MN Ch 12 Gas Exch Slides (12th ed.) PDF
Document Details
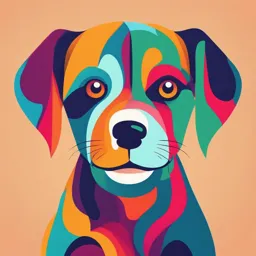
Uploaded by TranquilMagenta
2021
Michael Nazzaro
Tags
Summary
These are lecture notes on gas exchange and transport. In this document the author, Michael Nazzaro, talks about gas exchange, oxygenation, and other related aspects of respiratory physiology. The notes contain key concepts and formulas for gas exchange in the lungs.
Full Transcript
Gas Exchange & Transport RTT 100 Professor Michael Nazzaro Supplement to Text, Chapter 12 and Background for Chapter 42 You must study BOTH chapter 12 and this supplement Part 1: Gas Pressures and Gradients Revised 10-November-2021 1 Gas Exchange and Transport: Introduction Ventilation is the m...
Gas Exchange & Transport RTT 100 Professor Michael Nazzaro Supplement to Text, Chapter 12 and Background for Chapter 42 You must study BOTH chapter 12 and this supplement Part 1: Gas Pressures and Gradients Revised 10-November-2021 1 Gas Exchange and Transport: Introduction Ventilation is the mechanical process that moves ventilatory gases between the atmosphere and the alveoli. Gas exchange O2 and CO2 molecules cross the A/C membrane in the lungs, the erythrocyte membrane in the red blood cells (RBCs), and the tissue cell membranes by simple diffusion down concentration gradients. • Simple diffusion is how Gas molecules cross the A/C membrane and the cell membranes.. . Gas transport refers to the mechanisms by which the blood carries oxygen from the lungs to the tissue cells and carbon dioxide from the tissue cells to the lungs. The partial pressure of alveolar carbon dioxide (PACO2) is: ሶ Directly proportional to VCO2 (minute cellular CO2 production) and inversely proportional to Vሶ A (alveolar minute ventilation). Fig. 12-1 illustrates the diffusion gradients for the O2 and CO2 cascades. The CO2 in the PACO2 comes from the CO2 in the pulmonary capillary mixed venous blood, NOT from CO2 inhaled from the atmosphere because, as the figure shows, atmospheric PCO2 is almost zero PACO2 = ሶ VCO 2 x Vሶ K Terms in the PACO2 equations (lower left): • PACO2 is the partial pressure of CO2 alveolar gas. ሶ 2 is minute cellular production of CO2; 𝐕CO .normally about 200 ml/min. at rest. • 𝐕ሶ A is alveolar minute ventilation: (VT – VD) x RR); normally about 4.315 L/min. ሶ 2 is expressed as a flow of dry gas at • 𝐕CO standard temperature and pressure (STPD) and is reported in mL/min. • 𝐕ሶ A is expressed as a saturated gas at body temperature and ambient pressure (BTPS) and is reported in flow units of L/min. • K is a correction factor of 0.863 that reconciles the incompatibilities between STPD and BTPS. • K also allows the incompatible units of flow and pressure to be expressed as a pressure. A 200 x 0.863 = 40 mmHg 4.315 Professor Michael Nazzaro PACO2 = Reference, Note, Text pg. 105, 247, 250-252. 2 Oxygenation: The O2 Cascade Simple diffusion is the mechanism for movement of O2 molecules from the atmosphere to the mitochondria of the cells. This movement is usually called the Oxygen Cascade.1 Fick’s equation identifies the variables in diffusion across the A/C membrane (see Note!) • In the physiologic oxygen cascade, a series of pressure gradients run down from a PO2 of about 160 torr in the atmosphere to about 3-5 torr at the tissue cells. • Air contains about 21% O2 (20.95%), 79% N2 (78.08%), and about 1% other gases (0.97%). – Dalton’s Law of Partial Pressure explains gas behavior in mixtures and lets us calculate the pressure of each gas. The sea level pressure of all atmospheric gases ~ 760 torr. • When air is Inhaled into the alveoli the O2 has to share the space with three other gases: nitrogen (N2), water vapor (H2O), and carbon dioxide (CO2). 1. The normal PAN2 ≈ 573 torr. The FN2 is constant in the atmosphere, the alveoli, the blood, and the body tissues. Therefore, there is essentially no diffusion gradient for N2 to move across the A/C membrane (unless the patient is breathing 100% O2). 2. The PAH2O is also constant (47 torr at 370C), and its pressure must be deducted from PB (PB – 47 torr) when calculating alveolar PO2 (the PAO2). 3. Accounting for alveolar CO2 is a little more complicated: – CO2 is continuously diffusing into the alveoli (CO2 excretion) at a rate of 200 ml/min. • That constant diffusion from the capillaries explains the normal PACO2 of 40 torr. – O2 is continuously diffusing out of the alveoli (O2 uptake) at a rate of 250 ml/min. • The Respiratory Exchange ratio (R) is 200/250 = 0.8 (for a normal adult at rest). – R accounts for the dynamic reduction in alveolar O2 and the increase in alveolar CO2 when we use the Alveolar Gas Equation to calculate the PAO2. • One message from all this is that if the FIO2 remains constant, the PAO2 will vary INVERSELY with the PACO2 because increased CO2 will take up room in the alveolus and displace O2. 1Cascade means waterfall or a series of series of small waterfalls. In physiology a cascade is a stepwise process in which the end of one step starts the next step (like falling dominoes). Professor Michael Nazzaro Reference: Note, Text pg. 99-100, 247-49. 3 Gas Exchange: Pulmonary Gas Pressure Gradients The PO2 of air inhaled into the trachea (the PIO2) equals FIO2 x PB. (At sea level: 0.21 x 760 mmHg = 159.6 ≈ 160 torr). PA PA PaO2 PvO2 Capillary is about 1 mm long PvCO2 Pulmonary capillary PaCO2 Pressure gradients for O2 and CO2 between the alveolus, the venous and the arterial circulations. Blood entering the venous end of the pulmonary capillary is MIXED VENOUS BLOOD from the right atrium. The pressure of nitrogen (the PAN2 and PaN2) are constant. The PAO2 is less than the PBO2 because the other gases in the alveoli (N2, H2O, and CO2) take up space. Pressure (diffusion) gradients (the P1 minus P2 from Fluid Physics) provide the force for O2 and CO2 to move across the A/C membrane: – The average PIO2 is ≈ 160 torr (I means inspired atmospheric) – The average PAO2 is ≈ 100 torr – The average PvO2 is ≈ 40 torr – The average PaO2 is ≈ 100 torr The pressure gradient for O2 diffusion across the A/C membrane is about 60 torr. – The average PACO2 is ≈ 40 torr* – The average PvCO2 is ≈ 46 torr – The average PaCO2 is ≈ 40 torr The pressure gradient for CO2 diffusion across the A/C membrane is 6 torr. • The diffusion gradients are between capillary venous blood (the “Pv”) and alveolar gas (the “PA”) values. • At rest, erythrocytes transit the alveolus in about 0.75 seconds. • O2 and CO2 equilibration occurs in about 0.25 seconds. • Dalton’s Law predicts that the sum of the PaO2 and the PaCO2 will be about 140 torr. This helps us evaluate the accuracy of ABG results: – If the PaO2 + the PaCO2 is significantly >140 torr and the PaO2 is greater than about 120 torr, then the patient must be on an FIO2 >.21. Although the pressure gradient for O2 is 10 times higher than the CO2 gradient, CO2 diffuses across the A/C membrane about 20 times faster than O2 because CO2 is about 20 times more soluble in plasma than O2 (Henry’s Law). The P(A-a) O2 is NOT the diffusion gradient for oxygen. The actual gradient is the P(A-v)O2. *Atmospheric PCO2 is ≈ 0.0004 x 760 = 0.30 torr. (the text, pg. 251, states that it is 1 torr). Either number is essentially zero physiologically. The PACO2 comes from CO2 diffusing out of the pulmonary capillaries, NOT from inhaled CO2 . Reference: Note, Text pg. 99-100, 250–52 4 Effect of Alveolar Ventilation of Alveolar Gases The X axis is Vሶ A in Liters per minute and the Y axis alveolar gas pressure in mmHg. The purple curve is PAO2, and the blue curve is PACO2. The five vertical red lines show slight increases in Vሶ A between about 425 and 475 L/min. The only reference to Fig. 12-2 is in the Rule of Thumb on pg. 248. At the first glance the figure is essentially useless, but careful study reveals a great deal of information. • A Vሶ A of 4 L/min can be achieved with a comfortable VT of about 550 mL and an RR of 10/minute. o This results in a PAO2 of about 100 mmHg and a PACO2 of about 40 mmHg (orange lines). • Doubling the Vሶ A to 8 L/min cuts the PACO2 in half to 20 mmHg but only raises the PAO2 to about 120 mmHg (black lines). o The upper limit of VT for a normal healthy adult is about 600-800 mL per breath. o The upper limit for Vሶ A in a healthy adult is about 8 L/min. • To achieve a Vሶ A of 11 L/min the subject would have to take in a VT of about 750 mL and breathe about 18 times per minute: (750-150) X 18 = 10,800 mL. • The BCT would be 60/18 ̴ 3.3 seconds per breath • The I:E would have to be 1:1 so the TI and the TE would be 3.3/2 = 1.65 seconds for inhalation and 1.65 seconds for exhalation. • A well conditioned athlete might be able to do this for several minutes. “Free divers” (no SCUBA gear) can extend their time under water by hyperventilating before a dive. Hyperventilation reduces PACO2 and allows more room of O2 in the alveolus and increases hemoglobin saturation Professor Michael Nazzaro Reference: Note, Text pg. 248 (Rule of Thumb), 249 5 Cardiopulmonary Values: R/Q & V/Q • R is the Respiratory Quotient (R/Q) aka the Respiratory Exchange Ratio. ሶ 2) over minute O2 uptake (VO ሶ 2) • R is the quotient of minute CO2 production (VCO (there should be dots over the V and the Q). o R corrects for the dynamic nature of gas exchange across the A/C membrane when the Alveolar Air Equation is used to calculate the PAO2. • In normal individuals at rest: o CO2 diffuses out of the pulmonary capillaries and into the alveoli at a rate of 200 ml/min. o O2 diffuses out of the alveoli and into the pulmonary capillaries at a rate of 250 ml/min. The normal resting value is R= 200/250 = 0.8 • V/Q is the Ventilation/Perfusion ratio (there should be dots over the V and Q). • It is the ratio of minute alveolar ventilation (VA) over minute cardiac output (Q). • In normal individuals at rest: o 𝐕ሶ Ais about 4 L/min. The normal resting minute alveolar ventilation (minute volume). o 𝐐ሖ is about 5 L/min. The normal resting minute cardiac output. • The normal resting value is: 𝐕ሶ /𝐐ሶ = 4L/5L = 0.8. Other writers use 5L/6L, either way the V/Q for healthy people at rest = 0.8. Under normal, resting (steady state) conditions, both the R/Q, and the V/Q will equal 0.8 In order to maintain the R (i.e., the R/Q) and the V/Q equal at 0.8, the body must have a normal cardiopulmonary system that can change its level of alveolar minute ventilation (𝐕ሶ A) and cardiac output (Q) to keep pace with the different levels of CO2 production and O2 consumption encountered during exercise, while living at high altitude, and during illness. In cases of ventilatory failure or insufficiency, the cardiovascular system must increase its work to compensate. Professor Michael Nazzaro Reference: Note, Text, pg. 248-248 (& Mini-Clini), 250, 475- 477, 973 6 Cardiopulmonary Values: R/Q & V/Q 1 A calm, systematic appraisal of Fig. 12-5 reveals that the artist is trying to show the effect of abnormal levels of Vሶ A/Qሶ (minute alveolar ventilation, Vሶ A and pulmonary capillary perfusion, Q)ሶ on alveolar PO2, alveolar PCO2, and the Respiratory Exchange Ratio (R). V/Q values are shown above the purple curve and R values are below the curve. PAO2 is on the X axis and PACO2 is on the Y axis. Extremes of V/Q are shown at the ends of the curve: V/Q = 0 at the left and V/Q = infinity (∞) at the right end of the curve. A much more readable graph is shown in the figure at right. A normal V/Q of 1 produces a PAO2 of 100 mmHg and a PACO2 of 40 mmHg. Starting at the V/Q 1, follow the curve to the right and notice that perfusion is reduced. This makes the PAO2 higher and the PACO2 lower because there is no blood to take O2 out of the alveolus and deliver CO2 to the alveolus. Follow the curve to the left and see that ventilation is decreasing while blood is still perfusing the capillaries. Obviously, the PAO2 will get lower because there is less fresh air coming into the alveolus and the PACO2 will get higher because the blood is still delivering CO2 to the lungs. Professor Michael Nazzaro Reference: Note, Text, pg. 251 7 Pulmonary Physiology: Dr. West’s Concept (Again) • Zone 1 does not occur under normal conditions because pulmonary artery pressure is just high enough to raise blood to the top of the lung. • If arterial pressure is reduced, for example by severe blood loss (hemorrhage) or alveolar pressure is raised (positive pressure ventilation) a Zone 1 Condition will occur. – That statement means there will be increased Alveolar Dead Space. Some physiologists think that the pressure/ flow relationships in these vessels is not described by Poiseuille’s Law but resemble the p/f relationships of a waterfall: • The driving pressure for flow through the intraalveolar vessels may not always be the ∆P between capillary inflow and outflow pressure. • Flow may be controlled by the difference between the inflow pressure and the vessel’s critical closing pressure (the transmural pressure which is controlled by alveolar pressure) The relationship between alveolar pressure (distension) and pulmonary capillary perfusing pressure controls blood flow in different regions of the lung. Professor Michael Nazzaro Reference: Note, Text pg. 178, 252, 265-267. 8 Gas Exchange Abnormalities: V/Q Imbalance 1 • The formal physiological expression for the ventilation/perfusion ratio is 𝑽𝑨ሶ /𝑸ሶ𝑪Where 𝑽ሶ 𝑨 stands for minute alveolar ventilation and 𝑸ሶ 𝑪 stands for minute blood flow through the alveolar capillaries. • The actual normal V/Q is 0.8, but for convenience writers consider the normal V/Q to be one (1). – Abnormalities that lower perfusion with ventilation unchanged will increase the V/Q to a number >1. – Abnormalities that lower ventilation with perfusion unchanged will decrease the V/Q to a number <1. • The term V/Q imbalance means that the distribution of ventilation and/or perfusion in the lung is abnormal and no longer displays a 1:1 match. • Either some ventilation, some perfusion, or both will be wasted (not participate in gas exchange). Think of the black oval in the figure as a slide switch to control V/Q distribution in the model*. At the far right there is no perfusion but full ventilation. •V/Q equals a whole number divided by zero which equals infinity. •This represents pure dead space ventilation (VA = VD). •In pure dead space, alveolar gas pressures equal atmospheric. At the far left there is full perfusion but no ventilation. •V/Q equals zero divided by a whole number which equals zero. •This represents an absolute shunt . •In absolute shunt alveolar gas pressures equal capillary venous gas pressures. The far left and right positions are extremes shown for comparison. • In the normal lung the switch moves back and forth slightly (toward, not to) the extremes depending on a number of factors, including posture and exercise level. • Whatever degree of V/Q mismatching the patient with cardiopulmonary disease is experiencing will have to be identified by the clinician and treated accordingly. Reference: Note, Text, pg. 250-52 Professor Michael Nazzaro 9 Gas Exchange Abnormalities: V/Q Imbalance 2 Atmospheric air: PO2 150 mmHg PCO2 0 mmHg Absolute Shunt Unit Perfusion (Q) Alveolar air: PAO2 100 mmHg PACO2 40 mmHg Alveolar Capillary Blood: PaO2 100 mmHg PaCO2 45 mmHg Normal Unit O2 = 100 CO2 = 40 V/Q Ratio Absolute Dead Space Unit Ventilation (V) This is another way of looking at the information in the figure on Slide 9, with alveolar and capillary gas values found in the three different V/Q states. Professor Michael Nazzaro Reference: Text, pg. 250-52 10 Gas Exchange Abnormalities: V/Q Imbalance 3 V/Q imbalances that are below the extremes of pure deadspace ventilation and absolute shunt perfusion will still have an affect on oxygenation and CO2 elimination • In order to survive, patients with a low V/Q (underventilated alveoli due to lung disease) have to compensate for the low O2 by increasing VA. – They do this by increasing their rate and depth of breathing.. • These patients will have both hypoxemia and a normal or even a low PaCO2 (hypocapnia). • Patients who cannot increase VA will have both hypoxemia and hypercapnia. • Hypoxemic patients will also attempt to compensate by increasing cardiac output. • The “Guy In The Chair” is probably only able to compensate at a very low level. A relaxed, systematic survey of Fig.12-20 reveals the following information: • Arterial partial pressure of both gases is shown on the x axis. • Arterial content of both gases in shown on the y axis. • The drawing in the upper right represents alveolar/capillary units displaying both low V/Q (V/Q) and high V/Q (V/Q). • Point “a” on both curves indicates the normal arterial blood value for partial pressure and total content of both gases. − Point a on the O2 curve (midway between the extremes) shows a normal PaO2 of 90 torr and a CaO2 of 19.5 vol%. − Point a on the CO2 curve (midway between the extremes) is also normal, PaCO2 35 torr and a CaCO2 of 48 vol%. • V/Q imbalances are more likely to reduce PaO2 than they are to increase PaCO2 − This primarily results from to the great difference in solubility between O2 and CO2 and the fact that a low PaO2 stimulates increased ventilation. • In general, low V/Q units produce both increased CO2 and reduced O2 . Low V/Q indicates hypoventilation (point x). • In general, high V/Q units produce a low CO2 and a high O2 (point V/Q on both curves). Reference, Text, pg. 265-66 11 Alveolar Air (Gas) Equation: The PAO2 Calculating the partial pressure of O2 in the alveoli (PAO2) is the first step in assessing the adequacy of oxygen delivery across the A/C membrane; PAO2 is the driving pressure to get O2 across the A/C membrane into the plasma. The Clinical (simplified) Alveolar Gas Equation is used to calculate the PAO2: PAO2 = FIO2 (PB-47) - (PaCO2 1/0.8) OR (PaCO2 x 1.25) Either way gives the same answer. • We have seen that five factors determine the PAO2 1. The Fractional Inspired O2 Percentage (the ambient FIO2): - Ambient atmospheric FIO2 is always about 0.21 at any altitude from sea level to the top of Mount Everest (29,029 ft.) and out to the edge of space. 2. The Barometric Pressure (PB) which is measured with a barometer. - PB at sea level averages about 760 torr. - The PB in cities at higher altitudes will be known, for example; • The PB in Denver is assumed to average 640 torr. 3. Water vapor pressure (PH2O)) in the airways and the alveoli. - PH2O is controlled by body temperature (see vapor pressure in Ch. 6). - At body temperature, (37o C) the PAH2O is always 47 torr. 4. The patient’s PACO2 - PACO2 is difficult to measure, but the PaCO2 is essentially the same as the PACO2, so it is used.. 5. The Respiratory Exchange Ratio (aka the respiratory quotient; R or RQ) is the ratio of CO2 excretion to O2 uptake in the alveoli. – The value of the RQ can vary depending upon the type of diet and metabolic state. – RQ is different for carbohydrates, fats, and proteins (average value is around 0.82 for the human diet). – Normally R equals about 0.82 (clinically we use 0.8). – The equation uses1/R which equals 1.25. Hint: For a patient with a normal PaCO2 (40 torr), breathing room at sea level air: FIO2 x (PB - PH2O) = 150 torr, so PAO2 at sea level on room air is simply PAO2 = 150 – (PaCO2 x 1.25) = 100 torr Note: If the patient is on an FIO2 of ≥ .60 the correction factor R is dropped, and the equation becomes: PAO2 = FIO2 x (PB - 47) – PaCO2 (SEE NOTE) Reference: Note, Text pg. 105-06, 253 12 Alveolar Gas Equation: Clinical Problem • The ABG lab reports that a patient on an FIO2 of 0.21 has the following arterial blood gas values: pH 7.42 PaCO2 35 torr PaO2 150 torr. (At this point we are not evaluating acid-base status so the HCO3 is not included) • Your interpretation tells you that this ABG must be in error for at least two reasons: 1. The sum of this patient’s PaO2 and PaCO2 is 185 torr, which violates Dalton’s Law for a room air (FIO2 0.21) ABG (sum of the values in the capillary on Slide 4). – On room air the PaO2 + PaCO2 cannot be much greater than 140 torr. 2. This patient’s PaO2 is reported as 150 torr on room air. – Breathing room air (0 .21 O2) at sea level, the PAO2 would be 150 torr if there was no CO2 in the alveoli to take up space (figure on Slide 4). – This patient’s PaCO2 is 35 torr so his room air PAO2 should be 106 torr: 150 – 44 = 106 torr. (where did the 44 come from? PaCO2 x R = 35 x 1.25 = 43.75). • A patient with a normal PaCO2 (40 torr) on room air would have a PAO2 of 100 torr (150 - 50 = 100 torr) (Slide 4). – In either case, the lung is not a perfect machine, the small normal shunts and normal V/Q mismatching, prevents the PaO2 from being equal to the PAO2. • In order for the reported PaO2 of150 torr to be correct, the patient would have to be on an FIO2 of about .27, you can work it out for yourself: [0.27 x (760 - 47)] – (35 x 1.25) = [(.27 x 713)] – 44 = 193 – 44 ≈ 150 torr Labs do make mistakes and clinicians MUST be able to identify errors. Professor Michael Nazzaro Reference: Note, Text pg. 248 (Rule of Thumb) and Fig. 12-2, 373-374. 13 Oxygen Dissolved in the Plasma: PaO2 Oxygen is carried in the blood in two forms: Dissolved in the plasma and Bound to the Hb. • The PaO2 is the partial pressure of the oxygen molecules that are dissolved in the arterial blood plasma and in the intracellular fluid of the erythrocytes. o Dissolved O2 molecules move around randomly in the plasma (the Kinetic Theory of gases). o That movement generates a pressure which is measured by the O2 electrode of the ABG analyzer. o Once the O2 molecules bind to Hb they no longer exert pressure in the plasma, so bound O2 molecules are not included in the PaO2 measurement. o Just as the PAO2 is the driving pressure to get O2 across the A/C membrane so that it can dissolve in the plasma, the PaO2 is the driving pressure to get O2 across the erythrocyte plasma membrane so it can bind with the hemoglobin. • According to Henry’s Law (Text pg.103) the solubility coefficient for O2 in plasma at 37oC is 0.00003 ml of O2 per ml of blood per torr (mmHg of O2 pressure). o o o In clinical practice the deciliter (100 ml) is a more convenient unit than the ml for blood measurement, so the solubility coefficient becomes 0.00003 x 100 = 0.003 ml/dl. • Many writers now use the term volumes percent (vol%) in place of ml/dl (see Note). Texts state that the average normal PaO2 is 100 torr. • The equation for dissolved O2 is: Dissolved O2 (in ml/dl or vol%) = PaO2 x 0.003. • At a PaO2 of 100 torr, we get the textbook value for dissolved O2 as 0.3 vol% This tiny amount (< 2% of the total O2 carried) is highly significant because dissolved O2 acts as a reserve to keep the Hb saturated as the blood perfuses the systemic tissue capillaries. • Fig. 12-6, pg. 253 shows the direct, linear relationship between the PaO2 and the amount of O2 dissolved in the plasma. o A patient breathing 100% O2 (FIO2 = 1.0) with a normal PaCO2 (40 torr) will have a PaO2 ≈ 600 torr (do the alveolar air equation and then use a P(A-a)O2 of 65 torr to get the PaO2). o A patient on 100% at 3 atm (in a hyperbaric chamber) will have a PaO2 of 2240 torr and have about 6.7 vol% of dissolved O2. Professor Michael Nazzaro Reference: Note, Text pg. 253 & Fig.12-6, Ch 6 Slides. 14 Oxygenation: The P(A-a)O2 & the a/A Ratio The P(A-a)O2 is commonly referred to as the “A-a Gradient.” This is incorrect because the P(A-a)O2 does NOT represent a diffusion gradient. The correct term is the “A-a difference.” The difference between the PAO2 and the PaO2 should be very small because it indicates a gas exchange abnormality. 1. P(A-a)O2 is simply the difference between the alveolar and arterial PO2. • Since almost all the O2 in the alveoli (indicated by the PAO2) will normally diffuse across the A/C membrane, the P(A-a)O2 should be a very low number. • The text says it should be less than 5-10 torr on room air (FIO2 0.21) and ≤ 65 torr on 100% (FIO2 1.0). • Other writers say that the room air P(A-a)O2 should be “less than the patient’s age in years”. • The text gives a formula (Pg.976, Rule of Thumb): P(A-a) O2 should be less than the (patient age/4)+4. o For example, a normal 36 YO’s P(A-a O2) should be less than 13 torr: (36/4)+4 = 9+4 = 13 torr. o If her calculated PAO2 was 100 torr, her PaO2 should therefore be greater than 87 torr. • A PaO2 < 87 torr indicates a high P(A-a)O2 and a problem with O2 transfer across the A/C membrane. • The formula also states that P(A-a)O2 may normally increase by 1-3 torr for each decade >20 YO. • In addition, the P(A-a)O2 is expected to increases about 5-7 torr for every 10% increase in FIO2. o On 100% O2 the A-a O2 will be about 60-70 torr (the text says 65 torr). 2. The a/A ratio is another clinical tool used to evaluate the efficiency of O2 transfer from the alveoli into the blood across the A/C membrane. • The a/A is the ratio between arterial and alveolar oxygen pressures. • The arterial oxygen, PaO2, is divided by the alveolar oxygen PAO2: (PaO2 / PAO2). • The normal a/A ratio is ≥ 90% (≥ 0.9). o Normally, more than 90% of alveolar O2 diffuses across the A/C membrane into the blood. • A high P(A-a)O2 and a low a/A ratio are evidence of a diffusion defect. o The factors that determine gas diffusion include the distance the gas has to diffuse. (see Graham’s, Henry’s, and Fick’s Laws, Text pg. 117, 250). • Diffusion defects are conditions that have the effect of increasing the pathway distance for gas diffusion across the A/C membrane, as if the membrane had become thicker. They include conditions such as: o Pulmonary edema, hyaline membrane formation, and conditions that lead to pulmonary fibrosis. Reference: Note, Text, pg. 199Professor Michael Nazzaro 202, 248 (Mini-Clini), 1149, 1263. 15 PAO2 , P(A-a)O2, & the a/A ratio: Clinical Problem • A 20 YO female with an altered level of consciousness is brought to the ER by her boyfriend. The boyfriend reports that he came home from work and found her “asleep” on the sofa, but when he tried to awaken her, she just mumbled. He wonders if this might be caused by her taking too much pain medicine for a sprained ankle she suffered a few days ago. He doesn’t think she takes any other medications or has any drug allergies. • Physical examination reveals the following: – Respiratory rate 8/min. – Room air ABG: pH 7.21, PaCO2 75 torr, PaO2 41 torr. • The ER physician asks you to help identify the probable cause(s) of her hypoxemia, is it: 1. Hypoventilation secondary to a pain medication overdose? 2. A diffusion defect caused by vomiting and aspiration (aspiration pneumonia) which is a common occurrence in people with an altered level of consciousness? • You report to the physician: 1.She is obviously hypoventilating because her PaCO2 of 75 torr is much higher than the normal range (35-45 torr). 2. Based on calculation of her P(A-a)O2 and her a/A ratio, you conclude that she also has a diffusion defect and suggest that the physician order a chest X-Ray to confirm aspiration pneumonia. Her PAO2 = 150 - (75 x 1.25) = 150 - 94 = 56 torr (remember the Hint on Slide 9). Her a/A ratio (should be >90%) but it actually is 41/56 = 73% Her predicted P(A-a)O2 = (20/4) + 4 = 9 torr. Her actual P(A-a)O2 is 56 - 41 = 14 torr. A high P(A-a)O2 and a low a/A ratio indicate a diffusion defect, in this case it is probably an acute increase in Right to Left shunting secondary to atelectasis or consolidation. Professor Michael Nazzaro Reference: Slide 15. 16 Oxygenation: Erythrocytes (RBCs) • The primary function of the erythrocytes (red blood cells, RBCs) is to carry the hemoglobin (Hb) which carries >98% of the O2 transported by the blood. • Erythrocytes are soft, flexible, biconcave disks about 8 μm in diameter by about 2 μm thick. – Their shape maximizes the surface area for gas exchange and aids in deformability to allow the RBCs to fit through even the smallest capillaries. • RBCs are about 66% water and 33% Hb by volume • They don’t have a nucleus (they can’t divide) and they don’t have mitochondria (they don’t use O2), but they do have all the enzymes needed for anaerobic glycolysis and metabolic activity. – During glycolysis RBCs don’t produce CO2, they produce the organophosphate 2,3-DPG (aka 2,3-BPG). – 2,3-DPG is produced in an amount roughly equal to the RBC’s Hb content. – The level of 2,3-DPG rises when the erythrocyte is metabolically active and falls when activity diminishes. – 2,3-DPG is also essential to Hb’s ability to deliver (unload) O2 at the tissues. – The organophosphate stabilizes Hb in the deoxygenated state. – When the Hb is in the systemic capillaries, the 2,3-DPG present lowers the Hb’s affinity tor O2. – Low affinity for O2 causes the HbO2 to unload O2 and shifts the dissociation curve to the Right (Slide 13). This RBC does not contain HbS (sickle cell). Its flexible nature has allowed it to deform in order to pass through a small capillary Professor Michael Nazzaro Normal erythrocytes Normal adult RBC count: • Males 4.6 – 6.2 x 106 /mm3 • Females 4.2 – 5.4 x 106/mm3 Normal adult Hb: • Males 13.5 – 16.5 g/dl • Females 12.0 – 15.0 g/dl Normal adult hematocrit (Hct): • Males 40% - 54% • Females 38% - 47% (Note, 106 = 1,000,000) Reference: Note, Text pg. 253-54, 344 (Table 17-1) 17 Oxygenation: Hemoglobin (Hb) • Oxygen‘s low solubility in plasma (Slide 9) makes it impossible for the plasma to carry enough dissolved O2 to meet the normal, average, resting adult body’s need for about 250 ml of O2 per minute. • Hb makes adequate blood transport of O2 possible because, in theory, each of our 15 grams of Hb can carry 1.39 ml of O2. – The actual capacity is closer to 1.31 ml/g, but some writers use 1.34 or 1.35. (the Text uses 1.34 ml of O2 per gram of Hb). – In theory, O2 bound to Hb should = 1.39 ml/g x 15 g/dl = 20.1 ml/dl. – In fact, some Hb is abnormal (defective) and some is in shunted blood. – So bound O2 = 1.34 x Hb x % SaO2.. • In heathy adults bound O2 = 1.34 x 15 x 0.97 ≈ 19.5 ml/dl. • One of the most important characteristics of Hb is its ability to alter (change) its affinity* for O2 . – Hb is an allosteric molecule; it has the four primary binding sites on the hemes, but it also has secondary binding sites. – Atoms bound to secondary sites affect binding at the primary sites. – As each molecule of O2 binds or unbinds, it becomes easier for the next molecule to bind or unbind and so the processes of O2 binding and • The HbA molecule consists of four protein unbinding speed up. chains called globins; two alpha chains and two beta chains (shown in different colors) - Binding and releasing O2 causes a conformational (shape) change as the Hb molecule goes from the tense (T) state with LOW affinity for O2 and four porphyrin rings called hemes. to the relaxed (R) state with HIGH O2 affinity. • The globin chains are held together by salt Terms and abbreviations for the Hb types commonly seen in clinical practice: • Normal adult Hb is called HbA. There are many • Oxygenated Hb (oxyhemoglobin) is HbO2. other abnormal • Deoxygenated (Hb available to carry O2) is Hb. variants. See Text, • Carboxyhemoglobin (bound to Co) is HbCO. pg. 258-259 for • Fetal Hb is HbF (abnormal in adults). some of the more • Methemoglobin is called metHb types. • Sickle cell Hb is called HbS. *In chemistry affinity is the strength of the bonds that hold atoms together in compounds. In respiratory physiology, it is the strength of the bond that holds O2 to hemoglobin. • • • • bridges. Each heme contains an iron atom that acts as a binding site for O2. The four heme groups with their iron atoms are enfolded within the globin chains. Binding and unbinding causes conformational (shape) changes. Conformational changes alter the light refractive properties of the Hb and explain its color change. Reference: Note, Text pg. 255-61. 383, 1148-49 (Mini Clini). 18 O2 Transport: The Oxyhemoglobin Dissociation Curve The HbO2 curve showing the “Standard” curve and curves shifted Left (increased affinity) and Right (decreased affinity). All three curves are normal, the HbO2 curve shifts back and forth as the blood circulates between the pulmonary and the systemic capillaries. • The steep portion of the curve shows the greatest increase in SaO2 for each incremental increase in PaO2. • The flat portion of the curve shows little or no increase in SaO2 even when PaO2 increases. • Start with the P50 (dashed line), compare the different PaO2 levels and SaO2 points. • Right shift (red) occurs In the systemic capillaries for three reasons: 1. CO2 diffusing in from the cells lowers the pH. 2. The temperature in metabolically active tissues is higher. 3. The RBCs are actively producing 2,3-DPG. • Left shift (green) occurs in the pulmonary capillaries for three reasons: 1. Capillary pH is higher as CO2 diffuses out of the blood. 2. The temperature in the lungs is lower than in systemic tissue. 3. The RBCs are producing less 2,3-DPG. • Arterial Oxygen Saturation (SaO2) is the ratio between the amount of These ~ PaO2 Hb that is available to carry O2and the amount that is actually carrying % O2 approximate O2 (just like the relative humidity formula). The SaO2 formula is: (mmHg or PaO2-SaO2 Saturation correlations are Torr) extrapolated 50% 27 Torr from the O2Hb • The HbO2 Curve is a graph of the relationship between PaO2 and SaO2. dissociation curve 70% 40 Torr – The shifted curves show how Hb’s affinity for O2 changes in for normal response to physical (temperature) and chemical (pH and 2,3-DPG) 80% 50 Torr hemoglobin. They changes in the blood are a guide only, 90% 60 Torr • 2,3-DPG binds to deoxygenated Hb, lowering its affinity for O2 (R shift, which helps O2 unload at the tissues). NOT a substitute 100 & > • The HbO2 curve is sigmoid (S-shaped) because the relationship for an ABG. 96 – 100% between SaO2 and PaO2 is not linear like the relationship between PO2 and dissolved O2 which is linear. Torr Reference: Note Text pg. 254 & (Mini-Clini) 19 Changing Hb’s Affinity: The Bohr Effect & 2,3-DPG • Hb’s normal affinity for O2 is so high that it simply wouldn’t release O2 at the tissues. – Hb only works physiologically because it can change its affinity for O2 in response to changes in the environment the RBC is currently in. “Environment” means the particular capillary network the blood containing the RBCs happens to be perfusing. • Hb’s ability to lower (R shift) its affinity for O2 in response to increased CO2 (and the resulting decrease in pH) is called the Bohr effect (discovered by Christian Bohr). − Blood entering the systemic capillaries has a pH about 7.40. In the systemic capillaries, CO2 produced by the metabolically active cells diffuses into the capillaries and dissolves in the plasma lowering the pH to around 7.37. The reaction, which we have seen before, looks like this: CO2+ H2O <-------> H+ + HCO-3 − Hb affinity shifts to the Right because the CO2 and the free (loose) hydrogen ion (H+) bind to sites on the protein globin chain. CO2 binds to valine and produces carbamino compounds. The loose hydrogen ion (H+) binds to (and strengthens) the salt bridges connecting the globin chains together. All this tends to stabilize the globin in the Tense state and promote O2 release. • Obviously, the Bohr effect has a lot to do with CO2 transport in the blood. • Another important modulator of Hb affinity is the organophosphate 2,3-DPG (aka 2,3-BPG). − 2,3-DPG is a byproduct of anaerobic glycolysis (RBCs make it when they are metabolically active). One molecule of 2,3-DPG reacts with one molecule of Hb. Increased temperature raises 2,3-DPG production. • O2 binds loosely to the beta (β) globin chains of the Hb but it binds tightly to the alpha (α) chains. At the tissues, O2 molecules leave the β chains easily, but O2 tends to stay bound to the α chains. − The 2,3-DPG molecule slides into the cavity between the two β chains after they have released their O2, This allosteric binding changes the Hb molecule’s shape and promotes O2 release from the two α chains. That is the mechanism by which 2,3-DPG lowers Hb’s affinity for O2. • Increased DPG makes the Hb more efficient at unloading O2 at the tissues. − DPG production increases in anemia, chronic hypoxia and in chronic cardiopulmonary disease. • Decreased DPG makes the Hb more efficient at picking up O2 in the lungs. − DPG production decreases in chronic acidemia (CO2 retention in COPD) and in stored blood. Professor Michael Nazzaro Reference: Text pg. 257. 20 Oxygen Content of Arterial Blood: CaO2 • The significance of the last 14 pages of Text and the last 20 slides is that systemic arterial blood delivers the O2 molecules that the tissue cells need for metabolism. • The oxygen is delivered in two ways: dissolved and bound, and there are three clinical measurements of arterial O2. – We already covered the first two, the PaO2 and the SaO2. • By now it should be apparent that NEITHER the PaO2 nor the SaO2 adequately reflect the patient’s oxygenation status. • The content of arterial oxygen (CaO2) is the measure that reflects the total oxygen content of arterial blood and therefore, fully assesses oxygenation status. . • Oxygen content is measured directly by an ABG analyzer, or it can be calculated with the oxygen content equation. – The CaO2 can be expressed either as ml of O2 per dl or blood or as vol%. • To calculate the CaO2 we also have to know the patient’s total hemoglobin (HbTotal). • The classic version of the equation shown in the text is: CaO2 = PaO2 x (.003 ml O2/mm Hg/dl) + (Hb (in gm/dl) x (1.34 ml O2/gm Hb) x SaO2) • 0.003 ml of O2 will dissolve in each dl (100 ml) of plasma For every mmHg of pressure (Slide 9). • 1.34 ml of O2 can bind to each gram of Hemoglobin (Slide 12). • The clinical version of the equation is: CaO2 = (0.003 ml x PaO2) + (HbTotal x 1.34 x SaO2) • In our average normal healthy adult at rest the CaO2 is predicted to be: CaO2 = (0.003 x 100) + (15 x 1.34 x 0.97) = 0.3 + 19.5 = 19.8 vol%. That means that each deciliter (100 mL) of blood can carry almost 20 mL of O2. Professor Michael Nazzaro Reference: Note, Text pg. 254, Ch 6 Slide 48. 21 Oxygen Loading & Unloading: C(a-v)O2 • As the blood circulates through the systemic capillaries, it releases O2 according to the metabolic needs of the cells being perfused (how does it know?) • The C(a-v)O2 is the difference between the O2 content at the arterial end of the capillary and content at the venous end. –The C(a-v)O2 measures the amount of O2 taken up by (or delivered to or unloaded at) the tissues. • Normally, in healthy subjects at rest, each dl of arterial blood releases about 5 ml of O2 to the tissues. –That means that the normal C(a-v)O2 is about 5 vol% (or ml/dl). • Remember the structure of the circulation: – Arteries carry blood away from the heart. Veins carry blood back to the heart. Capillaries are the very short, thin-walled exchange vessels that connect arteries and veins. – The term exchange vessels means that the capillaries are where blood arterializes in the pulmonary capillaries and becomes venous again in the systemic capillaries. A relaxed, systematic survey of Fig. 11-9 (Text, pg. 261) reveals that it uses the standard HbO2 dissociation curve to examine the C(a-v)O2 concept. •The X axis plots dissolved O2 (as vol% without values) and PaO2 in torr. •The Y axis plots SbO2 (b = whole blood) as % saturation and CaO2 (in vol%). •Point V is blood just leaving the systemic (tissue) capillaries (unloading point) and entering the pulmonary capillaries. •Point A is blood just leaving the pulmonary capillaries (loading point) and heading for the systemic capillaries. •The line marked A-V Po2 difference (should be a-v) is read from the X axis. It shows the PaO2 about 100 torr and the PvO2 about 40 torr. •The A-V Hb difference (again, A should be a) is read from the Y axis. It shows the SO2 of A as about 97% and the SO2 of V as about 72%. Note that at a PO2 of 100 torr the HbO2 dissociation curve flattens out and SO2 no longer increases even though more and more O2 is dissolving in the plasma. Professor Michael Nazzaro Reference: Note, Slide 4 (Figure), Text, pg. 255-56 22 Physiological Oxygen Transport: Summary • Clinically, the CaO2 is a much more useful measure of oxygenation than the PaO2. – Healthy people, who have 15 vol% of normal Hb and a PaO2 of 100 torr will have a CaO2 of about 20 vol%. This CaO2 is adequate for a normal level of activity. In normal people a PaO2 of 100 torr by itself can be presumed to produce a normal SaO2 and a normal CaO2. • Unfortunately, in disease, it is possible for a patient to have a normal PaO2 and still be severely hypoxic, and the reported PaO2 value may not indicate the level of tissue hypoxia. – With adequate pulmonary ventilation none of the factors that lower CaO2 will show up in the PaO2 (O2 crosses the A/C membrane and dissolves in the plasma producing the Pressure that we read as PaO2). Factors that lower CaO2 without affecting PaO2 include: • Anemia* (reduction in Hb), HbCO (CO poisoning), metHb (methemoglobin), and various conditions that cause an excessive and permanent shift in the HbO2 curve. – The paradox of hypoxia with a normal PaO2 most often happens in one of two ways: Either there is a reduction in the amount of Hb or there is an abnormality in Hb’s affinity for O2. • On the other hand, a hypoxemic (low PaO2) patient may still have an adequate CaO2. – A defect in gas transfer across the A/C membrane can cause a low PaO2. But if the patient has an adequate supply of normal Hb he may be able to maintain an adequate CaO2. – For example, a patient with a PaO2 of 55 torr will have an SaO2 of 88%. If the patient has a normal Hb of 15 vol% we can calculate his CaO2: (15 x 1.34 X .88) + (0.003 x 55) ≈ 18 vol%. – The normal CaO2 ≈ 20 vol%. As long as our hypoxemic patient can increase his cardiac output, he can avoid hypoxia and oxygenate his tissue cells even though his PaO2 is low. *Note that anemia does NOT affect either the PaO2 nor the SaO2. It reduces the CaO2 and we must know the actual HbTotal to do the CaO2 calculation. Professor Michael Nazzaro Reference: Slide 13, Text, pg. 256 & 265 (both Mini-Clinis). 23 Gas Exchange & Transport RTT 100 Professor Michael Nazzaro Supplement to Text, Chapter 12 and Background for Chapter 42 You must study BOTH chapter 12 and this supplement Part 2: Carbon Dioxide Transport Revised 10-November-2021 24 CO2 Transport: Essential Terminology Definitions based on the BrØnsted-Lowry theory used in physiology Acid-Base physiology will be covered in depth during RTT 210, however there are some acid-base chemistry terms and concept that are essential to understanding CO2 transport: • Acid: any substance that donates a hydrogen ion proton (H+)* when hydrolyzed in an aqueous solution (aqueous means that water is the solvent in the solution). • Base: any substance that donates a hydroxyl ion (OH-) and is able to accept a H+ proton when hydrolyzed in an aqueous (water based) solution (alkaline and alkalinity are synonyms). – The terms strong and weak acids and bases refer to how completely the substance disassociates (ionizes) in an aqueous solution, not to the substance’s concentration. • pH: acidity (or basicity) of an aqueous solution is defined as the logarithm of the reciprocal of the hydrogen ion concentration (the negative logarithm of H+). In 1909 Sørenson suggested using the lower-case letter “p” to denote the H+. There are all kinds of explanations for what “p” stands for, the one I like best is the power (in both its mathematical and physical sense) of H+. • Buffer (buffer system, buffering, etc.): In the body, buffers are substances in the blood that prevent large, rapid changes in pH when H+ or OH- ions are added. • Acid-base balance refers to the physiological mechanisms to keep the H+ concentration in body fluids within a range compatible with survival (pH 7.35 – 7.45). *Chemists know that H+ (free hydrogen ion protons) do not exist in aqueous solutions, because the protons combine with water to form hydrated hydronium ions (H3O+). Medical textbook writers know this as well, but they use H+ as a convenient shorthand for acids in the body. Professor Michael Nazzaro Reference: Note, Text, pg. 259-61, 274-75, 1318 (definitions) 25 Physiologic Gas Transport: CO2 Transport • Blood transport of CO2 is much more complicated than O2 transport. Unfortunately, some writers manage to make it look even more complicated than it actually is. • CO2 is produced by the body cells during metabolism; the conversion of food to energy. Most texts state that normal tissue cell CO2 production is about 200 ml/min with a normal range of about 120 to 280 ml/min depending on body size and metabolic rate. The standard physiologic value for CO2 production (in health, at rest) is 2.4 ml/kg/min. • The CO2 is carried from the cells to the alveoli by the blood and excreted by ventilation. The blood carries a volume of about 45-55 ml/dl of CO2 in three forms in the blood. – CO2 transport operates in a reverse cascade of increasing pressures from the cellular level where PCO2 is high to the atmosphere where PCO2 is low (Text, Fig. 12-3) – CO2 diffuses into capillary blood because CO2 pressure in the interstitial fluid is about 4647 torr while capillary PCO2 is about 40 torr (see Note). This diffusion raises the capillary PvCO2 to the 46 or 47 torr shown in Text Pg. 247 Fig.12-3. • CO2 is about 20 times more soluble in plasma than O2, so a larger portion (5 - 10%) is simply carried dissolved in the plasma and in the RBC intracellular fluid. The rest is carried as reversible chemical combinations in the RBC and the plasma. These are two types of reversible combinations: carbamate compounds and bicarbonate. – Carbamates bound to plasma proteins are called carbamino compounds and those bound to Hb within the RBC are called carbaminohemoglobin (which is NOT carboxyhemoglobin). • Three modes of CO2 transport: 1. Dissolved in the plasma: about 5-10% (the Text uses 8%). 2. Bound as carbamates (mostly carbaminohemoglobin): about 12-22% (the Text uses 12%). 3. As bicarbonate ions both in the RBC and in plasma: about 80-90% (the Text uses 80%). Professor Michael Nazzaro Reference,: Note, Text, pg. 259-60 26 CO2 Transport: Chemical Events in the Blood A relaxed, systematic survey of Fig. 12-13 reveals the following information: • The left edge of the figure represents the tissue interstitial space (the tissue space). • CO2 diffuses into the capillary from the tissue space and O2 diffuses out of the capillary into the tissue space. – About 10% of the CO2 enters the plasma, of which: • 5% simply dissolves in the plasma. • 5% slowly ionizes to bicarbonate (HCO3-) and a free hydrogen ion (H+) • <1% combines with the free amino groups (NH2) of protein molecules in the plasma to form carbamino compounds (the prot-NHCOO- + H+ in the figure) and a free hydrogen ion (H+). – About 90% of the CO2 enters the erythrocyte, of which: • 5% simply dissolves in the erythrocyte intracellular fluid. • 63% quickly ionizes to HCO3- because erythrocytes have the enzyme carbonic anhydrase (CA) that makes the reaction almost instantaneous (actually it takes about 2 milliseconds). • 21% forms the carbamino compound carbaminohemoglobin when it binds to amino groups on the globin chains of deoxygenated Hb. Figure 12-13, pg. 261 clearly sums up the chemical events in CO2 transport in the blood. Binding to protein (the globin in Hb) buffers H+ and changes Hb’s affinity for O2 and CO2 • The Bohr effect (discovered by Christian Bohr) shows how high CO2 and H+ levels In metabolically active tissues lower Hb’s affinity for O2 (R shift) and increase Hb’s affinity for CO2. − Bohr speeds up unloading of O2; and deoxygenated Hb has a high affinity for CO2. • The Haldane effect (discovered by John Scott Haldane) describes how high levels of O2 in the lungs increase Hb’s affinity for O2 (L shift) and decrease its affinity for CO2. − Haldane speeds up of loading of O2 and oxygenated Hb (HbO2) has a low affinity for CO2. During all these rapid chemical changes in the plasma and the erythrocytes, chloride ions (Cl-) shift into the RBC to maintain electrolytic equilibrium (discovered by Hertog Jakob Hamburger). A reverse chloride shift occurs in the pulmonary capillaries. Professor Michael Nazzaro Reference: Note, Text, pg. 259-61. 27 The CO2 Dissociation and the Haldane Effect The Bohr effect and the Haldane effect are examples of heterotrophic interactions: where the presence of one molecule (CO2) affects the binding of a second molecule (O2) to a third molecule (Hb). • The Haldane effect describes how high levels of O2 in the lungs increase (L shift) Hb’s affinity for O2 and thereby decrease its affinity for CO2. Blood in the pulmonary capillaries is in a high O2 environment. − Haldane’s effect describes how high O2 levels speed up loading of O2 onto Hb at the lungs, producing saturated Hb (HbO2). − HbO2 has a low affinity for CO2, so unloading of CO2 and loading of O2 are simultaneously speeded up at the lungs. − Haldane’s effect also works at the tissues but to a lesser degree. • The Bohr effect (Slide 13) describes how high levels of CO2 and H+ in the tissues decrease (R shift) Hb’s affinity for O2 and thereby increase its affinity for CO2. The bottom half of Fig. 12-14, pg. 261. – Blood in the systemic capillaries is in a high CO2 and H+ The CO2 Dissociation Curve graphs environment. the Haldane effect. – Bohr’s effect describes how high CO2 and H+ levels speed up The colored curves represent SaO2. unloading of O2 at the tissue cells, producing deoxygenated Hb. The PCO2 (x axis) and CO2 content (y axis) are shown at two physiologic – Deoxygenated Hb has a high affinity for CO2 so loading of CO2 SaO 2 values and an extreme value for and unloading of O2 are simultaneously speeded up at the comparison. tissues. • Point a on the 97.5% saturated curve – Bohr’s effect also works at the lungs, but to a lesser degree. shows a PCO2 of 40 torr and a CO2 • During all of these rapid chemical changes in the plasma and the content of 48 vol%. erythrocytes, chloride ions (Cl-) shift into the RBC to maintain • Point v is on the 70% saturated curve electrolytic equilibrium (a phenomenon named after its discoverer, shows a PCO2 of 46 torr and a CO2 content of 52 vol%. Hertog Jakob Hamburger). • CO2 content increases as O2 saturation decreases. Revised 05-January-2020 Professor Michael Nazzaro Reference: Slide 4 (Figure), Text pg. 261 28 Gas Exchange & Transport RTT 100 Professor Michael Nazzaro Supplement to Text, Chapter 12 and Background for Chapter 42 You must study BOTH chapter 12 and this supplement Part 3: Gas Exchange Abnormalities Revised 10-November-2021 29 Gas Exchange Abnormalities: Introduction • The primary purpose of breathing is gas exchange. o Adequate breathing requires a level of VA that is sufficient to take in enough O2 and eliminate enough CO2 to meet the body’s physiological needs. • It is important to remember that what appears to be normal breathing (“normal” tidal volume and respiratory rate) does not automatically guarantee adequate oxygenation. o In fact, hyperventilation is one of the first signs of hypoxemia and hypoxia. ሶ The ሶ 2) is the product of O2 content (CaO2) and Cardiac Output (Q). • Oxygen delivery to the tissues (DO ሶ 2 formula is: DO ሶ 2 ≈ 990 ml/min If Hb =15 vol%, SaO2 = 97%, PaO2= 100 torr, and CO (Q)ሶ = 5 l/min, then DO ሶ 2 = ((15 x 1.34 x .97) + (0.003 x 100)) x 5 x 10 = 990 (multiplying by 10 converts ml/dl to ml/L). 𝐃O ሶ 2 is inadequate to meet tissue needs, the result is hypoxia. • When DO • When the PaO2 is below the predicted normal for the patient’s age, the result is hypoxemia. • Since hypoxia and hypoxemia have a range of causes that often require different forms of treatment, the clinician has to understand the underlying pathologies of hypoxia, for example: o A patient who is hypoventilating will have hypoxemia. Normobaric O2 therapy will correct the hypoxemia (but it will not correct the cause of the hypoventilation). o A patient with a low V/Q (< 1 but > 0) will need O2 under positive pressure to correct the hypoxemia by opening collapsed alveoli. o A patent foramen ovale (the opening between the right and left atria in the fetal heart) that doesn’t close spontaneously after birth will require surgery to close the hole. Professor Michael Nazzaro Reference: Text, pg. 261, Slide17 30 Abnormalities of Oxygenation: Hypoxemia NORMAL PAO2 for any age is > 80 mmHg. • Hypoxemia is defined as a PaO2 lower than the predicted value for the patient’s age (from the equation for older adults on Pg. 263): PaO2 = 100 − (0.323 x age in years). • Hypoxemia is classified as (on room air at sea level): o Mild (PaO2 70-79 torr) which is only slightly < than normal), o Moderate (PaO2 60-69 torr). o Severe (PaO2 50-59 torr). A PaO2 value < 40 mmHg is usually incompatible with life. • There are two basic causes of hypoxemia: 1. An inadequate amount of oxygen is reaching the alveoli which causes a low PAO2. Likely causes include inadequate breathing producing a low alveolar minute volume (VA) which may result from: o Overall hypoventilation: ▪ The PAO2 is down because the PaCO2 is up. ▪ The P(A-a)O2 will remain normal because blood perfusing the underventilated alveoli will have a low PaO2 that equilibrates with the low PAO2. o Tachypnea: ▪ Rapid, shallow breathing increases deadspace ventilation because a higher proportion of the lower VT ventilates the fixed amount of anatomic deadspace (remember, Ve ≠ VA from Ch. 11). 2. An inadequate amount of oxygen is crossing the A/C membrane into the blood. Likely causes include: ▪ Low PB causing a low PIO2 , (e.g., mountain climbing, aircraft depressurization, etc.). ▪ Low FIO2 (e.g., being in a closed room with a combustion heater). o Textbook descriptions of diffusion defects across the A/C membrane can be confusing because anything that reduces O2 transfer across the A/C membrane can be classified as a diffusion defect; including entities ranging from low PIO2, all the way to circulatory failure. o Mixing of low PO2 blood with high PO2 blood is also potentially confusing because of the diverse terminology used in textbooks. –Both diffusion defects and shunts will be covered separately. Professor Michael Nazzaro Reference: Text pg. 263 (Fig. 12-15), 264 (Fig. 12-17), 373 (Table 19-1), 1148-49 & Mini-Clini 31 Poor Oxygenation: Ischemia, Shock, & Dysoxia Additional causes of tissue hypoxia involve cardiovascular inadequacies and cellular toxicity 1. Ischemia is the term for a temporary local reduction of oxygenated blood flow to a particular tissue or organ. – Occlusion of a blood vessel will reduce blood flow in the tissue being perfused. If a coronary artery is occluded the result may be a myocardial infarction. If the vessel is in the brain the result may be a stroke. 2. Shock is the term for generalized circulatory failure characterized by excessively low blood pressure. – Shock occurs when the cardiovascular system cannot adequately perfuse the body’s tissue cells. This may result from failure of the heart as a pump, inadequate blood volume, or inability of the blood vessels to produce sufficient muscle tone to maintain pressure. 3. Dysoxia (aka histotoxic hypoxia, hypoxidosis) is a condition in which the cells are unable take up and use O2 normally. In these cases, the PaO2, CaO2, SaO2, and O2 transport are all normal, but venous blood returns to the heart with virtually the same PO2 it had when it returned to the left atrium from the lungs. − The classic illustration for dysoxia is cyanide poisoning. • Cyanide inhibits the intracellular enzyme cytochrome oxidase • Cytochrome oxidase is essential to allow the mitochondria to use O2. • Dysoxia is also seen in Adult Respiratory Distress Syndrome (ARDS), now usually called Acute Respiratory Distress Syndrome. Dysoxia is also seen in septic shock. – Septic shock reduces blood flow past the peripheral chemoreceptors (cells located in the aorta and carotid arteries that respond to low O2 levels). This low blood flow causes an increase in the ventilatory drive. • Cyanide alters the neural conductivity of the peripheral receptors and produces a brief period (lasting seconds to minutes) of hyperpnea before death. Professor Michael Nazzaro Reference: Note, Text, pg. 264-265 & Fig. 12-19. 32 Diffusion Hypoxia: Clinical Problem 1. An 18 YO male patient is in the recovery room after an uncomplicated appendectomy. He is awake and alert and breathing normally, but the nurse reports that his SpO2 is 88%. You put the patient on a simple O2 mask at 8 L/min (FIO2 ≈ .6) and within several minutes his SpO2 increases to 99%. What caused the low SpO2 and why did the O2 mask correct the problem? – The patient’s condition is diffusion hypoxia which often happens after anesthesia with nitrous oxide (N2O). – Nitrous oxide anesthesia (N2O) is administered by inhalation, so it diffuses across the A/C membrane into the bloodstream where it reaches the CNS and produces anesthesia. – After surgery, the residual N2O diffuses out of the blood into the alveoli and is excreted through the lungs. – During the time it takes to clear the N2O from the blood, the gas displaces O2 in the alveoli and causes a mild hypoxia. – Giving the patient O2 displaces some of the nitrogen (N2) in the alveoli and increases the PAO2 by allowing more room for O2. • If we could measure the patient’s PaN2O we could calculate his actual PAO2 and then calculate his P(A-a)O2. • Since we aren’t able to do those calculations, we have to treat empirically, that is the treatment has to be based on clinical signs (the low SaO2) and our clinical intuition and judgment. Professor Michael Nazzaro Reference: Note 33 Pulmonary Physiology: Pulmonary Shunting A Shunt occurs when volumes of oxygenated and deoxygenated blood mix. Terminology for shunts is confusing: True Shunt (absolute shunt) and Shunt-like Effect are names for broad categories: True Shunts can be anatomic or capillary shunts. True shunts are refractory to oxygen therapy. • Anatomic Shunts occur when blood passes from the right side of the circulation to the left side without entering the pulmonary capillaries. – The normal anatomic shunt (about 2-5% of cardiac output) arises from the portion of bronchial, pleural, and coronary venous blood that empties directly into the pulmonary veins and the left atrium. – Many pathological conditions can cause anatomic shunts including congenital heart defects, and tumors of the pulmonary vasculature to name just two. • Capillary Shunts occur when blood from the right circulation passes through the pulmonary capillaries, but no gas exchange occurs. – The most common cause of capillary shunts is atelectasis. Professor Michael Nazzaro Shunt-like Effect occurs when pulmonary perfusion exceeds alveolar ventilation. • Most common causes of shunt-like effect are hypoventilation, uneven distribution of ventilation, and A/C diffusion defects. • Shunt-like effect is usually responsive to oxygen therapy. • Venous Admixture is the final result of all types of shunting. Venous admixture is just a fancy way of saying that various aliquots of deoxygenated blood mix with oxygenated blood and lower the overall O2 content of the arterial blood. Reference: Text pg. 250, 266-67, 974 & Fig.44-2 34 Pulmonary Physiology: Normal V/Q Ratio Ventilation Alveolar ventilation (𝐕ሶ A): Alveolar ventilation: ( VA) 𝐕ሶ A