolized, and excreted 8. Learn drug interactions and adverse effects of these drugs. II. CONTENT Cholinergic drugs Cholinergic drugs promote the action of the neurotransmitter acetylcholine. These drugs are also called parasympathomimetic drugs because they produce effects that imitate parasympathetic nerve stimulation. There are two major classes of cholinergic drugs: 1. Cholinergic agonists mimic the action of the neurotransmitter acetylcholine. 2. Anticholinesterase drugs work by inhibiting the destruction of acetylcholine at the cholinergic receptor sites. Cholinergic agonists Directly stimulating cholinergic receptors, cholinergic agonists mimic the action of the neurotransmitter acetylcholine. They include such drugs as: Bethanechol Carbachol Cevimeline Pilocarpine. Pharmacokinetics (how drugs circulate) The action and metabolism of cholinergic agonists vary widely and depend on the affinity of the individual drug for muscarinic or nicotinic receptors. No I.M. or I.V. o Injections Cholinergic agonists rarely are administered by I.M. or I.V. injection because they’re almost immediately broken down by cholinesterases in the interstitial spaces between tissues and inside the blood vessels. Moreover, they begin to work rapidly and can cause a cholinergic crisis (a drug overdose resulting in extreme muscle weakness and possibly paralysis of the muscles used in respiration). Cholinergic agonists are usually administered: Topically, with eye drops Orally By subcutaneous (subQ) injection. SubQ injections begin to work more rapidly than oral doses. Metabolism and excretion All cholinergic agonists are metabolized by cholinesterases: At the muscarinic and nicotinic receptor sites In the plasma (the liquid portion of the blood) In the liver. All drugs in this class are excreted by the kidneys. Pharmacodynamics (how drugs act) Cholinergic agonists work by mimicking the action of acetylcholine on the neurons in certain organs of the body called target organs. When they combine with receptors on the cell membranes of target organs, they stimulate the muscle and produce: Salivation Bradycardia (a slow heart rate) Dilation of blood vessels Constriction of the bronchioles Increased activity of the GI tract Increased tone and contraction of the bladder muscles Constriction of the pupils. Pharmacotherapeutics (how drugs are used) o Cholinergic agonists are used to: treat atonic (weak) bladder conditions and postoperative and postpartum urine retention treat GI disorders, such as postoperative abdominal distention and GI atony reduce eye pressure in patients with glaucoma and during eye surgery treat salivary gland hypofunction caused by radiation therapy or Sjogren’s syndrome. Drug interactions o Cholinergic agonists have specific interactions with other drugs. Examples include the following: Other cholinergic drugs, particularly anticholinesterase drugs (such as ambenonium, edrophonium, neostigmine, physostigmine, and pyridostigmine), boost the effects of cholinergic agonists and increase the risk of toxicity. Cholinergic blocking drugs (such as atropine, belladonna, homatropine, methantheline, methscopolamine, propantheline, and scopolamine) reduce the effects of cholinergic drugs. Quinidine also reduces the effectiveness of cholinergic agonists. Anticholinesterase drugs Anticholinesterase drugs Block the action of the enzyme acetylcholinesterase (which breaks down the neurotransmitter acetylcholine) at cholinergic receptor sites, preventing the breakdown of acetylcholine. As acetylcholine builds up, it continues to stimulate the cholinergic receptors. Pharmacokinetics Here’s a brief rundown of how anticholinesterase drugs move through the body. o Many of the anticholinesterase drugs are readily absorbed from the GI tract, subcutaneous tissue, and mucous membranes. Because neostigmine is poorly absorbed from the GI tract, the patient needs a higher dose when taking this drug orally. o Because the duration of action for an oral dose is longer, however, the patient doesn’t need to take it as frequently. o When a rapid effect is needed, neostigmine should be given by the I.M. or I.V. route. Metabolism and excretion Most anticholinesterase drugs are metabolized by enzymes in the plasma and excreted in urine. Donepezil Galantamine Rivastigmine Tacrine are metabolized in the liver Pharmacodynamics Anticholinesterase drugs promote the action of acetylcholine at receptor sites. Depending on the site and the drug’s dose and duration of action, they can produce a stimulant or depressant effect on cholinergic receptors. Cholinergic blocking drugs Cholinergic blocking drugs interrupt parasympathetic nerve impulses in the central and autonomic nervous systems. These drugs are also referred to as anticholinergic drugs because they prevent acetylcholine from stimulating cholinergic receptors. Cholinergic blocking drugs don’t block all cholinergic receptors, just the muscarinic receptor sites. Muscarinic receptors are cholinergic receptors that are stimulated by the alkaloid muscarine and blocked by atropine. The major cholinergic blocking drugs are the belladonna alkaloids: Atropine (the prototype cholinergic blocking drug) Belladonna homatropine hyoscyamine sulfate Methscopolamine Scopolamine Pharmacokinetics Here’s how cholinergic blockers move through the body: Absorption The belladonna alkaloids are absorbed from the: Eyes GI tract Mucous membranes Skin Distribution The belladonna alkaloids are distributed more widely throughout the body than the quaternary ammonium derivatives or dicyclomine. The alkaloids readily cross the blood-brain barrier; the other cholinergic blockers don’t. Metabolism and excretion The belladonna alkaloids are only slightly to moderately protein bound. This means that a moderate to high amount of the drug is active and available to produce a therapeutic response. The belladonna alkaloids are metabolized in the liver and excreted by the kidneys as unchanged drug and metabolites. Pharmacotherapeutics Cholinergic blockers are often used to treat GI disorders and complications. All cholinergic blockers are used to treat spastic or hyperactive conditions of the GI and urinary tracts because they relax muscles and decrease GI secretions. These drugs may be used to relax the bladder and to treat urinary incontinence. Cholinergic blockers such as atropine are given before surgery to: Reduce oral, gastric, and respiratory secretions Prevent a drop in heart rate caused by vagal nerve stimulation during anesthesia. Drugs that decrease the effects of cholinergic blockers include: 1. cholinergic agonists such as bethanechol 2. anticholinesterase drugs, such as neostigmine and pyridostigmine. Common Board Topics Adverse reactions to cholinergic blockers. Adverse reactions resulting from cholinergic blockers are closely related to the drug dose. With these drugs, the difference between a therapeutic dose and a toxic dose is small. Adverse reactions may include: Dry mouth Reduced bronchial secretions Increased heart rate Decreased sweating. How atropine speeds the heart rate: Atropine, a cholinergic blocking drug, competes with acetylcholine for cholinergic receptor sites on the SA and AV nodes. By blocking acetylcholine, atropine speeds up the heart rate. Adverse reactions to anticholinesterase drugs Most of the adverse reactions caused by anticholinesterase drugs result from increased action of acetylcholine at receptor sites. Adverse reactions associated with these drugs include: Cardiac arrhythmias Nausea and vomiting Diarrhea Shortness of breath, wheezing, or tightness in the chest Seizures Headache Anorexia Insomnia Pruritus Urinary frequency and nocturia. III. KEY POINTS FOR REVIEW (This is the highlight or summary of your discussions to this handout) Cholinergic drugs Cholinergic drugs promote the action of the neurotransmitter acetylcholine. These drugs are also called parasympathomimetic drugs because they produce effects that imitate parasympathetic nerve stimulation. Cholinergic agonists Directly stimulating cholinergic receptors, cholinergic agonists mimic the action of the neurotransmitter acetylcholine. Anticholinesterase drugs Block the action of the enzyme acetylcholinesterase (which breaks down the neurotransmitter acetylcholine) at cholinergic receptor sites, preventing the breakdown of acetylcholine. Cholinergic blocking drugs Cholinergic blocking drugs interrupt parasympathetic nerve impulses in the central and autonomic nervous systems. These drugs are also referred to as anticholinergic drugs because they prevent acetylcholine from stimulating cholinergic receptors. IV. TEST YOUR KNOWLEDGE 1. During bethanechol therapy, which common adverse reactions should you expect to observe? A. Dry mouth, flushed face, and constipation B. Fasciculations, dysphagia, and respiratory distress C. Nausea, vomiting, diarrhea, and intestinal cramps D. Anorexia, cardiac arrhythmias, fatigue, and bronchospasm 2. Catecholamines act as potent inotropes. That means that they: A. Causes the heart to contract forcefully. B. Slows the heart rate. C. Lower blood pressure. D. Decrease urinary output. 3. Noncatecholamines can interact with monoamine oxidase inhibitors to cause: A. Arrhythmias B. Severe hypertension C. Seizures. D. Tachycardia 4. Beta-adrenergic blockers have widespread effects because they produce their blocking action in the: A. Hypothalamus B. Anterior pituitary gland C. Pituitary gland D. Adrenal medulla 5. Block the action of the enzyme acetylcholinesterase (which breaks down the neurotransmitter acetylcholine) at cholinergic receptor sites, preventing the breakdown of acetylcholine. A. Anticholinesterase B. Cholinergic blockers C. Adrenergic drugs D. Betablockers HANDOUT No.4: DRUGS AFFECTING THE RESPIRATORY SYSTEM I. LEARNING OUTCOMES At the end of this lecture, the students should be able to: 9. Recognize classes of drugs used to treat respiratory disorders 10. Identify uses and varying actions of these drugs 11. Describe how these drugs are absorbed, distributed, metabolized, and excreted 12. Evaluate drug interactions and adverse reactions to these drugs. II. CONTENT Drugs and the respiratory system The respiratory system, extending from the nose to the pulmonary capillaries, performs the essential function of gas exchange between the body and its environment. In other words, it takes in oxygen and expels carbon dioxide. Drugs used to improve respiratory symptoms are available in inhalation and systemic formulations. These drugs include: Beta2-adrenergic agonists Anticholinergic Corticosteroids Leukotriene modifiers Mast cell stabilizers Methylxanthines Monoclonal antibodies Expectorants Antitussives Mucolytics Decongestants. Beta2-adrenergic agonists Beta2-adrenergic agonists are used to treat symptoms associated with asthma and chronic obstructive pulmonary disease (COPD). Drugs in this class can be either short-acting or long-acting. Short-acting beta2-adrenergic agonists include: Albuterol (systemic, inhalation) Levalbuterol (inhalation) Metaproterenol (inhalation) Pirbuterol (inhalation) Terbutaline (systemic) Long-acting beta2-adrenergic agonists include: Formoterol (inhalation) salmeterol (inhalation). Pharmacokinetics (how drugs circulate) Beta2-adrenergic agonists are minimally absorbed from the GI tract; inhaled forms exert their effects locally. After inhalation, beta2-adrenergic agonists appear to be absorbed over several hours from the respiratory tract. These drugs don’t cross the blood-brain barrier; they’re extensively metabolized in the liver to inactive compounds and rapidly excreted in urine and stool. Pharmacodynamics (how drugs act) Beta2-adrenergic agonists increase levels of cyclic adenosine monophosphate by stimulating the beta2-adrenergic receptors in the smooth muscle, resulting in bronchodilation. These drugs may lose their selectivity at higher doses, which can increase the risk of toxicity. Inhaled forms are preferred because they act locally in the lungs, resulting in fewer adverse reactions than systemically absorbed forms. Pharmacotherapeutics (how drugs are used) Short-acting inhaled beta2-adrenergic agonists are the drugs of choice for fast relief of symptoms in the patient with asthma. They’re generally used as needed for asthma (including exercise-induced asthma) and COPD. A patient with COPD may use them around-the-clock on a specified schedule. However, excessive use of a short-acting beta2-adrenergic agonist Anticholinergics Inhaled ipratropium, an anticholinergic, is a bronchodilator used primarily in the patient suffering from COPD, but it may also be used as an adjunct to beta2-adrenergic agonists. Ipratropium Ipratropium is the most common anticholinergic used for respiratory disorders. Ipratropium inhibits muscarinic receptors, which results in bronchodilation. This drug works by blocking the parasympathetic nervous system, rather than stimulating the sympathetic nervous system. Anticholinergics are used to relieve symptoms in the patient with COPD. They’re less effective in long-term management of the patient with asthma; however, they may be used as adjunctive therapy (usually in combination with a short-acting beta2-adrenergic agonist on a scheduled basis). Corticosteroids Corticosteroids are anti-inflammatory drugs available in inhaled and systemic forms for the short- and long-term control of asthma symptoms. Many products with differing potencies are available. Inhaled corticosteroids include: Beclomethasone dipropionate Budesonide Flunisolide Fluticasone Triamcinolone acetonide. Oral corticosteroids include: Prednisolone Prednisone. I.V. corticosteroids include: Hydrocortisone sodium succinate Methylprednisolone sodium succinate. Pharmacokinetics Oral prednisone is readily absorbed and extensively metabolized in the liver to the active metabolite prednisolone. The I.V. form has a rapid onset. Inhaled drugs are minimally absorbed, although absorption increases as the dosage is increased. Pharmacodynamics Corticosteroids work by inhibiting the production of cytokines, leukotrienes, and prostaglandins; the recruitment of eosinophils; and the release of other inflammatory mediators. They also affect other areas in the body, which can cause long-term adverse reactions. Pharmacotherapeutics Corticosteroids are the most effective drugs available for the longterm treatment and prevention of acute asthma attacks. Drug interactions Interactions are uncommon when using inhaled forms. Hormonal contraceptives, ketoconazole, and macrolide antibiotics may increase the activity of corticosteroids in general, resulting in the need to decrease the steroid dosage. Barbiturates, cholestyramine, rifampin, and phenytoin may decrease the effectiveness of corticosteroids, resulting in the need to increase the steroid dosage. Leukotriene modifiers Leukotriene modifiers are used for the prevention and long-term control of mild asthma. Leukotriene receptor antagonists include: Montelukast Zafirlukast. Leukotriene formation inhibitors include: Zileuton Pharmacokinetics Montelukast is rapidly absorbed. Z Afirlukast’s absorption is decreased by food, so it should be given 1 hour before or 2 hours after meals. All of the leukotriene modifiers are highly protein-bound (more than 90%). Metabolism and excretion Zafirlukast is extensively metabolized in the liver by the cytochrome P450 2C9 (CYP2C9) enzyme into inactive metabolites and excreted primarily in stool. In general, this class of drugs is metabolized, induced, or inhibited by the cytochrome P450 enzyme system, which is important for establishing drug interactions. Caution required Zileuton is contraindicated in the patient with active liver disease. Closely monitor the patient with liver impairment whose taking zafirlukast for adverse reactions; he may require a dosage adjustment. This doesn’t apply for montelukast. Pharmacodynamics Leukotrienes are substances released from mast cells, eosinophils, and basophils that can cause smooth-muscle contraction of the airways, increased permeability of the vasculature, increased secretions, and activation of other inflammatory mediators. Leukotrienes may be inhibited by two different mechanisms. The leukotriene receptor antagonist’s zafirlukast and montelukast prevent the D4 and E4 leukotrienes from interacting with their receptors, thereby blocking their action. The leukotriene formation inhibitor zileuton inhibits the production of 5- lipoxygenase, thereby preventing the formation of leukotrienes. Pharmacotherapeutics Leukotriene modifiers are primarily used to prevent and control asthma attacks in the patient with mild to moderate disease. Montelukast is also indicated for the treatment of allergic rhinitis. Drug interactions Zafirlukast inhibits CYP2C9 and thus could increase the risk of toxicity if used with phenytoin or warfarin. Zafirlukast and zileuton inhibit CYP3A4 and thus could increase the risk of toxicity if used with amlodipine, atorvastatin, carbamazepine, clarithromycin, cyclosporine, erythromycin, hormonal contraceptives, itraconazole, ketoconazole, lovastatin, nelfinavir, nifedipine, ritonavir, sertraline, simvastatin, or warfarin. Zileuton inhibits CYP1A2 and thus could increase the risk of toxicity if used with amitriptyline, clozapine, desipramine, fluvoxamine, imipramine, theophylline, or warfarin. Zafirlukast, zileuton, and montelukast are metabolized by CYP2C9 and thus could increase the risk of toxicity if used with amiodarone, cimetidine, fluconazole, fluoxetine, fluvoxamine, isoniazid, metronidazole, or voriconazole. If carbamazepine, phenobarbital, phenytoin, primidone, or rifampin is used with leukotrienes, the effectiveness of the leukotrienes could be reduced. Zileuton and montelukast are metabolized by CYP3A4 and thus could increase the risk of toxicity if used with amiodarone, cimetidine, clarithromycin, cyclosporine, erythromycin, fluoxetine, fluvoxamine, grapefruit juice, itraconazole, ketoconazole, metronidazole, or voriconazole and could result in decreased effectiveness if used with carbamazepine, efavirenz, garlic supplements, modafinil, nevirapine, oxcarbazepine, phenobarbital, phenytoin, primidone, rifabutin, rifampin, or St. John’s wort. Methylxanthines Methylxanthines, also called xanthines, are used to treat respiratory disorders. Types of methylxanthines Methylxanthines include anhydrous theophylline and its derivative salt aminophylline. Theophylline is the most commonly prescribed oral methylxanthine. Aminophylline is preferred when an I.V. methylxanthine is required. Caffeine is also a xanthine derivative. Pharmacokinetics The pharmacokinetics of methylxanthines vary according to which drug the patient is receiving, the dosage form, and the administration route. Absorption When theophylline is given as an oral solution or a rapid-release tablet, it’s absorbed rapidly and completely. High-fat meals can increase theophylline concentrations and the risk of toxicity Gastric measures Absorption of some of theophylline’s slow-release forms depends on the gastric pH. Food can alter absorption. When converting the patient from I.V. aminophylline to oral theophylline, the dosage is decreased by 20%. Metabolism and excretion Theophylline is metabolized primarily in the liver by the CYP1A2 enzyme. In adults and children, about 10% of a dose is excreted unchanged in urine; therefore, no dosage adjustment is required in patients with renal insufficiency. Elderly patients and those with liver dysfunction may require a lower dose Pharmacotherapeutics Theophylline and its salts are used as second- or third-line therapy for the long-term control and prevention of symptoms related to: Asthma Chronic bronchitis Emphysema. Useful for neonates? Theophylline has been used to treat neonatal apnea (periods of not breathing in the neonate) and has been effective in reducing severe bronchospasm in an infant with cystic fibrosis. Drug interactions Inhibitors of the CYP1A2 enzyme (including cimetidine, ciprofloxacin, clarithromycin, erythromycin, fluvoxamine, hormonal contraceptives, isoniazid, ketoconazole, ticlopidine, and zileuton) decrease theophylline metabolism, thus increasing its serum level as well as the risk of adverse reactions and toxicity. The dosage of theophylline may need to be reduced. Inducers of the CYP1A2 enzyme (including carbamazepine, phenobarbital, phenytoin, rifampin, St. John’s wort, and charbroiled meats) increase theophylline metabolism, thus decreasing its serum level and possibly its effectiveness. The dosage of theophylline may need to be increased. Smoking cigarettes or marijuana increases theophylline elimination, thus decreasing its serum level and effectiveness. Taking adrenergic stimulants or drinking beverages that contain caffeine or caffeinelike substances may result in additive adverse reactions to theophylline or signs and symptoms of methylxanthine toxicity. Activated charcoal may decrease theophylline levels. The use of enflurane or isoflurane with theophylline or theophylline derivatives increases the risk of cardiac toxicity. Theophylline and its derivatives may reduce the effects of lithium by increasing its rate of excretion. Thyroid hormones may reduce theophylline levels; antithyroid drugs may increase theophylline levels. Expectorants Expectorants thin mucus so it’s cleared more easily out of the airways. They also soothe mucous membranes in the respiratory tract. The result is a more productive cough. Guaifenesin The most commonly used expectorant is guaifenesin Pharmacokinetics Guaifenesin is absorbed through the GI tract, metabolized by the liver, and excreted primarily by the kidneys. Pharmacodynamics By increasing production of respiratory tract fluids, expectorants reduce the thickness, adhesiveness, and surface tension of mucus, making it easier to clear from the airways. Expectorants also provide a soothing effect on the mucous membranes of the respiratory tract. Pharmacotherapeutics Guaifenesin is used to relieve symptoms due to ineffective, productive coughs from many disorders, such as: Bronchial asthma Bronchitis Colds Emphysema Influenza Minor bronchial irritation Sinusitis Drug interactions Guaifenesin isn’t known to have specific drug interactions; however, it does cause some adverse reactions. Antitussives Antitussive drugs suppress or inhibit coughing Antitussives are typically used to treat dry, nonproductive coughs. The major antitussives include: benzonatate codeine dextromethorphan hydrocodone bitartrate. Pharmacokinetics Antitussives are absorbed well through the GI tract, metabolized in the liver, and excreted in urine. Pharmacodynamics Antitussives act in slightly different ways. Removing the sensation Benzonatate acts by anesthetizing stretch receptors throughout the bronchi, alveoli, and pleurae. Codeine, dextromethorphan, and hydrocodone suppress the cough reflex by direct action on the cough center in the medulla of the brain, thus lowering the cough threshold. Pharmacotherapeutics The uses of these drugs vary slightly, but each treats a serious, nonproductive cough that interferes with a patient’s ability to rest or carry out activities of daily living Benzonatate relieves cough caused by pneumonia, bronchitis, the common cold, and chronic pulmonary diseases such as emphysema. It can also be used during bronchial diagnostic tests, such as bronchoscopy, when the patient must avoid coughing. Dextromethorphan is the most widely used cough suppressant and may provide better antitussive effects than codeine. Its popularity may stem from the fact that it isn’t associated with sedation, respiratory depression, or addiction at usual doses. For really tough coughs The opioid antitussives (typically codeine and hydrocodone) are reserved for treating an intractable cough. Drug interactions Antitussives may interact with other drugs. Codeine and hydrocodone may cause excitation, an extremely elevated temperature, hypertension or hypotension, and coma when taken with monoamine oxidase inhibitors (MAOIs). Dextromethorphan use with MAOIs may produce excitation, an elevated body temperature, hypotension, and coma. Codeine taken with other central nervous system (CNS) depressants, including alcohol, barbiturates, phenothiazines, and sedative-hypnotics, may increase CNS depression, resulting in drowsiness, lethargy, stupor, respiratory depression, coma, and even death. Mucolytics Mucolytics act directly on mucus, breaking down sticky, thick secretions so that they’re more easily eliminated. Acetylcysteine Acetylcysteine is the only mucolytic used clinically in the United States for the patient with abnormal or thick mucus. Pharmacokinetics Inhaled acetylcysteine is absorbed from the pulmonary epithelium. When taken orally, the drug is absorbed from the GI tract. Metabolism and excretion Acetylcysteine is metabolized in the liver; its excretion is unknown. Pharmacodynamics Acetylcysteine decreases the thickness of respiratory tract secretions by altering the molecular composition of mucus. It also irritates the mucosa to stimulate clearance and restores glutathione, a substance that plays an important role in oxidation-reduction processes. Liver cleaner Glutathione’s enzymatic action in the liver reduces acetaminophen toxicity from overdose. Pharmacotherapeutics Mucolytics are used with other therapies to treat the patient with abnormal or thick mucus secretions, such as the patient with: Atelectasis caused by mucus obstruction, as may occur in pneumonia, bronchiectasis, or chronic bronchitis Bronchitis Pulmonary complications related to cystic fibrosis Mucolytics may also be used to prepare the patient for bronchography and other bronchial studies. Acetylcysteine is the antidote for acetaminophen overdose. However, it doesn’t fully protect against liver damage caused by acetaminophen toxicity. Drug interactions Activated charcoal decreases acetylcysteine’s effectiveness. When using acetylcysteine to treat an acetaminophen overdose. Decongestants Decongestants may be classified as systemic or topical, depending on how they’re administered. Types of decongestants As sympathomimetic drugs, systemic decongestants stimulate the sympathetic nervous system to reduce swelling of the respiratory tract’s vascular network. Systemic decongestants include: Ephedrine Phenylephrine Pseudoephedrine Pharmacokinetics The pharmacokinetic properties of decongestants vary When taken orally, the systemic decongestants are absorbed readily from the GI tract and widely distributed throughout the body into various tissues and fluids, including cerebrospinal fluid, the placenta, and breast milk. Pharmacodynamics The properties of systemic and topical decongestants vary slightly. Systemic decongestants cause vasoconstriction by stimulating alpha-adrenergic receptors in the blood vessels of the body. This reduces the blood supply to the nose, which decreases swelling of the nasal mucosa. They also cause contraction of urinary and GI sphincters, dilated pupils, and decreased insulin secretion. These drugs may also act indirectly, causing the release of norepinephrine Pharmacotherapeutics Systemic and topical decongestants are used to relieve the symptoms of swollen nasal membranes resulting from: Acute coryza (profuse discharge from the nose) Allergic rhinitis (hay fever) The common cold Sinusitis Vasomotor rhinitis. Storage sites in the body, which results in peripheral vasoconstriction. Drug interactions Because they produce vasoconstriction, which reduces drug absorption, topical decongestants seldom produce drug interactions. Common Board Topics Drug interactions of decongestants Activated charcoal decreases acetylcysteine’s effectiveness. When using acetylcysteine to treat an acetaminophen overdose. Acetylcysteine decreases the thickness of respiratory tract secretions by altering the molecular composition of mucus. Guaifenesin is used to relieve symptoms due to ineffective, productive coughs from many disorders Codeine, dextromethorphan, and hydrocodone suppress the cough reflex by direct action on the cough center in the medulla of the brain, thus lowering the cough threshold. Theophylline has been used to treat neonatal apnea (periods of not breathing in the neonate) and has been effective in reducing severe bronchospasm in an infant with cystic fibrosis. Leukotrienes are substances released from mast cells, eosinophils, and basophils that can cause smooth-muscle contraction of the airways, increased permeability of the vasculature, increased secretions, and activation of other inflammatory mediators. III. KEY POINTS FOR REVIEW Adverse reactions to beta2- adrenergic agonists. Adverse reactions to short-acting beta2- adrenergic agonists include: Paradoxical bronchospasm Tachycardia Palpitations Tremors Dry mouth Adverse reactions to long-acting beta2- adrenergic agonists include: Bronchospasm Tachycardia Palpitations Hypertension Tremors Adverse reactions to anticholinergics The most common adverse reactions to anticholinergics include: Nervousness Tachycardia Nausea and vomiting Paradoxical bronchospasm (with excessive use) Dry mouth. These special populations may require special care when taking corticosteroids: Children: Growth should be monitored, especially when they’re taking systemic drugs or higher doses of inhaled drugs. Elderly patients: May benefit from receiving drugs that prevent osteoporosis, such as alendronate during therapy with corticosteroids, especially if they’re taking higher doses of inhaled or systemic steroids. Patients with diabetes: May require closer monitoring of their blood glucose levels while on steroids. Breast-feeding women: Corticosteroid levels are negligible in the breast milk of mothers who take less than 20 mg/day of oral prednisone. The amount found in breast milk can be minimized if the woman waits at least 4 hours after taking prednisone to breast-feed her infant. Adverse reactions to inhaled corticosteroids. Adverse reactions to inhaled corticosteroids may include: Mouth irritation Oral candidiasis Upper respiratory tract infection. To reduce the risk of adverse reactions from inhaled steroids, the patient should use the lowest possible dosage to maintain control, administer doses using a spacer, and rinse out his mouth after administration. Adverse reactions to leukotriene modifiers Adverse reactions that may occur with leukotriene modifiers include: Headache Dizziness Nausea and vomiting Myalgia. Memory jogger. How can you remember what theophylline and its salts are used to treat? Simple: Just remember that you really need to “ACE” this one! It’s used for long-term control and prevention of symptoms related to: A— asthma C—chronic bronchitis E—emphysema. Adverse reactions to guaifenesin. Adverse reactions to guaifenesin include: Nausea and vomiting (if taken in large doses) Diarrhea Abdominal pain Drowsiness Headache Hives Rash Adverse reactions to acetylcysteine During administration, acetylcysteine has a “rotten egg” odor that may cause nausea. With prolonged or persistent use, acetylcysteine may produce: Bronchospasm Drowsiness Nausea and vomiting Severe runny nose Stomatitis Acetylcysteine isn’t recommended for the patient with asthma because it may cause bronchospasm. IV. TEST YOUR KNOWLEDGE 1. Which adverse reaction can occur if guaifenesin is taken in larger doses than necessary? A. Constipation B. Vomiting C. Insomnia D. Diarrhea 2. Which medication should the patient avoid when taking dextromethorphan? A. Acetaminophen B. Guaifenesin C. Phenelzine D. Famotidine 3. Besides bronchitis, acetylcysteine may also be used to treat: A. Acetaminophen overdose. B. Severe rhinorrhea. C. Stomatitis. D. Diarrhea. 4. Which adverse reaction most commonly occurs with a decongestant, such as tetrahydrozoline, especially if it’s taken more often than recommended? A. Nausea B. Dizziness C. Diarrhea D. Rebound nasal congestion 5.Acetylciteine causes nausea and vomiting because: A. Smells like a rotten egg B. Decreases appetite C. Increases gastric acid D. Causes B-complex to diminish HANDOUT No. 5 CARDIOVASCULAR SYSTEM I. LEARNING OUTCOMES At the end of this lecture, the students should be able to: 13. List classes of drugs used to treat cardiovascular disorders 14. Classify the uses and varying actions of these drugs 15. Describe how these drugs are absorbed, distributed, metabolized, and excreted 16. Recognize drug interactions and adverse reactions to these drugs. II. CONTENT Drugs and the cardiovascular system The heart, arteries, veins, and lymphatics make up the cardiovascular system. These structures transport life-supporting oxygen and nutrients to cells, remove metabolic waste products, and carry hormones from one part of the body to another. Because this system performs such vital functions, a problem with the heart or blood vessels can seriously affect a person’s health. Types of drugs used to improve cardiovascular function include: Inotropic Antiarrhythmic Anti-anginal Antihypertensive Diuretic Antilipidemic Inotropics Inotropic drugs, such as cardiac glycosides and phosphodiesterase (PDE) inhibitors, increase the force of the heart’s contractions. In other words, the drugs have what’s known as a Cardiovascular drugs Just the facts In this chapter, you’ll learn: ♦ Classes of drugs used to treat cardiovascular disorders ♦ Uses and varying actions of these drugs ♦ How these drugs are absorbed, distributed, metabolized, and excreted ♦ Drug interactions and adverse reactions to these drugs. Positive inotropic effect. (Inotropic means affecting the force or energy of muscular contractions.) Cardiac glycosides also slow the heart rate (called a negative chronotropic effect) and slow electrical impulse conduction through the atrioventricular (AV) node (called a negative dromotropic effect). Cardiac glycosides Cardiac glycosides are a group of drugs derived from digitalis, a substance that occurs naturally in foxglove plants and in certain toads. The most frequently used cardiac glycoside is digoxin. Pharmacokinetics (how drugs circulate) The intestinal absorption of digoxin varies greatly; the capsules are absorbed most efficiently, followed by the elixir form, and then tablets. Digoxin is distributed widely throughout the body, with highest concentrations in the heart muscle, liver, and kidneys. Digoxin binds poorly to plasma proteins. In most patients, a small amount of digoxin is metabolized in the liver and gut by bacteria. This effect varies and may be substantial in some people. Most of the drug is excreted by the kidneys as unchanged drug. Pharmacodynamics (how drugs act) Digoxin is used to treat heart failure because it strengthens the contraction of the ventricles by boosting intracellular calcium at the cell membrane, enabling stronger heart contractions. Digoxin may also enhance the movement of calcium into the myocardial cells and stimulate the release, or block the reuptake, of norepinephrine at the adrenergic nerve terminal. Digoxin Acts on the central nervous system (CNS) to slow the heart rate, thus making it useful for treating supraventricular arrhythmias (abnormal heart rhythms that originate above the bundle branches of the heart’s conduction system), such as atrial fibrillation and atrial flutter. It also increases the refractory period (the period when the cells of the conduction system can’t conduct an impulse). Pharmacotherapeutics (how drugs are used) In addition to treating heart failure and supraventricular arrhythmias, digoxin is used to treat paroxysmal atrial tachycardia (an arrhythmia marked by brief periods of tachycardia that alternate with brief periods of sinus rhythm). Drug interactions Many drugs can interact with digoxin. Antacids, barbiturates, cholestyramine resin, kaolin and pectin, neomycin, metoclopramide, rifampin, and sulfasalazine reduce the therapeutic effects of digoxin. Calcium preparations, quinidine, verapamil, cyclosporine, tetracycline, clarithromycin, propafenone, amiodarone, spironolactone, hydroxychloroquine, erythromycin, itraconazole, and omeprazole increase the risk of digoxin toxicity. Amphotericin B, potassium-wasting diuretics, and steroids taken with digoxin may cause hypokalemia (low potassium levels) and increase the risk of digoxin toxicity. Beta-adrenergic blockers and calcium channel blockers taken with digoxin may cause an excessively slow heart rate and arrhythmias. Succinylcholine and thyroid preparations increase the risk of arrhythmias when they’re taken with digoxin. St. John’s wort, an herbal preparation, can increase digoxin levels and risk of toxicity. Antiarrhythmic drugs Antiarrhythmic drugs are used to treat arrhythmias, disturbances of the normal heart rhythm. Unfortunately, many antiarrhythmics are also capable of worsening or causing the very arrhythmias they’re supposed to treat. The benefits need to be weighed carefully against the risks of antiarrhythmic therapy. Antiarrhythmics are categorized into four classes: I (which includes classes IA, IB, and IC) II III IV Class I antiarrhythmics Class I antiarrhythmics consist of sodium channel blockers. This is the largest group of antiarrhythmics. Class I agents are frequently subdivided into classes IA, IB, and IC. One drug, adenosine (an AV nodal blocking agent used to treat paroxysmal supraventricular tachycardia), doesn’t fall into any of these classes. The mechanisms of action of antiarrhythmics vary widely, and a few drugs exhibit properties common to more than one class. Class IA antiarrhythmics Class IA antiarrhythmics are used to treat a wide variety of atrial and ventricular arrhythmias. Class IA antiarrhythmics include: Disopyramide Procainamide Quinidine (sulfate and gluconate). Pharmacokinetics When administered orally, class IA drugs are rapidly absorbed and metabolized. Because they work so quickly, sustained-release forms of these drugs were developed to help maintain therapeutic levels. These drugs are distributed through all body tissues. Quinidine, however, is the only one that crosses the blood-brain barrier. All class IA antiarrhythmics are metabolized in the liver and are excreted unchanged by the kidneys. Acidic urine increases the excretion of quinidine. Pharmacodynamics Class IA antiarrhythmics control arrhythmias by altering the myocardial cell membrane and interfering with autonomic nervous system control of pacemaker cells. No (para)sympathy Class IA antiarrhythmics also block parasympathetic stimulation of the sinoatrial (SA) and AV nodes. Because stimulation of the parasympathetic nervous system causes the heart rate to slow down, drugs that block the parasympathetic nervous system increase the conduction rate of the AV node. Rhythmic risks. This increase in the conduction rate can produce dangerous increases in the ventricular heart rate if rapid atrial activity is present, as in a patient with atrial fibrillation. In turn, the increased ventricular heart rate can offset the ability of the antiarrhythmics to convert atrial arrhythmias to a regular rhythm. Pharmacotherapeutics Class IA antiarrhythmics are prescribed to treat such arrhythmias as premature ventricular contractions, ventricular tachycardia, atrial fibrillation, atrial flutter, and paroxysmal atrial tachycardia. Drug interactions Class IA antiarrhythmics Can interact with other drugs: Disopyramide taken with macrolide antibiotics, such as clarithromycin and erythromycin, increases the patient’s risk of developing a prolonged QT interval. In turn, this may lead to an increased risk of arrhythmias, especially polymorphic ventricular tachycardia. Disopyramide plus verapamil may increase myocardial depression and should be avoided in patients with heart failure. Other antiarrhythmics, such as beta-adrenergic blockers, increase the risk of arrhythmias. Quinidine plus neuromuscular blockers may cause increased skeletal muscle relaxation. Quinidine increases the risk of digoxin toxicity. Rifampin, phenytoin, and phenobarbital can reduce the effects of quinidine and disopyramide. GI symptoms are a common adverse reaction to class IA antiarrhythmics. Class IB antiarrhythmics Class IB antiarrhythmics are used for treating acute ventricular arrhythmias. They include: Lidocaine Mexiletine. Pharmacokinetics Mexiletine is absorbed from the GI tract after oral administration. Lidocaine is administered I.V. to prevent rapid metabolism by the liver after it enters the hepatic portal circulation. Lidocaine is distributed widely throughout the body, including the brain. Lidocaine and mexiletine are moderately bound to plasma proteins. (Remember, only that portion of a drug that’s unbound can produce a response.) Class IB antiarrhythmics are metabolized in the liver and excreted in urine. Mexiletine also appears in breast milk. Pharmacodynamics Class IB drugs work by blocking the rapid influx of sodium ions during the depolarization phase of the heart’s depolarization- repolarization cycle. This decreases the refractory period, which reduces the risk of arrhythmia. Because class IB antiarrhythmics especially affect the Purkinje fibers (fibers in the conducting system of the heart) and myocardial cells in the ventricles, they’re used to treat only ventricular arrhythmias. Pharmacotherapeutics Class IB antiarrhythmics are used to treat ventricular ectopic beats, ventricular tachycardia, and ventricular fibrillation. Class IB antiarrhythmics are usually the drug of choice in acute care because they don’t produce immediate serious adverse reactions. Drug interactions Class IB antiarrhythmics may exhibit additive or antagonistic effects when administered with other anti-arrhythmics, such as: phenytoin, propranolol, procainamide, and quinidine. Other drug interactions include the following: Rifampin may reduce the effects of mexiletine. Theophylline levels increase when given with mexiletine. Use of a beta-adrenergic blocker or disopyramide with mexiletine may reduce the contractility of the heart. Class IC antiarrhythmics Class IC antiarrhythmics are used to treat certain severe, refractory (resistant) ventricular arrhythmias. Class IC antiarrhythmics include: Flecainide Moricizine Propafenone. Pharmacokinetics After oral administration, class IC antiarrhythmics are absorbed well, distributed in varying degrees, and probably metabolized by the liver. They’re excreted primarily by the kidneys, except for propafenone, which is excreted primarily in stool. Pharmacodynamics Class IC antiarrhythmics primarily slow conduction along the heart’s conduction system. Moricizine decreases the fast inward current of sodium ions of the action potential, depressing the depolarization rate and the effective refractory period. Pharmacotherapeutics Like class IB antiarrhythmics, class IC antiarrhythmics are used to treat and prevent life-threatening ventricular arrhythmias. They’re also used to treat supraventricular arrhythmias (abnormal heart rhythms that originate above the bundle branches of the heart’s conduction system). Flecainide and propafenone may also be used to prevent paroxysmal supraventricular tachycardia (PSVT) in patients without structural heart disease. Moricizine is used to manage life-threatening ventricular arrhythmias such as sustained ventricular tachycardia Drug interactions Class IC antiarrhythmics may exhibit additive effects with other antiarrhythmics. Other interactions include the following: When used with digoxin, flecainide and propafenone increase the risk of digoxin toxicity. Propafenone increases plasma concentrations of warfarin and increases prothrombin times. Quinidine increases the effects of propafenone. Cimetidine may increase the plasma level and the risk of toxicity of moricizine. Propanolol or digoxin given with moricizine may increase the PR interval on the electrocardiogram. Theophylline levels may be reduced in a patient receiving moricizine. Ritonavir increases the plasma concentration and the effects of propafenone. Propafenone increases the serum concentration and the effects of metoprolol and propranolol. Class II antiarrhythmics Class II antiarrhythmics are composed of beta-adrenergic antagonists, or beta-adrenergic blockers. Beta-adrendergic blockers used as antiarrhythmics include: Acebutolol (not used very often) Esmolol Propranolol Pharmacokinetics Acebutolol and propranolol are absorbed almost entirely from the GI tract after an oral dose. Esmolol, which can be given only by I.V., is immediately available throughout the body. Acebutolol and esmolol have low lipid solubility. That means that they can’t penetrate the highly fatty cells that act as barriers between the blood and brain, called the blood-brain barrier. Propranolol has high lipid solubility and readily crosses the blood-brain barrier. Propranolol undergoes significant first-pass effect, leaving only a small portion of these drugs available to reach circulation and be distributed to the body. Esmolol is metabolized exclusively by red blood cells (RBCs), with only 1% excreted in urine. Propranolol’s metabolites are excreted in urine. Pharmacodynamics Class II antiarrhythmics block beta-adrenergic receptor sites in the conduction system of the heart. As a result, the ability of the SA node to fire spontaneously (automaticity) is slowed. The ability of the AV node and other cells to receive and conduct an electrical impulse to nearby cells (conductivity) is also reduced. Class II antiarrhythmics also reduce the strength of the heart’s contractions. When the heart beats less forcefully, it doesn’t require as much oxygen to do its work. Pharmacotherapeutics Class II antiarrhythmics slow ventricular rates in patients with atrial flutter, atrial fibrillation, and paroxysmal atrial tachycardia. Drug interactions Class II antiarrhythmics can cause a variety of drug interactions: Administering these drugs with phenothiazines and other antihypertensives increases the antihypertensive effect. When given with nonsteroidal anti-inflammatory agents, fluid and water retention may occur, decreasing the antihypertensive effect. The effects of sympathomimetics may be reduced when taken with class II antiarrhythmics. Beta-adrenergic blockers given with verapamil can depress the heart, causing hypotension, bradycardia, AV block, and asystole. Beta-adrenergic blockers reduce the effects of sulfonylureas. The risk of digoxin toxicity increases when digoxin is taken with esmolol. Class III antiarrhythmics Class III antiarrhythmics are used to treat ventricular arrhythmias. The drugs in this class are: Amiodarone Dofetilide Ibutilide, Sotalol. Sotalol is a nonselective beta-adrenergic blocker (class II drug) that also has class III properties. Nonselective means that the drug doesn’t have a specific affinity for a receptor. Although sotalol is a class II drug, its class III antiarrhythmic effects are more predominant, especially at higher doses. Therefore, it’s usually listed as a class III antiarrhythmic. Pharmacokinetics The absorption of these antiarrhythmics varies widely After oral administration, amiodarone is absorbed slowly at widely varying rates. The drug is distributed extensively and accumulates in many sites, especially in organs with a rich blood supply and fatty tissue. It’s highly protein-bound in plasma, mainly to albumin. Dofetilide is very well absorbed from the GI tract, with almost 100% overall absorption. Of that, about 70% is bound to plasma proteins. Ibutilide, which is administered only by I.V., has an absorption of 100%. Sotalol’s absorption is slow and varies between 60% and 100%, with minimal protein- binding. Pharmacodynamics Although the exact mechanism of action isn’t known, class III antiarrhythmics are thought to suppress arrhythmias by converting a unidirectional block to a bidirectional block. Class III antiarrhythmics have little or no effect on depolarization. Rather, these drugs slow repolarization, prolonging the refractory period and duration of the action potential. Pharmacotherapeutics Class III antiarrhythmics are used for life-threatening arrhythmias. Amiodarone is the first-line drug of choice for ventricular tachycardia and ventricular fibrillation. Drug interactions Amiodarone increases phenytoin, procainamide, and quinidine levels. Amiodarone also increases the risk of digoxin toxicity. Ibutilide shouldn’t be administered within 4 hours of class I or other class III antiarrhythmics because it increases the potential for a prolonged refractory period. Dofetilide shouldn’t be administered with cimetidine, ketoconazole, megestrol, prochlorperazine, sulfamethoxazole, trimethoprim, or verapamil because of their potential to induce lifethreatening arrhythmias. Sotalol shouldn’t be administered with dolasetron or droperidol because of the increased risk of life-threatening arrhythmias. Concomitant use of amiodarone and fluoroquinolones, macrolide antibiotics, and azole antifungals may cause prolongation of the QTc interval, leading to cardiac arrhythmias, including torsades de pointes. Pressure plunge Severe hypotension may develop from too-rapid I.V. administration of amiodarone. Class IV antiarrhythmics Class IV antiarrhythmics are composed of calcium channel blockers. The calcium channel blockers verapamil and diltiazem are used to treat supraventricular arrhythmias with a rapid ventricular response (rapid heart rate in which the rhythm originates above the ventricles). For a thorough discussion of calcium channel blockers and how they work, see “Calcium channel blockers” Adenosine Adenosine is an injectable antiarrhythmic indicated for acute treatment of PSVT. Pharmacokinetics After I.V. administration, adenosine is probably distributed rapidly throughout the body. It’s metabolized inside RBCs as well as in vascular endothelial cells. Pharmacodynamics Adenosine depresses the pacemaker activity of the SA node, reducing the heart rate and the ability of the AV node to conduct impulses from the atria to the ventricles. Pharmacotherapeutics Adenosine is especially effective against reentry tachycardias (when an impulse depolarizes an area of heart muscle, then returns and repolarizes it) that involve the AV node. Drug interactions Methylxanthines antagonize the effects of adenosine, so larger doses of adenosine may be necessary. Dipyridamole and carbamazepine potentiate the effects of adenosine, so smaller doses of adenosine may be necessary. When adenosine is administered with carbamazepine, there’s an increased risk of heart block. Antianginal drugs Although angina’s cardinal symptom is chest pain, the drugs used to treat angina aren’t typically analgesics. Instead, antianginal drugs treat angina by reducing myocardial oxygen demand (reducing the amount of oxygen the heart needs to do its work), by increasing the supply of oxygen to the heart, or both. The three classes of antianginal drugs discussed in this section include: Nitrates (for treating acute angina) Beta-adrenergic blockers (for long-term prevention of angina) Nitrates Nitrates are the drugs of choice for relieving acute angina. Nitrates commonly prescribed to treat angina include: Amyl nitrite Isosorbide dinitrate Isosorbide mononitrate Nitroglycerin Pharmacokinetics Nitrates can be administered in a variety of ways. Nitrates given sublingually (under the tongue), buccally (in the pocket of the cheek), as chewable tablets, as lingual aerosols (sprayed onto or under the tongue), or by inhalation (amyl nitrite) are absorbed almost completely because the mucous membranes of the mouth have a rich blood supply. Swallowed nitrate capsules are absorbed through the mucous membranes of the GI tract, and only about one-half of the dose enters circulation. Transdermal nitrates (a patch or ointment placed on the skin) are absorbed slowly and in varying amounts, depending on the quantity of drug applied, the location of its application, the surface area of skin used, and circulation to the skin. I.V. nitroglycerin, which doesn’t need to be absorbed, goes directly into circulation. Pharmacodynamics Nitrates cause the smooth muscle of the veins and, to a lesser extent, the arteries to relax and dilate. This is what happens: When the veins dilate, less blood returns to the heart. This, in turn, reduces the amount of blood in the ventricles at the end of diastole, when the ventricles are full. (The volume of blood in the ventricles just before contraction is called preload.) By reducing preload, nitrates reduce ventricular size and ventricular wall tension (the left ventricle doesn’t have to stretch a much to pump blood). This, in turn, reduces th