MEC2181 CFD Lecture Notes 2024-2025 PDF
Document Details
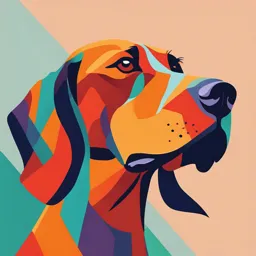
Uploaded by CushyOnyx2073
Singapore University of Technology and Design
2024
Dr Victor Wang
Tags
Summary
These lecture notes introduce the concept of Computational Fluid Dynamics (CFD). They cover fundamental topics like Reynolds number, turbulence, and different approaches to understanding fluid dynamics. The notes also discuss the standard procedures for using CFD, including geometry and mesh creation, and simulation process.
Full Transcript
MEC2181 Dr Victor Wang Email: [email protected] Office: 65928510 Objectives Introduce students to: Concept of Computational Fluids Dynamics (CFD) The relevance of CFD in today’s world Fundamentals/standard procedure (best practice) of...
MEC2181 Dr Victor Wang Email: [email protected] Office: 65928510 Objectives Introduce students to: Concept of Computational Fluids Dynamics (CFD) The relevance of CFD in today’s world Fundamentals/standard procedure (best practice) of using CFD The possible challenges/difficulties – and the solutions needed to be presented to address them Particularly, External flows Shown live demo on how to use a CFD package Important steps needed to run a proper CFD simulation What is CFD? Computational Fluids Dynamics – CFD The use of computer aided tools (numerical methods) to design/replicate/simulate real-life situations that involves Fluid motion Turbulence & Reynolds number One of the most common features in Fluid Dynamics is its inherent turbulent nature. For an internal flow (a pipe flow), the Reynolds number: Hydraulic Diameter It is good to be aware of various Dh of different geometries commonly used in internal flows: Turbulence & Reynolds number The three regimes of viscous flows (a) laminar flow at low Re, (b) transition or intermediate flow, (c) turbulent flow at high Re Turbulence & Reynolds number Low Reynolds number, flow is laminar. Fluid particles move in a very orderly manner, essentially along pathlines in layers, without mixing of fluid particles across layers Laminar flow is characterized by smooth streamlines and highly ordered motion. Turbulence & Reynolds number High Reynolds number, flow becomes turbulent Mixing is very significant and fluid particles move haphazardly in all directions. Flow characterized by an unsteady fluctuating (i.e., random and 3-D) velocity components superimposed on a temporal steady mean (i.e., along the pipe) velocity. Turbulent flows are characterized by velocity fluctuations and high disorganized, chaotic motion. Turbulence & Reynolds number The changeover is called transition to turbulence. Transition does not occur suddenly; it occurs over some region in which the flow fluctuates between laminar and turbulent before it becomes fully turbulent. http://www.youtube.com/watch?v=NplrDarMDF 8&feature=player_detailpage Turbulence & Reynolds number For a pipe flow (internal flow): Flow in a pipe is: Laminar when Re < 2100 Turbulent when Re > 4200 For practical purposes, the Sketches of pipe flow critical value for flow transition transition: (a) low speed, is taken to be laminar flow; (b) High speed, turbulent flow Recrit = 2300 Turbulence & Reynolds number Note that Recrit is different for different geometries and flow conditions. It is desirable to have precise Reynolds number to determine flow behavior – not true in real practice. Besides the factors (?) mentioned already, the transition from laminar to turbulent depends also on upstream & downstream effects of: Degree of surface roughness Vibration 3 main approach to understanding Fluids 1.Theoretical 2.Experimental 3.Computational Theoretical Approach Theoretical/analytical studies of fluid dynamics involves: Solving of mathematical equations (known as Navier-Stokes equations) that represent the physical principles behind the behaviour of fluid flows in given volume of space Mass, momentum and energy are conserved here Theoretical Approach Examples of the equations for a compressible flow are: (Conservation of mass) (Conservation of momentum) Theoretical Approach However, to adopt a theoretical approach, these equations are usually simplified or fluid flows are analysed in ideal assumptions. As flows become complicated (or rather machineries becoming more complicated), sophisticated applied mathematics are required to study these flows. Experimental Approach Experimental studies in fluid dynamics are more common Methods have also been adopted and improved over the years to improve the quality (accuracy & details) of the experimental results. Nevertheless, until only very recently the outcome of most fluids experiments were mainly a qualitative (and not quantitative) understanding of fluid motion. Experimental Approach Always an issue against time and cost. Often, large scale resources have to be available in order to achieve desired results. It is usually very difficult and challenging to provide and accommodate to every single detail and parameters that are required for the experiment. Physical limitation of each set-up often proves to be a stumbling block for research to progress and advance. Scaled model might not be representative Measurement error Experimental Approach Nevertheless, still the most important approach in the design process http://www.youtube.com/watch?v=2CYUuBiW_lY&f eature=player_detailpage Question now is how can we: Minimize cost of running an experiment At which stage of a design process should experiments be conducted The most efficient of an experiment Get the most out of an experiment The above 4 points…can be aided by the 3rd approach Computational Approach Constitutes the new ‘third approach’ in the study of Fluids Dynamics after the traditional two approaches of theoretical studies and experimental methods. Pure experiment Theoretical approach 17th century 18th &19th century CFD 1960’s Computational Approach First introduced in the 60’s Always this industrial need for both theoretical and experiment methods CFD was used to compliment the traditional two approaches of theory and experiment Understanding of fluids dynamics lies on the proper balance of these three approaches with CFD providing the ability to interpret, analyze and understand the results of theory and experiments and vice versa Alternative to Experiment & Analytical Cheaper and faster More complete information Practically unlimited amount of information Higher degree of complexity/difficulty Can be used for any problems CFD is however dependent on: Solving of Mathematical equations Quality of CFD code/solver Governing equations Boundary conditions – what is input to get the desired output Computer Hardware http://www.youtube.com/watch?v=P3ulqzRR9UA&feat ure=player_detailpage So Why use CFD….? Increasing confidence in the use of CFD on many applications (e.g. Aerospace/ Oil & Gas/Marine Offshore/ Wind Engineering….) CFD originally used as a research tool, but is increasingly becoming an alternative to experiments (design tool) – due to lower cost/faster turn-around time/modification of conditions) Fluids in Today’s world Fluid mechanics: is that branch of applied mechanics that is concerned with the behaviour (statics – at rest and dynamics – in motion) of liquids and gases. The analysis of the behaviour of fluids can be branched out into sub-disciplines (list not exhaustive) such as: 1. Aerodynamics 2. Hydraulics 3. Geophysical fluid dynamics 4. Bio-fluid mechanics….and many more. The importance of fluid dynamics will be stressed here, by providing specific examples from two main areas: 5. Pure sciences 6. Technology Pure Sciences Ocean Geo Sciences Biological Typhoon Haiyan Astrophysics/Atmospheric Fluids in Technology Buildings (HVAC) Automotive Marine Aerospace Technology Gas Turbine Engine Military Jets Civil Jets Sports Technology How do we use CFD? Geometry and Mesh How do we use CFD CFD code varies from simple 1-Dimensional (1D) code to 2D/3D – frequently used now due to improving computer resources and demand for larger data analysis and visualization Commercial software (ANSYS products etc..) In-house academia/industrial codes Always requires a geometry to start with – geometry describes the shape/domain of the problem that needs to be solved Mesh/grid needed to represent the geometry (discrete representation) Which the flow problem is solved Numerical Methodology Numerical Methodology of CFD Solving (discretisation) of 3 equations 3 underlying physical principles of fluid flows – Navier Stokes Equations) 1. Conservation of mass: 2. Conservation of momentum: 3. Conservation of Energy: 3 different methods involved in CFD modeling 1. RANS – Reynolds Averaged Navier Stokes 2. LES – Large Eddy Simulation 3. DNS – Direct Numerical Simulation Standard procedure of CFD Identifying of problems – Previous case study/experimental work/flight data/test results Visualisati High performing on Solution software: computing domain Tecplot Geometr (HPC) y 360, Fieldview, Pre- Actual Post- Excel processing Simulation processing spreadshe et,Matlab …. CFD code (e.g Detailed Boundary Mesh Commercial based/ analysis of Conditions numerical in-house) solutions Validation/Comparing against existing (valid) data to judge performance (accuracy) of CFD predictions Developing a basic Computational Fluid Dynamics (CFD) model 1. Geometry (using ANSYS Spaceclaim) 2. Mesh (using ANSYS Meshing ) 3. Boundary Conditions (ANSYS FluentTM) 4. Simulation, results and analysis (ANSYS FluentTM ) Geometry Modelling a fluid domain In CFD, the fluid domain (e.g., surrounding air) will be modeled to solve for the flow physics. The flow behaviour of a solid body (also known as the ‘bluff body’, e.g., a cylinder/airfoil) within the fluid domain will be investigated by observing key flow parameters (e.g., velocity, pressure, lift/drag forces). H 5 10H 5 H H Geometry Modeling a fluid domain Sketch Mode End Sketching Tool – Once sketching Rectangle is completed, press the ‘End Sketching’ Tool Geometry Modeling a fluid domain Press ‘Esc’ key to remove these modeling options before continuing to the next step Once sketching is successfully completed, a 2D surface will be generated based on the sketch Geometry Modeling a fluid domain This is the expected outcome of the interface after pressing the ‘Esc’ key Geometry Sketching a circle Sketch a circle at the origin axis H (representing a 2D 5 10H cylinder) 5 H H Geometry Modeling the bluff body It can now be observed that there is a 2D cylindrical geometry (bluff body) within the larger rectangular surface (fluid domain). The purpose of the next step is to separate the bluff body from the fluid domain and remove the interior of the bluff body. This way, the fluid domain will be left with the outline/boundary of the cylindrical bluff body. Geometry Select the ‘Combine’ Select the rectangular fluid tool domain as the target object Geometry Spaceclaim will navigate to the Cutter function by fault. Select the cylindrical surface. This will Cutter allow the geometry to ‘cut’ the cylindrical tool surface from the larger fluid domain Geometry The 2D cylindrical surface can now be selected separately from the larger fluid domain Geometry Select the surface of the 2D cylinder. Right-click and select the ‘Suppress for Physics’ option After the surface is suppressed Geometry The bluff body domain can be deleted to retain only the outline of the bluff body. Right-click on the bluff body domain and select the ‘Delete’ option. Geometry Creating Named Selection. In CFD modeling, boundary conditions are critical and must be applied to the different sections of the geometry. Numerical computation will be performed on the CFD model based on the different boundary conditions applied. Select an edge (for 2D geometry) or surface (for 3D geometry). Click on the ‘Create NS’ option. Specify an appropriate name for the different boundary conditions (e.g., Inlet, Outlet). Bluff body removed Geometry Symmet ry Outle Inl t et cylinde r Symmetr y Mesh Navigate back to the Workbench folder. Please ensure that there is a green tick beside the Geometry module. Search for the Mesh module within the toolbox. Drag the module and link it with the Geometry module. Right-click on the Mesh module and select Edit to enter the meshing interface (Double-clicking the Mesh module also works) Mesh The ANSYS Meshing interface will display the geometry of the fluid domain that is transferred from Spaceclaim Under the Physics preference tab, select CFD from the drop- down menu Mesh Under the Element Size tab, apply an element size of 0.01m. Generate Mesh Click Generate Mesh to commence the meshing option process The fluid domain will be discretised into small grids with a size of 0.01m where the flow physics within this domain will be numerically solved in each of the grids Mesh Completed Mesh model of Fluid domain Simulation (Setting up of correct BC) Simulation (Setting up of correct BC) Navigate back to the Workbench folder. Right-click on the Mesh module and select Update. Please ensure that there is a green tick beside the Mesh module. Drag the Fluent module and link it with the Mesh module. Right-click on the Fluent module and select Edit to enter the CFD solver interface (Double-clicking the Setup tab also works) Simulation (Setting up of correct BC) Simulation (Setting up of correct BC) The purpose of the simulation is to observe the effect of a simplified 2D cylinder on an external turbulent environment. To obtain results that make physical sense, the initial and boundary conditions must be accurate, and the assumptions made should be physical. The pre-processing steps consist of assigning these conditions prior to running the simulation and analysing the results. Ensure that the solver is in steady-state mode Under Models, select Viscous. By default, the solver is using the k-omega SST turbulence model to model the viscosity effect within the fluid domain Change the k-omega SST to k-epsilon. Leave other parameters unchanged Simulation (Setting up of correct BC) Ensure boundary conditions Under the velocity are rightfully assigned. inlet boundary Fluent can detect the condition, assign an appropriate boundary arbitrary velocity of conditions based on the 10 m/s under the names assigned Velocity Magnitude option. Leave the rest of the boundary conditions unchanged Simulation (Methods) Simulation (Controls) Simulation (Pre-processing) Hybrid Initialisation Simulation (Running of Simulation) Once Hybrid Initialisation is completed, proceed to Run Calculation. Assign 1000 iterations and click Calculate to start the simulation process Simulation (Post-processing) Reminder: Standard procedure of CFD Identifying of problems – Previous case study/experimental work/flight data/test results Visualisati High performing on Solution software: computing domain Tecplot Geometr (HPC) y 360, Fieldview, Pre- Actual Post- Excel processing Simulation processing spreadshe et,Matlab …. CFD code (e.g Detailed Boundary Mesh Commercial based/ analysis of Conditions numerical in-house) solutions Validation/Comparing against existing (valid) data to judge performance (accuracy) of CFD predictions Internal Pipe Flow (Case description) Steady state Outlet Laminar and Turbulent Incompressible Wall 𝐷=40 𝑚𝑚=0.04 𝑚 𝐿=3 𝑚 3 𝜌 𝑤 =1000 𝑘𝑔 /𝑚 𝜇 𝑤 =0.001 𝑃𝑎. 𝑠 Inlet or Laminar, let us set Reynold’s Number to 1000 𝜌𝑤 𝑣 𝐷 𝑅𝑒= 𝜇𝑤 This leads to pipe velocity as below: 𝑣 =0.025𝑚 /𝑠 or Turbulent, let us set Reynold’s Number to 20,000 This leads to pipe velocity as below: 𝑣 =0.5 𝑚/ 𝑠 Internal Pipe Flow (Workbench) Another way, is by creating an “Analysis System” which include all simulation steps Drag and drop “Fluid Flow (Fluent)” in the “Project Schematic” Rename the case as shown Geometry Right-click on “Geometry” and choose “SpaceClaim” In Ansys, there is also two more CAD software: “Discovery” and “DesignModeler” In recent versions, “SpaceClaim” is the default, so if you double-click on “Geometry” it will automatically lunch “SpaceClaim” Geometry Click “New Sketch Plane” Geometry 2- Click on the “Circle” tool 3- Click on the origin to start sketching the circle at the origin, then input 40 mm for the circle diameter 1- Hoover the mouse over the “Z” axis, until you see the XY plane visible, then click on the Z Axis, to draw a circle in the XY plane Geometry Notice the creation of a “Surface” because we didn’t extrude it yet Click on the “Return to 3D mode” or on “End Sketch Editing”, alternatively you can click on “D” letter on the keyboard Geometry 1- Click on “Pull” to extrude the circle to a cylinder 2- Click the circle face and pull towards the –ve Z-axis, so that the inlet stays at the origin 3- Input 3000 mm as the extrude distance then press “Enter” 4- Press “Esc” on the keyboard twice to exit the “Pull” command Geometry Notice the generation of a solid “Body” You can rotate by clicking and dragging the middle mouse button And can always return to the default isometric view by clicking the shortcut keyboard key “H” Geometry Name boundary conditions (can also be done in the meshing step) 1- Click on the left pipe face 2- Click “Groups” then “Create NS” and type “inlet_pipe” 3- Repeat the steps for the outlet and pipe walls Geometry You should have by now three named surfaces as shown Next we go to the meshing step 1- Save the project, close “SpaceClaim” 2- Double click on “mesh” in the project schematic Mesh Right-click on “mesh”, then “Insert”, then “Method” Mesh Make sure you select “Body” in the selection filter panel. Click on the pipe, it should become green Click on “No selection” highlighted in yellow and click “Apply” “1 Body” should be shown in front of “Geometry” Mesh 2- Right click “Mesh” then hoover the 1- Change mouse on Method from “Insert” and “Automatic” to choose “MultiZone” “Inflation” 3- Choose the pipe body as the “Geometry” And pipe wall face as the “Boundary” 4- Inflation option: First Layer Thickness First Layer Height: 1 mm Keep the rest default Mesh Click on “Mesh” Then “Generate” The mesh should look like this If satisfied with the mesh click “Update” The project schematic should look like this Save the project, close the meshing software and move to Simulation (Setting number of cores) Keep the default “Double Precision” Use “4” for “Solver Processes” (This number can be increased for computers with more cores Simulation (Adding Gravity Vector) Check “Gravity” And add - 9.81 in the Y-axis This step will not make any difference in this case because it is single phase flow in a horizontal pipe. But it is always recommended to add gravity vector Simulation (Setting length units) 2 1 3 4 Simulation (Adding Liquid Water) Currently, air is in the materials, we need to add 1 water 3 4 5 2 Simulation (Adding Liquid Water) As seen, water-liquid is added to the materials list. But it is still not added in the fluid domain In the next slide, we assign water in the fluid domain, To change fluid properties, either double- and can “Delete” air click on the fluid or “Create/Edit” Change “Density” to 1000 and “Viscosity” to 0.001 then click “Change/Create” Simulation (Adding Liquid Water) 1 2 Assign “Water-Liquid” in the 3 “Cell Zone Conditions” Then can go back to the “Materials” and delete “Air” Simulation (Adding Liquid Water) 1 2 Assign “Water-Liquid” in the 3 “Cell Zone Conditions” Then can go back to the “Materials” and delete “Air” Simulation (Part 1: Laminar case) First, we start with the laminar flow case, therefore we choose “Laminar” in the “Viscous Model” menu Simulation (Boundary Conditions) 2 Change “Velocity 1 3 Magnitude” to 0.025 Keep Gauge Pressure as Zero, simulating a Double-click pipe discharging to “inlet_pipe” or the atmosphere highlight it and click “Edit” Keep everything for the wall_pipe default Simulation (Pre-processing) Hybrid Initialisation 2 3 1 Simulation (Report: Mass balance) 2 1 3 Mass in should be equal to Mass out So, subtracting both should give value as close to zero as possible We generate a report for this Simulation (Report: Mass balance) If you click on “Compute”, you can 1 see the value as below in the “Console” window: 3 After only “Hybrid Initialisation”, acceptable mass imbalance can be seen 2 3 Simulation (Running of Simulation) Set “Number of Iterations” to 1000 then click “Calculate” As seen, residuals are reducing nicely during iterating Solution converged almost halfway, before 1000 iterations Simulation (Running of Simulation) Mass-conservation also reducing nicely to almost zero Simulation (Running of Simulation) Mass-conservation also reducing nicely to almost zero e the project and move to the next step; Post-Processing Simulation (Post-Processing) Green check mark should be appearing in front of all previous st Double-click on “Results” to launch Ansys Post-Processing modul Simulation (Post-Processing) Click on “location” and choose “Plane” from the dropdown menu Name the Plane “Plane Symmetry” for example Click “OK” Simulation (Post-Processing) Method “YZ Plane” X=0 You should be able to see a plane like this cutting throughout the pipe Simulation (Post-Processing) With these icons, you can visualise contours, vectors, streamlines…..etc of different parameters such as velocity, pressure, temperature….etc Click on “Contours” and Name: “Contour Velocity” Simulation (Post-Processing) Select these choices and click “Apply” Click on the X-axis to see a view perpendicular to it Velocity contours show uniform velocity at inlet, and developing to a fully developed behaviour in the flow direction Perpendicular to pipe axis, velocity is zero at the pipe walls and increase to maximum at pipe centerline Simulation (Post-Processing) Create a line in the fully developed region along the pipe vertical diameter Simulation (Post-Processing) Input these dimensions to specify the line Click “Apply” You should be able to see a yellow line slightly before the pipe outlet in the fully- developed region Simulation (Post-Processing) Click on the “Chart” symbol and name the chart “Velocity Profile” Click “OK” Simulation (Post-Processing) Data Series: Choose the “Vertical Diameter” line that we created earlier X Axis: Choose Variable “Velocity w” (because the flow is in –ve Z-Axis Y Axis: Choose Variable “Y” Click “Apply” Simulation (Post-Processing) Notice, parabolic velocity distribution, proving its laminar flow Velocity values are –ve because the flow is in the negative Z-direction n always toggle between 3D viewer (contours, vectors…etc), charts, tables from Simulation (Part 2: Turbulent Flow) Right-click on this arrow, click “Duplicate” to create a copy of the Laminar case A copy will be created. You can double-click the name to change the case name to “Turbulent Internal Pipe Flow” Double-click on setup, to launch “Fluent” and proceed with the Turbulent flow case Simulation (Turbulence Model) 2 1 3 4 5 ge the Viscous model to k-omega, keep everything else default Simulation (Boundary Conditions) 2 1 3 4 5 Double-click “inlet_pipe” or Change “Velocity Magnitude” to 0.5 highlight it and click (Corresponding to Re = 20,000) “Edit” Specification Method to “Intensity and Hydraulic Diameter” “Hydraulic Diameter” to 40 Then click “Apply” Notice the appearance of new options when applying a turbulence model Simulation (Boundary Conditions) 1 2 For “outlet_pipe” keep everything default Note: Gauge pressure is kept at Zero (simulating pipe discharging to the atmosphere Simulation (Boundary Conditions) “wall_pipe” is set as “Stationary Wall” You can input “Roughness Height” as per pipe material Let's assume it is Galvanized steel (0.15) ed to initialise and run the case exactly as done for the “Laminar” case Simulation (Running of Simulation) As seen, residuals are reducing nicely during iterating Solution converged even faster than Double-click on “mass- the laminar case conservation” report and click compute. Simulation (Post-Processing: Comparing Laminar and Turbulent) Drag “Solution” from both cases and connect to the newly created “Results” 3 2 4 Drag “Results” from “Component Systems” and drop it in the “Project Schematic” Double-click the combined “Results” to launch CFD- Postprocessing module which will 1 display both cases Simulation (Post-Processing: Comparing Laminar and Turbulent) Cases arrangement for viewing can be adjusted from here 4 Proceed to create “symmetry plane” then velocity contours as previous Simulation (Post-Processing: Comparing Laminar and Turbulent) The difference between laminar and turbulent velocity profile can be observed Multiphase VOF (Dam breakup: Case description) Transient Incompressible Two-phase (Air and water) Water column is suddenly released, water flows down due to gravity, towards the step Dynamic mech adaption will be used to Width = 200 mm refine the mesh at areas of interest (air- water interface in this case) and coarsen the mesh everywhere else. Leading to accurate simulation while keeping mesh count reasonable. Multiphase VOF (Workbench) 3 Right-click “Geometry”, then “Replace Geometry” then “Browse”, locate “geometry_dam.scdoc” file to load the prepared geometry. You can then open it by clicking “Edit Geometry in SpaceClaim. Notice that faces are named already Next, double-click on “Mesh” 1 This time, we will use the 2 meshing capability of Rename the case: Fluent directly. “Dam Break + Drag and drop this Dynamic Mesh system Adaption” Geometry In recent versions, “SpaceClaim” is the default, so if you double-click on “Geometry” it will automatically launch “SpaceClaim” Mesh Fluent with meshing looks like this After the meshing is done, it can easily shift to Fluent solver by First clickstep this is to import the geometry. Click “Import Geometry” Mesh Insert clipping plane to see inside the fluid domain Mesh For now no need to add local sizing Click “Update” Mesh In “Generate the Surface Mesh” keep everything default, but you can also refine or coarsen the mesh here Mesh Notice the generation of In this step, use surface mesh the settings as after the previous shown step Then click “Describe Geometry” Mesh (Can also add Multizone to generate Hex only elements) Enable Multizone meshing: “Yes” Mesh (Can also add Multizone to generate Hex only elements) After enabling “Multizone”, this tab will appear Mesh We can set boundary condition types in this step, and also can change them in Fluent later when we “Switch to Solution” mode Keep all as “wall” then click “Update Boundaries Mesh There is only one “Fluid” region in this simple case. Click “Update Regions” Mesh In this step, we can add inflation layers. Change “Number of Layers” to 10 and click “Update” Mesh Last step is to generate the volume mesh Now the mesh is Change “Fill With” to ready for “poly-hexcore”, proceeding to the keep everything else solution mode default and click “Generate the Volume Mesh” Here mesh statistics can be seen, such as cell count, quality…etc Mesh Another view of the generated mesh Notice the inflation layers at the walls Save the project then click on “Switch to Solution” to go to Fluent solver Simulation (Solution Mode) Select “Transient” Input - 9.81 for gravity in the Y direction Add “Water-liquid” as a new material similar to previous tutorial. But this time keep “Air” because we will need both phases Solution mode of Fluent with meshing is exactly similar to Fluent without meshing from Previous tutorial Simulation (Multiphase VOF Model) Double-click on “Multiphase” in the “Models” Choose “Volume of Fluid” Formulation: “Explicit” Check “Implicit Body Force” and “Interfacial Ansi-Diffusion” Keep “Number of Eulerian Phases” as 2 (air and water) Keep everything else default Click “Apply” Simulation (Multiphase VOF Model) Click on “phases” button Rename primary phase as “Air” and make sure “Phase material” is set to air, click “Apply” Similarly, rename secondary phase as “water” and make sure phase material is set to “water-liquid” , click “Apply” Click on “Phase Interaction” button Choose “Constant” for “Surface Tension Coefficient” and input a value of 0.072 N/m Check “Surface Tension Force Modeling” Choose “Continuum Surface Force” And Check “Wall Adhesion” Simulation (Solution Methods) Make sure these options are chosen Double-click on “Methods” under “Solution” Simulation (Initialization) Go to “Initialization” Choose “Standard Initialization” Compute from “all-zones” Set “Turbulent Kinetic Energy” to 0.01 Set “Turbulent Dissipation Rate” to 100 Make sure “water Volume Fraction” is set to 0 (we will initialize the fluid domain with only air, and then patch a water column in a certain volume of the domain) Click “Initialize” Simulation (Dynamic Mesh Adaption) Click “Automatic” Automatic Mesh Adaption window will popup Go to “Predefined Criteria”, then “Multiphase” and choose “Volume of Fluid” Simulation (Dynamic Mesh Adaption) Choose “gradient_vof_refinement” from the dropdown menu of “Refinement Criterion” Set “Frequency (time-step) to 1 It should look like that Then click “OK” Click on “Controls” Set “Maximum Refinement Level to 2 Keep everything else default and click “OK” Simulation (Patching the water column) We need to define a region (create registry) for the zone where we want to patch the water column. Click “Manual” then “Cell Registers” then “New” then “Region” Add these coordinates then click “Save/Display” The region where the water column will be patched will can be seen. Note: Patching is not done yet Simulation (Patching the water column) Name the plane Choose “XY Plane” Z = 0.1 m Click “Create” Create “symmetry plane”: go to “General” then “Display” then “New Surface” and choose “Plane” Simulation (Patching the water column) Go to “Initialization” then “Patch” For “Phase” choose “water” “Volume Fraction” is selected already as the “Variable Put 1 for the “Value” Choose “region_water_column” for “Registers to Patch” Simulation (Patching the water column) To visualise the air and water regions, let us create a contour of VOF Go to “Results” then double-click “Contours”, in the popup window, choose options as shown and click “Save/Display” Simulation (Creating iso-surface for the air- water interface) Go to “General” then “Display” then “New Surface” and choose “Iso-Surface” Choose options as shown and click “Create” Simulation (Preparing Animation) Create a visualization for the mesh of the tank walls except the front wall, upper wall and vof-interface Simulation (Preparing Animation) Create a contour for the iso- surface Click on “Colormap Options” and uncheck “Show Colormap” Then click “Apply” then “Save/Display” Simulation (Preparing Animation) Now lets combine the mesh surface and iso-surface contours created in the previous 2 slides by generating a “Scene” Double-click on “Scene” Check “contour-iso” and “mesh- tank” Click “Save & Display” Simulation (Preparing Animation) Create animation before running the calculation for this case Simulation (Run the case) Set “Number of Time Steps” to 1000 “Time Step Size” to 0.01 s Keep everything else default Click “calculate” Notice the convergence during Simulation (Post-Processing) Coarse mesh No inflation Simulation (Post-Processing) Fine mesh With inflation