OPT112_Midterm 1 Study Guide FA24.pdf
Document Details
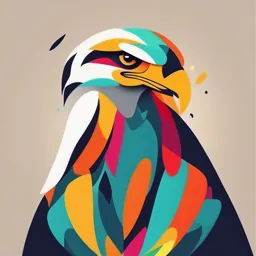
Uploaded by ConciliatoryPine3311
2024
Tags
Full Transcript
OPT112 Anatomy, Histology & Physiology I Study Guide Midterm I Examination Monday, October 7, 2024 (9:00 – 10:00 AM) Disclaimer: This is a general and not exhaustive outline of the topics you sh...
OPT112 Anatomy, Histology & Physiology I Study Guide Midterm I Examination Monday, October 7, 2024 (9:00 – 10:00 AM) Disclaimer: This is a general and not exhaustive outline of the topics you should be familiar with for the first midterm. I strongly recommend that you refer to the lecture slides and assigned reading. Questions from my lectures will consist mainly of multiple-choice questions, although a few ordering and/or matching sections may also be included. There may be items that include an image you will need to view in order to answer. For midterms, expect approximately 40-45 items. Course Introduction/Basic Anatomical Terminology Body Planes, Positions and True Anatomical Position: Cardinal body planes - Identify the following body planes from an image or a description: o Transverse or cross-section (superior/inferior sections) o Sagittal (medial/lateral sections) o Midsagittal or medial (left/right sections) o Coronal (anterior/posterior or ventral/dorsal sections) o Oblique Anatomical planes and directions: 1 Identify the following anatomical terms based on description: o Fossa o Foramen o Fissure o Fenestration o Ramus o Process True Anatomical Position: o Body is erect o Head facing directly forward o Arms hanging down and lateral to trunk/torso with palms of hands facing forward o Legs slightly apart with feet/toes facing directly forward with feet flat on the ground 2 Body cavities and serous membranes: o Body cavities ▪ Dorsal (contain CNS = brain and spinal cord) ▪ Ventral Thoracic (lungs, heart) Abdominal (gastrointestinal [GI] organs, kidneys, spleen, adrenal glands Pelvic (bladder/urethra, terminal portions of GI tract, reproductive organs) o Serous membranes line body cavities and organs (viscera) ▪ Parietal layer is outer layer of membrane lining interior wall of a body cavity. ▪ Visceral layer is inner layer of membrane lining the external surface of organ(s). ▪ Between parietal and visceral layers is a thin space (serous cavity) containing a very small amount of fluid that acts as a surfactant to reduce friction between the two layers when they slide against each other. ▪ These serous membranes and their accompanying cavities are located around the lungs, heart, and many organs and structures that comprise the GI system. 3 Cell Biology & Plasma Membrane Basic definitions: Cell biology/cell cytology: study of cellular structure Cell physiology: study of cellular function Imaging techniques: Scanning electron microscopy (SEM) offers 3-D views that allow for study of surface features Transmission electron microscope (TEM) offers 2-D views thorough thin-cut sections and is optimal for visualizing internal structures of a cell or within an organelle Maximum resolution of a light microscope is 0.2-0.5μm, would you be able to visualize a: ▪ mitochondrion? ▪ nucleus (typical 5-10um diameter in typical human cell)? ▪ ribosome? ▪ typical protein? ▪ structures that form the cytoplasmic skeleton, such as microfilaments/intermediate filaments/microtubules (0.01-0.03um in typical human cells)? Organelle Overview Nonmembranous organelles lack membranes and are in direct contact with the cytoplasm ▪ Examples: ribosomes, centrosome/centrioles, cilia/flagella, cytoskeleton, nucleolus Membranous organelles are surrounded by either one or two lipid bilayer membranes ▪ Examples with two lipid bilayer membranes: Nucleus (nuclear envelope) and mitochondria ▪ Examples with one lipid bilayer membrane: Lysosomes, peroxisomes, endoplasmic reticulum, Golgi body and the plasma membrane 4 Cellular organelles Nucleus ▪ Largest organelle within a cell with a round/ovoid body located near the cell center ▪ The double membrane nuclear envelope contains nuclear pores that allow molecules to pass between the nucleus and the cytoplasm ▪ No membrane-bound organelles are present in the nucleus ▪ Contains coiled strands of DNA (chromatin) which condense to form chromosomes at cell division ▪ Functions: Stores and transmits genetic information in the form of DNA; genetic information sent to cytoplasm where ribosomes read the codon sequence of mRNA to code for a series of amino acids which will eventually become a protein. Nucleolus ▪ Spherical, densely stained filamentous structure within the nucleus ▪ Function: Site of ribosomal RNA (rRNA) synthesis and protein components of ribosomal subunits, which then move to the cytoplasm through nuclear pores Ribosomes ▪ Packages of ribosomal RNA (rRNA) and protein ▪ Types: − Free ribosomes throughout cytosol that synthesize proteins used inside the cell from mature mRNA − Membrane-bound ribosomes are attached to the rough endoplasmic reticulum (RER) and synthesize protein needed for export or for use within the cell membrane (i.e., integral, peripheral proteins) − Mitochondrial proteins are produced by special ribosomes within the mitochondria Endoplasmic reticulum ▪ Rough ER (RER) is continuous with nuclear envelope with attached ribosomes that synthesize, process and packages proteins for export from the cell or to the cell membrane (NOTE: free ribosomes synthesize proteins for local use within the cell.) ▪ Smooth ER (SER) has no attached ribosomes, but does synthesize phospholipids (think plasma membrane), steroids (again, think plasma membrane and cholesterol that stabilizes membrane fluidity) and fats; also functions in detoxifying harmful substances like alcohol 5 Golgi apparatus/body ▪ Series of cup-shaped, closely apposed, flattened, membranous sacs with associated vesicles typically situated near the nucleus/rough ER − Cis-face: Side of protein ‘entry’ − Cisternae: Site of protein modification − Trans-face: Side of protein ‘exit’ (proteins packaged into secretory vesicles at this point) ▪ Function: Concentrates, modifies, and sorts proteins arriving from the rough ER prior to their distribution via vesicles that will remain in the cell (lysosome) or to the outside of the cell via exocytosis Lysosomes (“Membranous vesicles”) ▪ Formed in Golgi complex and filled with digestive enzymes (the ‘stomach’ of the cell) ▪ Pumps in H+ ions until internal pH reaches 5.0 (acidic environment) ▪ Functions: Digest foreign substances (i.e., bacteria) or digest/recycle components of the cell’s organelles (autophagy) or in cases of cell destruction (autolysis) Peroxisomes ▪ Vesicles smaller than lysosomes and contain enzymes (catalases) that oxidize toxic organic material (alcohol, aldehydes, hydrogen peroxide = H2O2) Mitochondria ▪ Double membrane organelle with a central cavity matrix and an inner membrane (crista) 6 ▪ Mitochondrial DNA almost exclusively from mother as sperm mitochondria broken off during fertilization and, therefore, fail to enter the egg cell. ▪ Function as ATP generators and can self-replicate if significant ATP is required by the cell to properly perform its functions Cytosol ▪ Takes up 55% of cell volume and contains 75-90% water with lesser concentrations of large organic molecules (proteins, carbohydrates, lipids), dissolved small organic molecules (simple sugars), ions, and aggregates of lipids and glycogen granules (inclusions) ▪ Site of many important chemical reactions, such as production of ATP, synthesis of building blocks for organelles Cytoskeleton ▪ Network of protein filaments throughout the cytosol that are continuously reorganized that provides cell support and gives the cell its characteristic shape ▪ Filaments types − Microfilaments (actin): Locomotion & division − Intermediate filaments (multiple proteins): Anchor organelles − Microtubules (tubulin): Flagella, cilia & centrosomes Centrosome ▪ Located near nucleus with two (2) centrioles oriented perpendicular ▪ Nine (9) clusters of 3 (triplet) microtubules (9+0 array) ▪ Play vital role in formation of cilia & flagella basal bodies as well as development of the mitotic spindle during cell replication 7 Cilia & Flagella ▪ General structure: o Shaft contains pairs (doublets) of microtubules along with a central pair (9+2 array) o Basal body derived from centriole, so microtubule arrangement is the same (i.e., triplet microtubules in a 9+0 array) ▪ Differences: Cilia are short and multiple projecting from the cell membrane (respiratory cilia) and typically have coordinated movements (some cilia are non-motile) while flagella are long, single (i.e., sperm) and exhibit wavelike movements Cell Membrane ▪ Flexible but sturdy barrier that surround cytoplasm of a cell ▪ Contents: − Phospholipids comprise 75% of cell membrane lipids in a bilayer configuration (phosphate heads facing intracellular/extracellular compartments and fatty acid tails facing each other) ▫ Polar parts (heads that containing phosphate and glycerol) are hydrophilic and face a watery environment (cytoplasm or external environment) ▫ Nonpolar parts (fatty acid tails) are hydrophobic and line up next to each other within the membrane ▫ Since phospholipids are comprised of a hydrophilic and hydrophobic component, they are amphipathic − Cholesterol & Glycolipids ▫ Cholesterol (20% lipid composition) and glycolipids (5%) scattered among a double row of phospholipid molecules ▫ Hydrophobic cholesterol contains stiff steroid rings and hide within the hydrophobic cell membrane (allows for cell rigidity) around the fatty acid tails of phospholipids − Proteins ▫ Integral proteins extend into or completely across cell membrane while peripheral proteins (i.e., G proteins = guanine nucleotide binding protein) lie proximal to the inside of the cell membrane within the cell’s cytoplasm (in other words, these latter proteins are intracellular) ▫ Those integral proteins extending completely across cell membrane are transmembrane proteins and function as channels (i.e., aquaporins, ion leak 8 channels), receptors (i.e., ionotropic vs. metabotropic), or as enzymes (i.e., adenylyl cyclase or phospholipase C that are crucial in the activation of second messengers intracellularly ▫ All integral proteins are amphipathic with hydrophobic (nonpolar) portions hiding among the phospholipid fatty acid tails ▫ Glycoproteins have the sugar portion facing the extracellular fluid to form a glycocalyx (along with glycolipids also in the cell membrane) which protects the cell from being digested or, in the case of the corneal surface as discussed in class, also allows for tear film adherence ▪ Membrane Fluidity & Permeability − Membranes are fluid structures (oil layer) and are self-sealing when punctured − Membrane molecules can rotate & move freely but need to stay in one-half of lipid bilayer as difficult for hydrophilic portion to pass though hydrophobic core of bi-lipid layer − Lipid bilayer is permeable to nonpolar (uncharged) molecules including oxygen, CO2, steroids as well as to very small amounts of small, polar (charged) molecules like water which flows through gaps that form in hydrophobic core of membrane as phospholipids move about (note: most water transport is via specialized membrane transporters called aquaporins) − Transmembrane proteins act as specific channels for the majority of small and medium- sized polar molecules − Very large molecules (macromolecules) that are unable to pass through the membrane require vesicular transport (i.e., endocytosis, exocytosis) 9 Cell Membrane Regulation and Potentials Body Compartments and Fluids Total body water mostly intracellular fluid (~2/3 volume) Extracellular fluid (plasma + interstitial) is mostly interstitial (~3/4 of extracellular volume) In order to maintain homeostasis, fluid intake (mostly obtained through eating and drinking) must roughly equal fluid output (mostly through urination and sweat) Composition of Intracellular and Extracellular fluids: Varies depending on compartment (NOTE: You do not need to know exact numbers, but should know where concentrations are higher/lower relative to intracellular vs. extracellular for Na+, Cl-, K+, Ca2+, ATP, amino acids, protein, glucose, and phosphate. − Na+ and Cl- highest in plasma and extracellular fluid (think of cell is ‘floating’ in salt water) while K+ highest in the intracellular fluid (all are important for maintaining membrane potential, although effects of Na+ and K+ dominate). − Ca2+ highest in the extracellular fluid and is vital in muscle contraction and the process involved in neurotransmitter release from the transmissive segment of a neuron. − Phosphate ions (Pi), proteins (and its precursor, amino acids) and ATP highest intracellular (both produced within cell). 10 − Glucose concentration highest outside cell as passes into the cell for metabolism via glucose (GLUT) transporters (more on this process in OPT122). Feedback loops are important in maintaining a physiological condition (i.e., body temperature), or homeostasis, within a normal range around a set point (i.e., 98.6°F/37.0°C is normal human body temperature) Positive feedback: Reinforcement of stimulus; requires major event to restore homeostasis Negative feedback: Opposite action to stimulus to restore homeostasis; most common feedback loop of the two Plasma membrane electrochemical gradients and the membrane potential Membranes can maintain difference in concentration of a substance inside versus outside of the membrane (chemical gradient) − Greater O2, Na+, and Cl- concentration outside cell (again, think of the cell floating in a salty ocean) and greater CO2 and K+ concentration inside cell Membranes can also maintain a difference in charged ions between inside & outside of membrane (electrical gradient) − Negative charge inside cell membrane and positive charge outside in resting state (resting membrane potential) due to leakier K+ (non-gated) ion channels vs. other ion leak channels within the cell membrane − The overall charge of a cell’s membrane in all human cells varies at rest depending on the cell type (chemical and electrical gradient of all ions are in balance), although all are overall negatively charged (this is the resting membrane potential, or RMP) ▪ Equilibrium potentials ◦ When an ion is in equilibrium (its diffusion and electrical forces are equal and, therefore, there is no net movement of an ion into or out of a cell) ◦ The Nernst equation can be used to determine, for any ion, the equilibrium potential that is necessary to balance an ionic concentration across the plasma membrane so the net movement of that ion is zero (i.e., the flow of the ion down its concentration gradient is opposed by the increasing change in charge of the compartment the ion is flowing into to the point the opposing charge is equal to the ions concentration gradient, so additional movement of the ion occurs) ◦ While the Nernst equation assumes the membrane is perfectly permeable to a specific ion, the Goldman-Hodgkin-Katz (GHK) equation also incorporates the ion concentration into which provides for a more accurate calculation of the RMP for a particular cell type 11 Membrane transport mechanisms overview: Passive Transport: Osmosis ▪ Osmosis is the net movement of water through a selectively permeable membrane − Although osmolarity refers to the concentration of solute particles, it also determines the water concentration in the solution − The higher the osmolarity (total concentration of solute[s] dissolved in a solvent [i.e., water] = solution), the lower the water concentration (i.e., fewer water molecules compared to solute particles) and vice versa − Osmotic pressure is the required minimum pressure applied to a solution in order to stop osmosis (oncotic pressure is a form of osmotic pressure we will cover later in fluid exchange within a capillary bed) 12 ▪ Non-penetrating solutes are those that cannot cross the membrane from the extracellular environment unassisted − Isotonic solutions (no change in cell shape) − Hypertonic solutions (crenation of cell = cell shrinkage) − Hypotonic solutions (cell expansion; lysis/hemolysis if expansion is great enough to rupture the cell membrane) Passive Transport: Simple diffusion ▪ Water and solutes can disperse in the presence or absence of a membrane down their concentration gradient ▪ When molecules are evenly distributed, equilibrium has been reached ▪ Influences on diffusion rate: − the greater the difference in concentration, the faster the rate of diffusion − the higher the temperature, the faster the rate of diffusion − the larger the size of the diffusing substance, the slower the rate of diffusion − an increase in surface area, increases the rate of diffusion − increasing diffusion distance, slows rate of diffusion ▪ The rates at which molecules diffuse across membranes (simple diffusion), as measured by their permeability coefficients, are a thousand to a million times slower than the diffusion rates of the same molecules through a water layer of equal thickness ▪ The major factor limiting diffusion across a membrane is the hydrophobic interior of its lipid bilayer; however, O2, CO2, fatty acids, and steroid hormones are all nonpolar molecules that diffuse rapidly through membranes (simple diffusion) − Remember that lipophilic (lipid-loving) substances move through the phospholipid bilayer with relative ease (particularly those that are nonpolar lipophilic) − Polar molecules do not diffuse readily through the plasma membrane and the vast majority require a protein transporter ▪ Diffusion types: ‒ Simple diffusion involves no transport mechanism and small, uncharged, nonpolar molecules can readily pass through the phospholipid bilayers of the plasma membrane ‒ Facilitated diffusion requires membrane protein transporter via ion channels or a carrier protein 13 Ions such as Na+, K+, Cl– and Ca2+ all use specific integral (transmembrane) protein channels (i.e., ion channels) to diffuse into and out of cells down their respective concentration gradient Specificity is determined by pore size of the channel, charge, and binding sites Two types: ▪ Non-gated (leak) channels are always open (ions and water) ▪ Gated channels open and close in response to a stimulus result in neuron excitability: ‒ Voltage-gated channels will open in response to change in voltage and are associated with action potentials ‒ Chemical-gated/Ligand-gated channels open & close in response to a specific chemical stimulus (i.e., hormone, neurotransmitter, ion) and are associated with graded potentials (EPSPs and IPSPs) ‒ Mechanically-gated channels will open with mechanical stimulation and associated with action potentials Sodium channels ▪ Mediate fast depolarization (rapid influx of Na+ down its electrochemical gradient) and conduct electrical impulses throughout excitatory cells like neurons and muscle ▪ Sodium channel blockers slow the rate and amplitude of the initial, rapid depolarization of an action potential reduces cell excitability and conduction velocity ‒ Examples: Class I antiarrhythmic medications, anesthetics, TTX/tetrodotoxin (irreversibly binds to binding site on Na+ channel, blocking ion influx into cell preventing depolarization; highly neurotoxic; found in several species of bacteria and in amphibians like certain newts, but most known is pufferfish, a delicacy specifically in Japan) Potassium channels ▪ K+ outflow mediates hyperpolarization and at a slower rate than depolarization ▪ K+ channel blockers: TEA (tetraethylammonium) previously used to treat heart arrythmias and HTN (now only used in research given the many potential severe side effects) and potassium chloride (KCl) used in lethal execution procedure (blocks repolarization that would allow for the initiation of another action potential) 14 Passive Transport & Mediated-Transport Systems: Facilitated diffusion ▪ Many molecules (i.e., glucose) are either too large and/or charged to get into the cell without help ▪ The protein transporters (also called carriers) are specific to a molecule and brings them into and out of cells by conformation changes ▪ Some do not require ATP (facilitated diffusion) while other require ATP (active transport) Active Transport: Mediated-Transport Systems ▪ Active transport utilizes ATP or an ion (typically Na+) to drive substances against their concentration gradients (i.e., from low to high concentration) − These transporters are often called “pumps” and may utilize two types of energy sources: ▫ The direct use of ATP in primary active transport where ATP is hydrolyzed to ADP to produce the energy needed to drive the pump ▪ The Na+/K+ ATPase pump is found in every cell and helps establish and maintain the membrane potential of the cell; pumps ions against their concentration gradient with 3 Na+ ions out with 1 ATP used and 2 K+ ions in with an additional ATP used ▪ Others: H+ ATPase pump (proton pump); Ca2+ ATPase pump ▫ The use of an electrochemical gradient across a membrane via a transporter ion (again, typically Na+) to drive the process occurs with secondary active transport ▪ Cotransporters (symporters) move two molecules in the same direction across the cell membrane (one down its gradient and the other against its concentration gradient); this process is symport secondary active transport ▪ Countertransporters (antiporters) move two molecules in opposite directions across the cell membrane (one down its gradient and the other against its concentration gradient); this process is antiport secondary active transport 15 Active processes: Vesicular transport Endocytosis involves bringing something into cell by phagocytosis (‘cell eating’) or pinocytosis (‘cell drinking’); some phagocytosis processes involve something (i.e., bacterium) binding to a receptor on the cell membrane (receptor-mediated endocytosis) Exocytosis is opposite of endocytosis where contents released from cell across the cell membrane − Vesicles form inside cell then fuse to cell membrane where contents are released into the extracellular environment − The cell membrane of the vesicle replaces the cell membrane that is lost during endocytosis as, in this event, the cell membrane envelopes phagocytized material as it enters the cell (phagosome) Graded and Action Potentials (Neuron) Resting Membrane Potential Muscle fibers and neurons are examples of cells with excitable cell plasma membranes In a typical neuron, the resting membrane potential is -70mV (cell is polarized and ready to fire an impulse) ▪ The Goldman-Hodgkin-Katz (GHZ) equation, an extension of the Nernst equation, determines the resting membrane potential considering 1) the ionic concentration for each contributing ion as determined by the Nernst equation as well as 2) the membrane permeability for each contributing ion (NOTE: you will not be expected to perform any calculations regarding these two equations) ▪ Remember, extracellular fluid rich in Na+ and Cl- ions and intracellular fluid rich in K+, negatively-charged proteins and amino acids, etc. 16 ▪ Resting membrane potentials are different for other cell types (cardiac muscle: about -90mV; epithelial cells: about -50mV) Graded potentials Small deviations from resting potential of -70mV and occur in the receptive segment of the neuron (i.e., dendrites and soma) Signal initiation from binding a ligand to an ion channel (i.e., neurotransmitter, hormone, etc.) Amplitude of response dependent on strength of stimulus (hence, a ‘graded’ response) Signal propagation limited to a short distance (i.e., synapse to neuron cell body) Individual graded potentials are summated at the initial segment of the neuron; if the membrane potential reaches threshold, then an action potential will be initiated in the initial segment of a neuron The initiated action potential will propagate down the conductive segment towards the transmissive segment Propagation of an Action Potential in the Conductive Segment of a Neuron ▪ The Na+/K+ ATPase pump helps to stabilize an action potential to return the membrane to its resting potential; the stage is now set for the next action potential ▪ An action potential spreads over the surface of the axon membrane (axolemma) ▪ Immediately prior to depolarization, a stimulus from multiple graded potentials in the soma changes membrane charge at the axon hillock (initial segment) from -70mV up to the membrane threshold of -55mV ▪ As the membrane reaches this threshold, voltage-gated Na+ channels rapidly open and Na+ streams into the cell as the membrane potential changes rapidly to around +30mV ▪ At full depolarization (around +30mV), the voltage-gated Na+ channels are inactivated (they remain open but no longer allow for passage of Na+ ions due to a ‘gate’ covering the opening of the channel), the voltage-gated K+ channels begin to slowly open and the process of repolarization begins (i.e., the membrane potential moves back towards a negative charge at a slower rate than what was experienced with depolarization) ▪ The outflow of K+ from the cell returns the membrane potential back to -70mV (repolarization: reversal in membrane charge from +30mV to -70mV); sometimes too much K+ leaves the cell and the membrane potential can become hyperpolarized (about -90mV) before returning to -70mV with the help of ion leak channels and the Na+/K+ ATPase pump. 17 ▪ Both the voltage-gated Na+ and K+ channels close when cell is polarized (i.e., back to the RMP of the neuron, or -70mV) and the cell is ready for the next action potential ▪ For a propagating impulse, local changes in the charge of the axonal surface and axoplasm between nodes of Ranvier (i.e., the myelinated portion between the nodes) move the depolarization/repolarization process down to the next node and so on (saltatory conduction) all the way to the neuron’s synapse with a downstream neuron or effector; if there are no nodes (i.e., such as in an unmyelinated axon), then continuous conduction occurs 18 ▪ Refractory Periods of an Action Potential − The refractory period is the time during an action potential when a neuron generally cannot generate another action potential − Absolute refractory period: Not even the strongest stimulus will generate another action potential even when the membrane potential is above the threshold − Relative refractive period: A strong-enough stimulus to reach threshold may generate another action potential even if the cell has yet to return to the polarized state (the RMP) ▪ Nerve Conduction/Propagation ▪ Continuous conduction: Step-by-step depolarization of each portion along the entire length of the axolemma of unmyelinated axons ▪ Saltatory conduction: Depolarization occurs only at the nodes of Ranvier of myelinated axons where there is a high density of voltage-gated ion channels − Current carried by ions flows through extracellular fluid from node-to-node down the axon − The propagation speed of a nerve impulse not related to stimulus strength 19 Fiber types and Speed of Impulse Propagation A fibers are largest and provide fastest impulse propagation (5-20μm & 130m/sec); are myelinated (somatic sensory + motor fibers) B fibers are medium-sized (2-3μm & 15m/sec); somewhat myelinated (autonomic) C fibers are smallest with slowest impulse propagation (.5-1.5μm & 2m/sec); unmyelinated (somatic sensory and autonomic fibers) In other words, myelinated AND large-diameter axons allow for fastest conduction of an impulse and unmyelinated AND small-diameter axons allow for slowest conduction Synaptic Transmission Synapses are locations where an axon of an upstream (presynaptic) neuron ‘communicates’ with the dendrite(s) of a downstream (postsynaptic) neuron or effector (i.e., muscle, gland, etc.) Types of synapses: Mechanical synapses: Channels are pulled open by physical movement (cochlear hair cells, muscle spindles) Electrical synapses: Currents (ions) pass through gap junctions rapidly between bound presynaptic and postsynaptic neurons (cardiac and smooth muscle, retina) Chemical (ligand) synapses: Most common type of synapse with the presynaptic neuron containing synaptic vesicles (containing neurotransmitter), mitochondria, and the active zone while the postsynaptic neuron is separated by the synaptic cleft and contains receptors that bind to a specific neurotransmitter Neurotransmission Neurotransmitters are synthesized mainly in the axon terminal and then stored in protein- coated (clathrin) membranous vesicles ▪ These vesicles are formed by budding and pinching of the cell membrane during endocytosis 20 ▪ Vesicles are then filled with neurotransmitter (manufactured within the neuron or recycled from the synaptic cleft, such as choline from the enzymatic degradation of acetylcholine by acetylcholinesterase) ▪ Vesicles are loosely docked to active zones within the presynaptic cell by SNARE proteins As an action potential reaches the presynaptic axon terminal, Na+ and K+ channels are activated; in addition, voltage-gated Ca2+ channels open, allowing for influx of these ions down their concentration gradient into the presynaptic axon terminal Ca2+ influx allows for binding of these ions to synaptotagmin which ‘interacts’ with the SNARE proteins embedded in the cell membrane allowing for vesicle to the presynaptic axon terminal membrane and release of the neurotransmitter into the synaptic cleft Neurotransmitters cross synaptic cleft and binds with postsynaptic receptors (some postsynaptic receptors have ‘extensions’ on their surface – dendritic spines – which increase the surface area for additional receptors) Chemical-gated channels open on the postsynaptic neuron/effector initiating a graded potential (depending on which channels open, the graded potential will be an EPSP or IPSP) ▪ A quantum of neurotransmitter released from a vesicle is required to initiate even the smallest potential possible on the postsynaptic cell (minimum end plate potential = MEPP) ▪ Multiple quanta neurotransmitter will then generate multiple MEPPs which may trigger a graded potential (NOTE: in skeletal muscles, these are referred to as EPPs although the term can loosely be applied to all excitatory cells as the concept is the same) ▪ The summation of multiple excitatory graded potentials will generate an action potential in the initial segment if the membrane potential has at least reached the threshold (-55mV in a neuron) Removal of neurotransmitter can occur several ways to stop the signal: 1. Actively transported back into presynaptic axon terminal (‘reuptake’) – some drugs like Prozac inhibit the reuptake of the neurotransmitter serotonin (hence, this medication is classified as a ‘serotonin reuptake inhibitor’); 2. Transported to nearby glial cells for degradation; 3. Diffuses down its concentration gradient away from the receptor site; 4. Enzymatically degraded (i.e., acetylcholinesterase degrades neurotransmitter acetylcholine). 21 Postsynaptic transmission ▪ Graded potentials generate in the receptive segment are either excitatory or inhibitory o Graded potentials do not require a threshold stimulus unlike action potentials o Remember, action potentials propagate in the conductive segment (axon) ▪ Excitatory Postsynaptic Potentials (EPSPs) vs. Inhibitory Postsynaptic Potentials (IPSPs) o EPSPs result in greater influx of Na+ into the cell vs. outflow of K+ resulting in the membrane potential becoming more positive/less negative than the resting membrane potential (depolarization) o IPSPs result in greater outflow of K+ and influx of Cl- vs. Na+ influx resulting in a net hyperpolarization of the cell (membrane potential becomes more negative than resting membrane potential) 22 ▪ Spatial vs. temporal summation o Spatial summation: Numerous EPSPs, IPSPs (or both) are initiated by different presynaptic neurons around the same time o Temporal summation: Numerous EPSPs or IPSPs (not both) are initiated by the same presynaptic neuron − In one example, a presynaptic neuron will initiate EPSPs on the postsynaptic neuron while another presynaptic neuron will initiate IPSPs on the same postsynaptic neuron − In a second example, a presynaptic neuron will initiate EPSPs on the postsynaptic neuron while another presynaptic neuron will also initiate EPSPs on the same postsynaptic neuron − In a third example, a presynaptic neuron will initiate IPSPs on the postsynaptic neuron while another presynaptic neuron will also initiate IPSPs on the same postsynaptic neuron o Both spatial and temporal summation signals integrate allowing the membrane potential of the neuron to possibly reach threshold; if threshold is reached, then an action potential will be initiated 23 Neural pathways can involve different types of circuits ranging from simple to much more complex ones: Diverging Converging Reverberating Parallel-after- discharge Neural diseases/drugs of interest… Myasthenia gravis is an autoimmune disease where the body produces antibodies against the acetylcholine (Ach) receptor ▪ Damage to the Ach receptor affects neurotransmission to the muscles and the patient can present clinically with symptoms of diplopia (double vision secondary to reduced ability for extraocular muscles to move) and/or ptosis (droopy eyelid due to weakened levator muscle in eyelid) ▪ Treatment is with an inhibitor of acetylcholinesterase (neostigmine) that allows more time for Ach to bind to still- functioning Ach postsynaptic receptors 24 Multiple sclerosis (MS) is another autoimmune disease affects neurotransmission by producing antibodies against the myelin sheath of myelinated axons ▪ Plaques form in white matter of CNS ▪ Optic nerve can be affected as it’s myelinated – patient can present with color vision defects, blurred vision, peripheral vision defects Botox injections block synaptic release of excitatory neurotransmitter from presynaptic axon terminal which ultimately relaxes muscle (i.e., removes wrinkles, relieves eyelid spasms) Neurotransmitter introduction; neuromodulation; G protein/second messenger signal transduction systems What are neurotransmitters? Synthesized by neurons and typically stored in synaptic vesicles in presynaptic axon terminal (knobs) Are released from vesicles that are fused to the membrane of the synaptic knob secondary to the actions of Ca2+, synaptotagmin, and SNAREs They bind to the receptor on the postsynaptic neuron (or effector) as a first messenger They trigger a physiologic response downstream by initiating a graded excitatory or inhibitory postsynaptic potential (EPSPs and IPSPs, respectively) ▪ Those neurotransmitters that trigger EPSPs, are excitatory ▪ Those neurotransmitters that trigger IPSPs, are inhibitory Neuromodulators are substances (may be a neurotransmitter) that can act as… agonists by mimicking the action of a neurotransmitter antagonists by blocking the action of a neurotransmitter facilitators by enhancing the effect of a neurotransmitter inhibitors by reducing the effect of a neurotransmitter Synaptic receptors Ligands (i.e., neurotransmitters, drugs) that bind to these receptors and activate it are agonists while those that inhibit are antagonists The postsynaptic receptors… ▪ Ionotropic receptors (see A below): Ligand binds to an ion-channel receptor, rapidly opening the channel and allowing for the influx of that ion through the channel into – or out of – the postsynaptic cell (and, therefore, alters the membrane potential) ▪ These are quick-acting ▪ Response quickly tapers off ▪ Metabotropic receptors (see B below): Water-soluble ligands binds to a receptor which triggers a G-protein - second messenger mechanism which, in turn, activates the opening of ion channels of another integral protein within the cell membrane allowing for an influx of ions into – or out of – the postsynaptic cell (and, therefore, alters the membrane potential) ▪ These are slower-acting vs. ionotropic ▪ Response lasts longer than seen with ionotropic 25 G proteins and second messengers G-proteins serve as a sort of ‘switch’ to couple a receptor to an ion channel in the cell membrane General steps: Ligand binds to the transmembrane receptor (these are G protein-coupled receptors or GPCRs) and results in a change its conformation (i.e., shape) Following receptor conformation, the GDP molecule bound to the G protein is replaced with GTP, hereby activating the G protein The activated G protein then binds to an enzyme that is embedded in the cell membrane, thereby activating/inhibiting this enzyme (i.e., adenylyl cyclase, phospholipase C as illustrated in Figs. 17.7 and 17.8 in the McKinley text; both shown below) ▪ Once the enzyme/ion channel has been activated, GTP on the G protein alpha subunit is cleaved into GDP and phosphate; the G protein is now inactivated A specific second messenger is activated by the enzyme when bound by the G-protein: ▪ Cyclic AMP (cAMP) ▪ Cyclic GMP (cGMP) ▪ Diacylglycerol (DAG) ▪ Inositol triphosphate (IP3) ▪ Ca2+ ▪ Arachidonic acid 26 The second messenger then activates a particular protein kinase which will stimulate (or inhibit) signal pathways within that cell ▪ Referring to the images immediately above in this example: ‒ Inactive G protein within the postsynaptic cell’s cytoplasm bound to guanosine diphosphate (GDP) ‒ After ligand (i.e., the first messenger) binds to receptor, the G protein binds to the receptor protein as well but on the intracellular side ‒ Immediately GDP is bumped off the G protein and replaced with guanosine triphosphate (GTP) which now activates the G protein 27 ‒ The G protein then binds to another transmembrane protein which is an enzyme (in this example, adenylate cyclase or also known as adenylyl cyclase) on the intracellular side which catalyzes ATP into a second messenger (in this case, its cyclic AMP or cAMP) ‒ The second messenger then activates another enzyme, a kinase, which will trigger modifications of the numerous activities taking place within a cell ‒ The binding of one ligand to a receptor will significantly amplify the number of final products (i.e., image to the right), so these ligands are very potent in a response ▪ In another example utilizing the images above: ‒ Phospholipase C acts as the transmembrane protein (enzyme) that a G protein will bind to triggering the formation of not one, but two second messengers, diacylglycerol (DAG) and inositol triphosphate (IP3) ‒ DAG activates a protein kinase while IP3 activates a third messenger, Ca2+, which can activate additional protein kinases 28