Muscle Physiology 3 PDF
Document Details
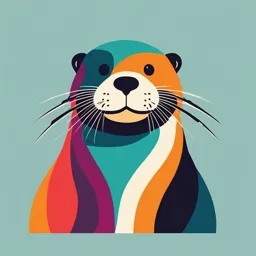
Uploaded by NimbleVoice
St. George's University
Dr Rade Durbaba
Tags
Summary
This document details lecture notes on muscle physiology, specifically focusing on the musculoskeletal system. It covers cardiac and smooth muscle, including their structure, function, and regulation, provided by Dr. Rade Durbaba at St. George's University (undergraduate level).
Full Transcript
Basic Principles of Medicine 1 Module: Musculoskeletal System Lecture 25: Muscle Physiology 3 Dr Rade Durbaba Copyright All year 1 course material, whether in print or online, is protected by copyright. The work, or parts of it, may not be copied, distributed or published in any form, printed, elect...
Basic Principles of Medicine 1 Module: Musculoskeletal System Lecture 25: Muscle Physiology 3 Dr Rade Durbaba Copyright All year 1 course material, whether in print or online, is protected by copyright. The work, or parts of it, may not be copied, distributed or published in any form, printed, electronic or otherwise. As an exception, students enrolled in year 1 of St. George’s University School of Medicine and their faculty are permitted to make electronic or print copies of all downloadable files for personal and classroom use only, provided that no alterations to the documents are made and that the copyright statement is maintained in all copies. View only files, such as lecture recordings, are explicitly excluded from download and creating copies of these recordings by students and other users are strictly illegal. The author of this document has made the best effort to observe current copyright law and the copyright policy of St George's University. Users of this document identifying potential violations of these regulations are asked to bring their concern to the attention of the author. Recommended Reading Medical Physiology: Principles for Clinical Medicine, 6e. Rodney A. Rhoades, David R. Bell. Chapter 13: Mechanics of cardiac pump. Read under headings, “ Introduction” and “Excitationcontraction coupling in cardiac muscle”. Medical Physiology: Principles for Clinical Medicine, 6e. Rodney A. Rhoades, David R. Bell. Chapter 8: Skeletal and smooth muscle. Read under headings, “Smooth Muscle” and “Activation-contraction coupling in smooth muscle”. Slides with blue background are supplemental information and will not be explained again during the lecture time. Please read the slides for clarification as they are equally important to know. Learning objectives Cardiac Muscle SOM.MK.II.BPM1.2.MSK.2.PHYS.0067. Contrast the duration of the action potential and the refractory period in a cardiac muscle, a skeletal muscle, and a nerve. Sketch the temporal relationship between an action potential in a cardiac muscle cell and the resulting contraction (twitch) of that cell. On the basis of that graph, explain why cardiac muscle cannot remain in a state of sustained (tetanic) contraction. SOM.MK.II.BPM1.2.MSK.2.PHYS.0068. List the steps in excitation-contraction coupling in cardiac muscle from the initiation of an action potential to the relaxation of that cell. SOM.MK.II.BPM1.2.MSK.2.PHYS.0069. Provide specific details about the special role of Ca2+ in the control of contraction and relaxation of cardiac muscle. SOM.MK.II.BPM1.2.MSK.2.PHYS.0070. Compare cardiac and skeletal muscle with respect to: cell size, electrical connections between cells, and arrangement of myofilaments. Based on ion permeability and electrical resistance describe role of gap junctions in creating a functional syncytium. SOM.MK.II.BPM1.2.MSK.2.PHYS.0071. Identify the role of extracellular calcium in cardiac muscle contraction. Identify other sources of calcium that mediate excitationcontraction coupling and describe how intracellular calcium concentration modulates the strength of cardiac muscle contraction (Inotropism). Learning objectives Smooth Muscle SOM.MK.II.BPM1.2.MSK.2.PHYS.0072. Compare the similarities and differences in the mechanisms of actomyosin regulation in the smooth muscle vs striated muscle (cardiac and skeletal muscles). SOM.MK.II.BPM1.2.MSK.2.PHYS.0073. Explain the concept of latch mechanism in the smooth muscle, and how these cells can develop and maintain force with a much lower rate of ATP hydrolysis than skeletal muscle. SOM.MK.II.BPM1.2.MSK.2.PHYS.0074. List the intracellular pathways that control contraction and relaxation in smooth muscle and explain the differences between electromechanical coupling and pharmacomechanical coupling. SOM.MK.II.BPM1.2.MSK.2.PHYS.0075. Compare the characteristics of multi-unit and unitary smooth muscle types. SOM.MK.II.BPM1.2.MSK.2.PHYS.0076. Explain the myofilament calcium sensitization and desensitization mechanisms. Cardiac muscle Myocardium forms the walls of atria and ventricles that pump blood throughout the vascular system SOM.MK.II.BPM1.2.MSK.2.PHYS.0067 Histology: A Text and Atlas: With Correlated Cell and Molecular Biology, 8e, 2020 Cardiac Myocyte Structure Linked to T-tubule to form diad SOM.MK.II.BPM1.2.MSK.2.PHYS.0070 http://www.cleveland.com/healthfit/index.ssf/2012/08/ghost_heart_a_framework_for_gr.html The T tubules of cardiac muscle are much larger than the T tubules of skeletal muscle and carry an investment of external lamina material into the cell. They also differ in that they are located at the level of the Z line. The portion of the sarcoplasmic reticulum adjacent to the T tubule is not in the form of an expanded cisterna but rather is organized as a “diad” anastomosing network. This diagram illustrates the organization of the sarcoplasmic reticulum and its relationship to the myofibrils in skeletal muscle. In skeletal muscle fibers, two transverse (T) tubules supply a sarcomere. Each T tubule is located at an A–I band junction and is formed as an invagination of the sarcolemma It is associated with two terminal cisternae of the sarcoplasmic reticulum that surrounds each myofibril, one cisterna on either side of the T tubule. The triple structure as seen in cross section, where the two terminal cisternae flank a transverse tubule at the A–I band junction, is called a triad. Depolarization of the T-tubule membrane initiates the release of calcium ions from the sarcoplasmic reticulum and eventually triggers muscle contraction. Types of Cardiac Muscle Action Potentials Two broad types of cardiac muscle action potentials: Fast response type: Ventricular muscle (A), Atrial muscle (C) & Purkinje fibers. Slow response type: Sinoatrial (SA) node (B) and Atrioventricular (AV) node Numbers in graph indicate phases of the AP AP generated at pacemaker sites (Automaticity). No absolute requirement for nerve stimulation for depolarization to occur. AP spreads throughout whole muscle via gap junctions to contract collectively (Functional Syncytium). Thus, no Spatial Summation. SOM.MK.II.BPM1.2.MSK.2.PHYS.0067 SOM.MK.II.BPM1.2.MSK.2.PHYS.0070 Cardiac action potential RMP close to K+ equilibrium potential depolarization: Na+ channels open/inactivate plateau phase: Ca++ channels open, K+ channels close repolarization: Ca++ channels close, K+ channels open refractory period ~250 milliseconds value of plateau & refractory period: heart must relax before contracting again SOM.MK.II.BPM1.2.MSK.2.PHYS.0067 Duration of the Action Potential: SOM.MK.II.BPM1.2.MSK.2.PHYS.0067 Cardiac Muscle – Importance of long cardiac AP duration Skeletal Cardiac Cardiac AP has a long Absolute Refractory Period (ARP). Protects heart from too frequent excitation as No Temporal Summation is possible in myocardium, and therefore No Tetanic Contraction Helps heart to work as an Effective Pump SOM.MK.II.BPM1.2.MSK.2.PHYS.0067 Cardiac E-C coupling – Contraction & Relaxation 1. DHP/ 1 2. 2 3. 4. 3 When Action Potential passes over the sarcolemma and down the T-tubules This trigger the entry of calcium into the cell via the DHP/L-type calcium channels in the T-tubules An increase in intracellular Ca2+concentration induces calcium release via the Ryanodine-sensitive calcium release channels on the junctional SR. When the intracellular free Ca2+ concentration is high (>1.0 μM), cross-bridges form between the thick and thin filaments found within the muscle. The amount of Ca2+ that enters the cell during a single action potential is quite small compared with that released from the SR. 4 Contraction Not all SR Ca2+ is released for contraction (normal conditions Ca2+ 0.5-2 µM vs 10 µM skeletal). SOM.MK.II.BPM1.2.MSK.2.PHYS.0068 SOM.MK.II.BPM1.2.MSK.2.PHYS.0069 SOM.MK.II.BPM1.2.MSK.2.PHYS.0071 Cardiac E-C coupling – Contraction & Relaxation Ca2+ unbinds to troponin About 80% of the calcium is actively taken back up into the SR by the action of SERCA pumps About 20% of the calcium is extruded from the cell into the extracellular fluid either via -Na+–Ca2+ exchanger -Ca2+-ATPase pumps Contraction Relaxation SOM.MK.II.BPM1.2.MSK.2.PHYS.0068 SOM.MK.II.BPM1.2.MSK.2.PHYS.0069 Striated Muscle: SKELETAL vs CARDIAC Skeletal muscle cells Cardiac muscle cells Nucleus of the cell Multiple, peripheral nuclei Central, one or two nuclei SHAPE of the cell Non-branched cylindric Branched cylindric SIZE of the cell Diameter: 25 μm Length: almost entire length of muscle (several cm) Diameter: 25 μm Length: 100 μm Electrical properties Cells electrically isolated from each other, each cell receive innervation, cannot contract without nerve stimulation All the cells form electrical syncytium (GAP junctions), AUTOMATICITY Action potential has short duration (like nerve cells): 5 msec Action potential has a long duration: 200-300 msec Sarcoplasmic reticulum (SR) Extracellular Ca++ and SR Source of activator Ca++ “calcium-induced calcium release” Arrangements of myofilaments SOM.MK.II.BPM1.2.MSK.2.PHYS.0070 Actin and myosin filaments arranged in sarcomeres (contractile units) Role of calcium in heart muscle performance Movement of ECF Ca2+ is essential for contraction Amount of Ca2+ released from the sarcoplasmic reticulum (SR) depends on the amount of Ca2+ moving into the cell during the action potential Amount of calcium released also depends on the stored Ca2+ in the SR. Force of contraction correlates directly with the intracellular Ca2+ concentration Greater the intracellular Ca2+ concentration → Greater the force of contraction SOM.MK.II.BPM1.2.MSK.2.PHYS.0071 Inotropism (contractility) and calcium Contractility, or inotropism, is the intrinsic ability of myocardial cells to develop force at a given muscle cell length. Increase in contractility: Positive inotropic effect; Decrease in contractility: Negative inotropic effect. All positive inotropic agents elevate intracellular Calcium. SOM.MK.II.BPM1.2.MSK.2.PHYS.0071 Inotropism - Factors/agents Positive inotropism Sympathetic nerve stimulation Catecholamines (Epi, NE & Dopamine) Exercise Beta receptor agonists Cardiac glycoside (Digoxin) PDE-3 inhibitor (Milrinone) Negative inotropism Sympathetic inhibition Heart failure with reduced ejection fraction Myocardial loss Beta receptor antagonists Calcium channel blockers e.g. Sympathomimetic drug such as Dobutamine which is a Beta1 receptor agonist, is useful in shock due to myocardial infarction SOM.MK.II.BPM1.2.MSK.2.PHYS.0071 Increasing Ca2+ entry occurs via increased cAMP levels in myocardium PDE-3 inhibitor (Milrinone): prevents degradation of cAMP Catecholamines, Sympathetic stimulation, Beta-receptor agonists, Exercise Beta1 SOM.MK.II.BPM1.2.MSK.2.PHYS.0071 Clinical Correlate - Digoxin (Positive Inotrope) Inhibition of the cardiac Na+/K+-ATPase thatresults in an increase in intracellular Na+ Na+/Ca2+ channel exchanger will work less efficiently since there is no Na+ gradient. Na+ Ca2+ Thus intracellular Ca2+ will accumulate. Increased storage of Ca2+ within SR Subsequent contraction, increased Ca2+ release from the sarcoplasmic reticulum, increasemyosinactin interaction and increase in contractile force. b receptor Note increased intracellular Ca2+ will prolong AP and hence reduce heart rate. SOM.MK.II.BPM1.2.MSK.2.PHYS.0071 INOTROPISM Anrep effect – autoregulatory mechanism for increasing cardiac myocyte contractility with increased afterload. It allows the heart to adjust for an increased end-systolic volume (ESV) and the decreased stroke volume (SV) that occurs due to increased aortic blood pressure. Without it, aortic blood pressure increases would led to decreased SV that would compromise peripheral and visceral tissue circulation. Cardiac myocyte stretch activates tension dependent Na+/H+ exchangers leading to ↑ Na+ ions within the myocyte. ↑ Na+ reduces the Na+ gradient for sodium-calcium exchangers and stops them from working effectively. Ca2+ ions ↑ inside and as a result are pumped by sarco/endoplasmic reticulum Ca2+-ATPase (SERCA) pumps into SR. With next action potential, greater amount of Ca2+ is released from SR, leading to more crossbridges being formed and hence an increase in the force of contraction of the cardiac muscle to try and increase SV and cardiac output to maintain tissue perfusion. Bowditch effect – autoregulatory mechanism for increasing cardiac myocyte contractility with increased heart rate. It is thought an inability of Na+/K+ ATPase to keep up with increased heart rate leads to ↑ Na+ ions within the myocyte. ↑ Na+ reduces the Na+ gradient for sodium-calcium exchangers and stops them from working effectively. Ca2+ ions ↑ inside and as a result are pumped by sarco/endoplasmic reticulum Ca2+-ATPase (SERCA) pumps into SR. With next action potential, greater amount of Ca2+ is released from SR, leading to more crossbridges being formed and hence an increase in the force of contraction of the cardiac muscle. SOM.MK.II.BPM1.2.MSK.2.PHYS.0071 Smooth Muscle Smooth Myocyte Comparison to Skeletal Muscle Each fiber is much smaller than in skeletal muscle Diameter 2 - 10 m Length 100 - 500 m Single nucleus No myofibrils Actin and myosin present - NO regular arrangement No Z-line - Actin attached to dense bodies (-actinin) SR present – NO terminal cisternae No T-tubule – small invaginations called caveoli Medical Physiology: Principles for Clinical Medicine, 3rd Ed., Rhoades & Bell Copyright © 2008, Lippincott, Williams & Wilkins. caveoli SR SOM.MK.II.BPM1.2.MSK.2.PHYS.0072 Smooth Myocyte Found in the hollow or visceral organs. Usually found in two different layers: Circular – to constrict or dilate Longitudinal – to stretch or shorten SOM.MK.II.BPM1.2.MSK.2.PHYS.0072 Smooth muscle location Gastrointestinal tract: Propulsion of the food bolus Cardiovascular: Regulation of blood flow and pressure via vascular resistance Renal: Regulation of urine flow Genital: Contractions during pregnancy, propulsion of sperm Respiratory tract: Regulation of bronchiole diameter Integument: Raises hair with erector pili muscle Sensory: Dilation and constriction of the pupil as well as changing lens shape SOM.MK.II.BPM1.2.MSK.2.PHYS.0072 Striated Smooth Thin filament Actin & Tropomyosin Actin, Tropomyosin & Troponin Thick filament Bipolar arrangement Heads absent from central region Medical Physiology: Principles for Clinical Medicine, 3rd Ed., Rhoades & Bell Copyright © 2008, Lippincott, Williams & Wilkins. Side-polar arrangement Heads along whole length of filament SOM.MK.II.BPM1.2.MSK.2.PHYS.0072 E-C coupling - Ca2+ release from SR Voltage gated Ca2+ channel Action potential mediated membrane depolarization activates these channels – electromechanical coupling Ligand-gated channels and ligand-receptor 2nd messenger systems Chemicals such as hormones and neurotransmitters bind to ligand gated channel and allows Ca2+ influx and initiates contraction when a specific agonist binds to a specific receptor. Sarcoplasmic reticulum Agonist receptor linked to 2nd messenger G-protein system to modulate release of Ca2+ from the sarcoplasmicreticulum. Contraction of smooth muscle via ligand gated channel and/or ligandreceptor 2nd messenger systems is called pharmacochemical Myoplasm coupling Stretch activated Ca2+ channel Neither voltage nor ligand mediated These channel play a role in the regulation of vascular smooth muscle contraction. Modified from Medical Physiology: Principles for Clinical Medicine, 4th Ed., Rhoades & Bell Copyright © 2013, Lippincott, Williams & Wilkins. SOM.MK.II.BPM1.2.MSK.2.PHYS.0074 Activation of Myosin & Crossbridge Cycling in Smooth Muscle Contraction of smooth muscle is dependent upon releasing Ca2+ that is stored within the SR to increase the intracellular Ca2+ concentration. Release is either via Ca2+ induced Ca2+ release mechanism or from 2nd messenger mediated modulation of Ca2+ released from the SR. Ca2+ binds to calmodulin (CaM). The Ca2+-CaM binds to and activates myosin light chain kinase (MLCK). When activated, MLCK phosphorylates myosin and allows it to bind to actin, thus initiating cross-bridge formation and recycling. Regulation of myosin phosphorylation, and hence cross formation, is a balance between MLCK and myosin light chain phosphatase (MLCP) activity. Medical Physiology: Principles for Clinical Medicine, 3rd Ed., Rhoades & Bell Copyright © 2008, Lippincott, Williams & Wilkins. SOM.MK.II.BPM1.2.MSK.2.PHYS.0074 E-C Coupling – Muscle Relaxation A. Ca2+ influx stops with the repolarisation of the membrane and removal of 2nd messenger agonists – Ca2+ channels close. B. Ca2+ is actively pumped out from the muscle fibre either to the extracellular space or into the SR, via SERCA. C. Within the SR, Ca2+ is sequestered by Calsequestrin & Calreticulin. D. Low cytosolic Ca2+ suppresses activation of calmodulin and MLCK and allows for MLCP activity to dominate. Myosin heads dephosphorylated and inactive. E. Muscle relaxes Medical Physiology: Principles for Clinical Medicine, 4th Ed., Rhoades & Bell Copyright © 2013 Lippincott, Williams & Wilkins. Sarcoplasmic reticulum Myoplasm SOM.MK.II.BPM1.2.MSK.2.PHYS.0074 Nett result Muscle Contraction Striated vs Smooth Muscle Striated muscle Smooth muscle Actomyosin regulation Thin filament: Troponin C/ Tropomyosin Thick filament: phosphorylation of the regulatory light chain of Myosin II (myosin-linked regulation) –Ca++/CaM mediated Contractile units Sarcomere No sarcomere Thin filaments Actin, Tropomyosin Troponin Thick filaments Thick to thin filament ratio (reports vary according to species and muscle) Caldesmon, calponin Myosin II Bipolar arrangement Side-polar arrangement Higher amount of Myosin II Lower amount of Myosin II 1:2 to 1:6 1:10 to 1:17 SOM.MK.II.BPM1.2.MSK.2.PHYS.0072 Smooth Muscle Tone Smooth muscle exhibiting rhythmic or intermittent activity is termed phasic smooth muscle, e.g. smooth muscles of the walls of the gastrointestinal tract and urogenital tract. Typically corresponds to the single- unit category via voltage gated calcium channel (i.e. membrane depolarization) Smooth muscle that is continuously active is termed tonic smooth muscle, e.g. vascular smooth muscle, respiratory smooth muscle and sphincters. Typically correspond to the multiunit smooth muscle via ligand gated calcium channels (hormonal, pharmacologic or metabolic factors) SOM.MK.II.BPM1.2.MSK.2.PHYS.0074 Smooth Muscle Innervation Nerve supply is autonomic - both sympathetic and parasympathetic. Postganglionic nerve fibers do not end in motor endplate but branch diffusely. The terminal axon of the postganglionic fibers have a network has a beaded appearance called Varicosities. They contain the neurotransmitter ACh/NE. SOM.MK.II.BPM1.2.MSK.2.PHYS.0075 Nerve-Muscle Arrangement Multi unit Single Unit: This represents the majority. Discrete units - similarity to skeletal muscle. Separately supplied by ANS axon One ANS axon supplies a single muscle fibre within a group. The muscle fibres associated within a group are joined together by gap junctions - FUNCTIONAL SYNCYTIUM. Allows for coordinated contractions Large blood vessels, large airways, ciliary muscle & iris Uterus, GIT SOM.MK.II.BPM1.2.MSK.2.PHYS.0075 Control of smooth muscle contraction Gq-protein-PLC-IP3 pathway Norepinephrine, Epinephrine & Dopamine (via alpha adrenoreceptors) in vascular smooth muscles Smooth muscle cell Sarcoplasmic Reticulum Ca++ IP3R + IP3 + DAG Ca++ Acetylcholine (via M2 receptors) in GIT RyR Angiotensin II (via angiotensin II receptors) in vascular smooth muscles PIP2 Receptor Phospholipase C Gq-protein Endothelin (via ETA receptors) Ligand binds to specific Receptor SOM.MK.II.BPM1.2.MSK.2.PHYS.0074 Control of smooth muscle contraction cAMP pathway Smooth muscle cell RELAXATION Enhances Ca2+ reuptake into SR AMP Inhibits MLCK & activates MLCP PDE cAMP ATP Receptor Norepinephrine, Epinephrine (via beta2 adrenoreceptor) in bronchial & uterine smooth muscles Dopamine (via D1 receptors) in renal vascular muscles Adenylyl cyclase Gs-protein Histamine (via H2 receptors) in skin blood vessels Beta-2 agonist, Albuterol inhaler is used in Asthma for bronchodilation Terbutaline, beta-2 agonist used to relax uterine smooth muscles in pregnant women with premature labor SOM.MK.II.BPM1.2.MSK.2.PHYS.0074 Control of smooth muscle contraction NO-cGMP pathway Smooth muscle cell RELAXATION Inhibits MLCK & activates MLCP Enhances Ca2+ uptake into SR + GTP Guanylate cyclase inactive + NO cGMP cGMP dependent protein kinase Guanylate cyclase active Nitric Oxide (NO) Nitrates used in angina increase NO in vascular smooth muscles improve blood flow to heart. Drugs that increase cGMP in vascular smooth muscles cause vasodilation and reduce blood pressure in severe hypertension (Examples: Hydralazine, Nitroprusside) SOM.MK.II.BPM1.2.MSK.2.PHYS.0074 Control of smooth muscle contraction Ca2+ sensitization and desensitization MLCK and MLCP activities alter calcium requirements of contraction. Increased MLCK activity and decreased MLCP activity: Can produce a stronger contraction even with a lower-than-normal calcium. This is called calcium sensitization. Decreased MLCK activity and increased MLCP activity: Can produce a weaker contraction even with a higher-than-normal calcium. This is called calcium de-sensitization. Mediated via Rho-kinase activity Myosin-P Myosin MLCP SOM.MK.II.BPM1.2.MSK.2.PHYS.0076 Latch state and smooth muscle Smooth muscles can maintain a high level of force of contraction with a very low level of calcium and ATP consumption. Cross-bridge cycling occurs at a slow rate so that each remains in the attached state for a longer time with least amount of energy and calcium usage. Latch state is NOT a rigor state Importance: This is helpful in organs where a sustained contraction for a long period is required with least energy expenditure. Example: blood vessels to maintain BP for the entire life. SOM.MK.II.BPM1.2.MSK.2.PHYS.0073 Distinguishing Characteristics of Muscle Types. Features Skeletal Muscle (Striated) Cardiac Muscle (Striated) Smooth Muscle (Nonstriated) Cells Thick, long, unbranched, cylindric Branched, cylindric Small, spindle-shaped Nuclei per cell Many; peripheral One or two; central One; central 1:2 to 1:6 1:10 to 1:17 Thick to thin filament ratio (varies according 1:2 to 1:6 to species and muscle) Sarcoplasmic reticulum and myofibrils Highly organized sarcoplasmic reticulum surrounds distinct myofibrils Less organized sarcoplasmic reticulum; irregular myofibrils Poorly organized sarcoplasmic reticulum; no myofibrils T tubules At A–I band junctions; form triads At Z lines; form dyads None Motor end-plates Present Absent Absent Motor control Voluntary Involuntary Involuntary Other Prominent fascicles, thick perimysium and epimysium Intercalated disks at cell- Abundant caveolae, cells to-cell junctions overlap Overall summary of the muscle types Tetanus Yes No Partial Rhythmicity No Yes Yes