Introduction to The Art and Science of Medicine (IASM) PDF
Document Details
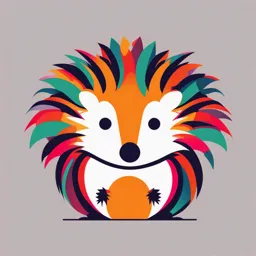
Uploaded by AmusingBambooFlute
The University of Hong Kong
Tags
Summary
This document is a content page for a book or course on the art and science of medicine. It provides a detailed outline of topics covered, ranging from molecules in medicine and precision medicine to nutrition and cellular processes.
Full Transcript
INTRODUCTION TO THE ART AND SCIENCE OF MEDICINE (IASM) Content page 1. Molecules in medicine 1.1. DNA 1.2. RNA 1.3. Amino acid 1.4. Protein 1.5. Enzyme 2. Precision medicine 2.1. Human genome 2.2. Gene sequencing 2.3. Gene editin...
INTRODUCTION TO THE ART AND SCIENCE OF MEDICINE (IASM) Content page 1. Molecules in medicine 1.1. DNA 1.2. RNA 1.3. Amino acid 1.4. Protein 1.5. Enzyme 2. Precision medicine 2.1. Human genome 2.2. Gene sequencing 2.3. Gene editing 3. Nutrition 3.1. Human nutrition 3.2. Vitamins 3.3. Minerals 4. Metabolism 4.1. Glucose metabolism 4.2. Lipid metabolism 4.3. Amino acid metabolism 4.4. Generation of ATP 4.5. Integrated metabolism 5. Cell and tissue 5.1. Structure of cell 5.2. Nucleus 5.3. Cytoskeleton 5.4. Epithelial tissue 5.5. Connective tissue 5.6. Cell proliferation 6. Signalling and movement 6.1. Cell membrane transport 6.2. Cell membrane signalling 6.3. Membrane excitability 6.4. Nervous system 6.5. Nerve tissue 6.6. Muscle tissue 7. Cellular development 7.1. Embryology 7.2. Genetic inheritance 7.3. Cell injury and death 7.4. Cell healing and repair 7.5. Neoplasia 7.6. Genetic basis of cancer 8. Microbial infection 9. Immune defence 10. Health and health system 1. Molecules in medicine 1.1. DNA 1.1.1. Structure B-DNA Most common form of DNA Right-handed double helix (anticlockwise) Ribose-phosphate backbone: homogenous, stable → good for storage 5' → 3' with antiparallel stand Each base pair has a purine and a pyrimidine 10.4 bases per rotation of 360º (bases rotating ~36º to the right of each other) A nucleotide includes pentose, base and phosphate The numbering of carbons on pentose sugar: ○ 1' – connect to base ○ 2' – determination of DNA or RNA- hydroxyl – RNA (oxy) ○ 3' – free hydroxyl group (linked to another phosphate) ○ 5' – linked phosphate group Purine (larger) : A, G Pyrimidine (smaller): T/ U (without methyl group), C Chirality ○ The chirality of nucleic acids derives from the compositional asymmetry of the sugars forming the DNA and RNA backbones: D- deoxyribose and D-ribose Major groove (long gap) ○ More accessible for transcription factors Minor groove (short gap) ○ Mutations may disrupt minor groove binding properties and hence DNA structure ○ Protein binding at minor groove will distort protein structure much more severely Diagram of B-DNA structure: Other types of DNA ○ A-DNA: similar right-handed double helix, but with a shorter, more compact helical structure whose base pairs are not perpendicular to the helix-axis as in B-DNA ○ Z-DNA: left-handed helical form of DNA in which the double helix winds to the left in a zigzag pattern Chargaff’s rule (1947): ○ A always binds to T, C always binds to G ○ %A = %T, %C = %G 1.1.2. Order of DNA structure DNA wraps around histone octamers to form a nucleosome Histone ○ Protein in chromatin ○ Histone modification (methylation/ acetylation/ phosphorylation) regulate gene expression by controlling which DNA is exposed to polymerase ○ Core histone proteins: H2A, H2B, H3 and H4 (2 copies) From DNA to nucleosome to chromatid LEVEL EQUIVALENT Chromatids (x2) 10 coils each Coil 30 rosettes Rosette 6 loops Loop ~75,000 bp 30 nm fibre / “Beads-on-a-string” form of chromatin / DNA 1.1.3. DNA expression and gene regulation Transcription factor ○ Bind to promoter areas ○ Direction methylation of DNA ○ Bind to mediator Brings together several transcription factors which directly bind to mediator protein May be an enhancer or silencer 1.1.4. Central dogma DNA is transcribed to RNA, which is translated to protein ○ Irreversible (except HIV: RNA → DNA) Colinearity of the DNA, mRNA and the amino acid sequence of polypeptide proteins ○ Coding strand (5' → 3') = mRNA stand (5' → 3') = polypeptide (amino terminus → carboxyl terminus) 1.1.5. Transcription Transcription process: A. Initiation Transcription unit: [promoter] + [transcribed region] ○ Promoter on template strand recognized by transcription factors (protein) → guide RNA polymerase to attach to gene TATA-box (within the promoter region) stabilise RNA polymerase ○ RNA polymerase unwinds the DNA double helix (Mg- dependent) B. Elongation RNA polymerase moves along the DNA template strand in 3' to 5' direction → synthesis RNA in 5' to 3' direction DNA rewinds into a double helix C. Termination Elongation continues until the RNA polymerase encounters a stop codon Transcription stops, RNA polymerase releases the DNA template strand mRNA precursor (or pre-mRNA) is formed Pre-translational mRNA process: D. Splicing Introns are cleaved by spliceosomes ○ Introns: intervening sequences ○ Extrons: expressing sequences (actually translated to protein) ○ Snurps catalyse the formation of spliceosome snRNA (short nuclear RNA) - join with protein→ snRNP (snurps: to cleave introns, join exons) E. 5' capping Initiate translation Prevent degradation by enzymes F. 3' poly(A) tail [AAAAA] poly(A) polymerase recognise polyadenylation signals [AAUAA] → formation of polyadenylated mRNA precursor (poly(A) tail) Initiate translation Prevent degradation by enzymes Mature mRNA is formed 1.2. RNA 1.2.1. Variety and function Types and functions of RNA ○ Messenger RNA (mRNA): Transcribed from DNA ○ Transfer RNA (tRNA): Adapter to link amino acids to codons ○ Ribosomal RNA (rRNA): Structure of ribosome, central catalytic site ○ Single guide RNA (sgRNA) ○ Small nuclear RNA (snRNA) RNA VS DNA RNA DNA Structure Transient, Resilient, Additional H-bond Strong sugar-phosphate → more variety of structure backbone → diverse function Hydroxyl group at 2' Hydrogen at 2' Uridine (U) instead of thymidine (T) 1.2.2. Translation A site tRNA brings amino acid to mRNA at ribosome P site Peptide linkage is formed between adjacent amino acids E site A polypeptide is thus formed according to genetic coding in DNA Summary of transcription and translation: 1.3. Proteins 1.3.1. Properties of amino acid Amino acids are the building blocks of proteins Containing both amine and carboxyl functional groups Proteins are macromolecules made up of L-α amino acids ○ chiral centre (enantiomeric) ○ L form: with H towards you, then spell CORN in clockwise direction Under neutral aqueous conditions, both carboxyl and amino groups ionise [NH2 → NH3+, COOH → COO-] Non-polar Glycine Smallest Only H in the R group Not chiral Alanine Methyl group Proline Only one that bond twice onto the backbone Valine, Leucine, Isoleucine Very hydrophobic Methionine Sulphur containing (in between carbon) Not involved in sulphur-sulphur bridge Polar Serine, threonine, cysteine, asparagine, glutamine Aromatic Phenylalanine, tyrosine, Largest amino acid R group tryptophan Useful to determine protein concentrations Amino acid sequence named from N-terminus to C-terminus Peptide bond forms between amino acids Protein (100+ amino acids) 1.3.2. Function of protein To catalyse reactions, e.g. pepsin (active conformation) For nucleic acid interaction, e.g. CRISPR Cas9 As receptors on membranes, e.g. serotonin receptors To provide structure to cells and tissues, e.g. collagen To aid the immune system, e.g. antibodies Example: insulin Insulin binds to receptor The glucose transporter is inserted into the membrane to allow glucose shuttling There are more glucose transporters embedded in the membrane [Downstream: glucose transporters are transported up into membrane] Change in conformation of intracellular domain of insulin receptor Increase uptake of glucose into liver cells/ muscle cells Increased glycogen synthesis (glycogenesis) as a response to increase in insulin Protein subunits demonstrate a large extent of complementarity ○ Contains two polypeptide chains with 𝛼-helical structure ○ Linked by sulphur-sulphur bridge between cysteines Cysteine + cysteine -oxidation (lose of electrons & H+)→ cystine ○ Extracellular proteins are frequently stabilised by covalent cross- linkages including disulphide bonds between Cys (extracellular conditions are usually oxidative → removal of H from SH group in Cys) 1.3.3. Protein bonds and structure Primary ○ Sequence of amino acids from N-terminal to C-terminal ○ Including post-translational modification (phosphorylation/ glycosylation) ○ Importance: specific order of amino acids dictates how the protein will fold and ultimately determines its function Secondary ○ Local conformation of backbone due to interaction between backbone atoms (eg α-helix and β-sheets) stabilised by H-bond ○ Αlpha-helix Side chain sticks out Difference in characteristics depends on side chain ○ Beta-sheet Pleated structure (zigzag like) Parallel/ antiparallel ○ Importance: provide stability and contribute to 3D structure of protein Tertiary ○ 3D structure due to interaction between amino acid side chains ○ By electrostatic attraction/ Van der Waals’ forces ○ Importance: determines active sites and binding sites that are essential for biological activities Quaternary ○ Involve more than one polypeptide chains ○ E.g. haemoglobin (alpha subunit x2, beta subunit x2) ○ Importance: interaction between subunits affect protein stability and functionality 1.3.4. Classification A. Fibrous proteins Have long, extended repetitive sequences that enable structural roles Fibrous proteins are insoluble and abundant in tissue Example 1 – Collagen: The most abundant protein in the human body – in skin, teeth, cartilage, bone Higher tensile strength than steel – useful in structural roles Structure: Left-handed alpha helix 3 alpha helices for right-handed superhelix (strong fibre) Glycine-proline-hydroxyproline Larger collagen structure: Triple helices join to form larger supramolecular structure (fibrils) Striation across fibril ○ Evenly spaced interactions between collagen triple helix molecules (heads come together in repetitive fashion to form striated pattern) Requires hydroxyproline for joining of polypeptides Hydroxyproline: Modified proline, hydroxyl group added Pentameric proline side chains are outside Related diseases: Osteogenesis imperfecta (OI) ○ Mutation in type I collagen ○ Increased bone fractures & collagen defects Scurvy ○ Lack of vitamin C (sodium ascorbate) Collagen lose of structural integrity Gum diseases, loosening of teeth ○ More sodium ascorbate is kept in reduced state by vitamin C → less Fe2+ → prolyl 4-hydroxylase (enzyme that convert proline to hydroxyproline) X act → X proper structure of collagen → gum disease Example 2 – Keratin: Structure: 2 alpha helices Coiled coil structure ○ Coiled coil structure → protofilament → protofibril → intermediate filaments Maintained by disulphide bridges in cysteine ○ To stabilise keratin Hair curling: Chemical (reducing agent) reduce cystine disulphide bridge to thiols Hair curls with heat Oxidised to stay in curled state (with less disulphide bridges) More on keratin: The tougher the keratin, the more cysteines involved in disulphide bonds Some infectious fungi feed on keratin B. Globular proteins Have rounded structure with functional (catalytic) roles Aqueous in soluble environment Example – Haemoglobin and Myoglobin Oxygen binding proteins found in red blood cells (erythrocytes) RBCs have lost their nucleus, mitochondria and other organelles → carry more haemoglobin (98% of protein in blood) with ~120 day half-life Haemoglobin: Tetrameric (α2β2) with 4 haem groups which carries oxygen Iron ion held in place by the haem and histidine O2 binding site is opposite to iron Iron and heme groups are close to surface of protein → accessible to O2 Haem: Can carry oxygen in haemoglobin and myoglobin Consists of a porphyrin ring & nitrogen in the centre ○ Nitrogens interact with central Fe2+ of haemoglobin/ myoglobin Histidine in backbone of myoglobin (and haemoglobin) ○ To stabilises oxygen at that position 4 haems 1 haem Transport oxygen in blood Facilitate oxygen diffusion in muscle Positive cooperativity of haemoglobin and myoglobin: More oxygen → strong binding to haemoglobin ○ Driven by structural change of subunit → second ligand bind stronger than the first Structural explanation of cooperativity: T state (Tense state): low affinity of O2, bind weakly (without any oxygen) R state (Relax state): high affinity for oxygen, bind stronger (after one or two O2 binds) R has higher O affinity than T 2 O2 binding triggers a T→R conformational change (by breaking of ion pairs between α1- β2 interface) High affinity state: At low pO2, O2 is still almost 100% in the haemoglobin (oxygen remains bound to haemoglobin and is not released) Low affinity state: Only half the carrying capacity of O2 is used by haemoglobin in the lungs Sigmoid curve: Allows effective transition from low to high affinity states Positive cooperativity enables saturation of haemoglobin oxygen in lungs, but also release to myoglobin in tissue x-axis: pO2, the partial pressure of oxygen in the surrounding atmosphere y-axis: 𝜃, the proportion of sites which are filled (with oxygen) Carbon monoxide poisoning CO disrupts haemoglobin cooperativity, locking haemoglobin into the high- affinity R state ○ CO has similar size and shape as O2 CO fit to the same binding site (O2 binds at an angle but not for CO) CO binds >20k times better than O because CO has a filled lone electron pair that can be donated to vacant Fe2+ CO poisoning is more serious than anaemia: Anaemic patients manage with half complement of haemoglobin (only have 50% capacity of haemoglobin but can still function reasonably but not for CO) 50% COHb: coma ○ CO blocks haemoglobin into high- affinity state Haemoglobin cannot release O2 to myoglobin in the tissues due to the tightly bound CO → loss of cooperativity 1.3.5. Protein folding Proteins fold into functional state Stepwise process (progressive stabilisation of intermediates) Defined by amino acid sequence entirely Some proteins require chaperones to help folding Chaperones: Large protein complexes Uses energy (ATP) to fold proteins from its unfolded states ○ E.g. GroEL (important in stress response & diseases mechanism) Proteins can fold into different states which can both be stable ○ e.g. Lymphotactin – signalling molecule chemokine Chemokine structure – signalling Glycosaminoglycan-binding structure – functional Related amyloid diseases: Prion diseases ○ Improperly folded proteins ○ Normal: PrPc – mainly an 𝛼-helix structure ○ Diseased: PrPSc – some beta strands Act to nucleate more of the PrPc to be converted into PrPSc (autocatalytic) PrPSc can act as sites of nucleation → prions are ‘infectious’ PrPSc form long strands → plaques and fibres in brain Fibrous protein aggregates in the brain – amyloid fibres Alzheimer Diseases ○ Amyloid-beta peptides self-associate into fibrils → amyloid plaque ○ Progression: conversion of native protein to more beta sheet type structure → develop longer filaments (toxic to the brain) Diabetes mellitus type 2 ○ Accumulation of islet amyloid polypeptide (IAPP) – amylin, which misfolds and aggregates in the pancreas Atherosclerosis (amyloids from APOA1) Huntington disease (amyloids from Huntingtin proteins with CAG repeats) Systemic amyloidosis (light chain antibody amyloids systemically) 1.4. Enzyme Protein catalyst Speed up biochemical reactions without being changed themselves Speed up reaction rate by lowering activation energy Specific in action ○ Able to select specific compound from a group of similar compounds & convert into product ○ E.g. glucokinase can only transfer a phosphate group to glucose from ATP Active site ○ A cleft or crevice that transform substrate to products Substrate-binding site ○ Orientates the substrate in the correct position for catalysis by the enzyme 1.4.1. Classification 1.4.2. Models of enzyme A. Lock & key model Amino acid residues of the substrate binding site are arranged in a complementary 3D surface that recognizes the substrate The substrate is bound via hydrophobic interactions,electrostatic interactions and hydrogen bonds B. Induced fit model Substrate binding site is not a rigid pocket Dynamic surface with flexible 3D structure As the substrate binds, the side chains of the amino acids in the active site will reposition to interact with the substrate for the reaction to occur 1.4.3. Michaelis Menten equation Describes the relationship of the enzyme rate (vi ; initial velocity) to the substrate concentration [S] Vmax = maximum velocity achieved at infinite amount of substrate Km (Michaelis constant)= concentration of substrate needed to achieve half of Vmax Km measures affinity of enzyme for a substrate ○ Low Km = high affinity ○ High Km = low affinity 1.4.4. Bisubstrate enzyme reaction Enzymatic reactions involving two substrates and giving two products E + 𝐴𝑋 + 𝐵 ⇌ 𝐸 + 𝐴 + 𝐵𝑋 A. Sequential reactions (single displacement reaction) Ordered: one substrate must bind first, before the second substrate can bind and a reaction can occur Random order: no requirement of which substrate has to bind first for reaction to occur B. Double displacement reaction (ping-pong reaction) Functional group is transferred from substrate 1 to enzyme to form product 1 Substrate 2 then binds and functional group is transferred from enzyme to substrate 2 to form product 2 1.4.5. Factors affecting enzyme activity A. Substrate concentration Limiting factor: number of enzymes ○ All active sites of enzymes are occupied by substrates Level: maximum velocity of enzymes reached B. Enzyme concentration C. pH Optimum pH range: enzymes are most active Changes in pH can change the ionisation of functional groups in the active site → loss of activity/ denaturation D. Temperature 1.4.6. Regulation of enzyme reaction Purpose: reduce wastage Regulatory enzymes usually catalyses the rate-limiting step in the pathway A. Reversible (1) Competitive Competes with the substrate for binding at the substrate-binding site Structurally similar to the substrate Increase in substrate concentration to a sufficiently high level can overcome competitive inhibition (2) Non-competitive Does not compete with the substrate for the substrate-binding site Does not affect the binding of the substrate Increase in concentration of the substrate will not overcome the inhibition (3) Uncompetitive Products as reversible inhibitors: All products are reversible inhibitors of the enzymes that produce them Accumulation of product → decrease in the rate B. Conformation (1) Allosteric activation & inhibition Allosteric site: site away from the active site where compounds can bind on an enzyme ○ Distort shape of active site → inhibition ○ Change the active site to fit the substrate → activation Advantages of regulation with allosteric effectors Stronger effect on the enzyme velocity than inhibitors in the active site Effectors can be activators as they do not bind the active site Allosteric effectors can be molecules other that substrate/ product of the enzyme Rapid effect (2) Covalent modification Phosphorylation ○ Addition/ removal of phosphate group of amino acid/ residue of enzyme ○ Phosphate group causes conformational change in ionic interaction/ hydrogen bond pattern ○ E.g. Muscle Glycogen Phosphorylase (breakdown glycogen) (3) Protein-protein interaction (4) Proteolytic cleavage Some enzymes are synthesised as proenzymes and need to undergo proteolytic cleavage to become fully functional Irreversible E.g. blood clotting C. Concentration of enzyme Regulating protein synthesis/ protein degradation Slow process Mostly for long term alterations