Module 14 Propulsion PDF
Document Details
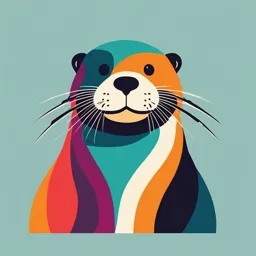
Uploaded by AffirmativeBougainvillea
Tags
Summary
This document provides a detailed overview of different types of jet engines, including turbine engines, ramjets, pulsejets, and rocket engines. It describes their operational principles, constructional arrangements, and thermodynamic cycles. The text includes historical context and details classifications and key characteristics of each engine.
Full Transcript
MODULE 14 PROPULSION Licence category B2 Issue number 2 14.1 (a): Engines - Turbine engines 14.1 (b): Engines - Auxiliary powe...
MODULE 14 PROPULSION Licence category B2 Issue number 2 14.1 (a): Engines - Turbine engines 14.1 (b): Engines - Auxiliary power units (APU) 14.1 (c): Engines - Piston engines 14.1 (d): Engines - Electric and hybrid engines 14.1 (e): Engines - Engine control 14.2: Electric/electronic engine indication systems 14.3: Propeller systems 14.4: Starting and ignition systems MODULE 14 PROPULSION Licence category B2 14.1 (a) Engines – Turbine engines Engines – Turbine engines Certification statement and objectives These Study Notes comply with the syllabus of EASA Regulation (EU) No. 1321/2014 Annex III (Part-66) Appendix I, including the amendment Regulation (EU) 2023/989, and the associated Knowledge Levels as specified below: Knowledge Part-66 Objective Levels Ref. B2 Engines 14.1 Turbine engines (a) 1 Constructional arrangement and operation of turbojet, turbofan, turboshaft and turboprop engines; 1 (a)-2 FOR TRAINING PURPOSE ONLY Issue 2 – June 2024 Engines – Turbine engines Table of contents (a) _____________________________________________ 4 Introduction ___________________________________ 4 Jet engine classification _________________________ 6 The rocket____________________________________ 6 The ramjet ___________________________________ 6 The pulsejet __________________________________ 8 The scramjet _________________________________ 10 Gas-turbine engines ___________________________ 10 Gas-turbine engine arrangements ________________ 12 The turbojet engine arrangement _________________ 14 The turboprop engine arrangement _______________ 18 The turboshaft engine arrangement _______________ 20 The turbofan engine arrangement ________________ 22 Operation − thermodynamic cycles _______________ 24 The Otto cycle _______________________________ 24 The Brayton cycle _____________________________ 26 Constructional arrangements summary ___________ 32 Turbojet engine arrangement ____________________ 33 Turbofan engine arrangement ___________________ 34 Turboprop engine arrangements _________________ 35 1 (a)-3 FOR TRAINING PURPOSE ONLY Issue 2 – June 2024 Engines – Turbine engines (a) Introduction The history of gas-turbine engines goes back to 150 BC when At the end of February 1937, the He S-1 turbojet engine with a Greek philosopher and mathematician, Hero, invented a toy hydrogen fuel was tested, producing a thrust of 250 pounds at that rotated by expelling steam from boiling water. This caused 10,000 RPM. So, impressed with the engine tests, Heinkel a reaction effect by the hot air steam from several nozzles pressed for an accelerated flight engine program. arranged on a wheel. Von Ohain’s team began development on the S-3 engine. One Then, in 1500, Leonardo da Vinci sketched a device that used of the main hurdles they faced was the development of a liquid hot air, which came from a fire and rose upwards to pass fuel combustor. This resulted in the engine shown below with a through a series of fan-like blades that turned a roasting spit. front combustor. Detailed design began in early 1938 on the test aircraft, the Heinkel He-178. On August 27, 1939, it made Steam turbines convert hot steam (from evaporated water) into the first successful flight of a jet power aircraft. mechanical useful work. The first practical steam turbine was made in the 1550s and has been used ever since to power, for In 1930, Sir Frank Whittle patented a design for a gas-turbine instance, ships and vessels, industrial powerplant and engine for jet propulsion in England, which was granted in electrical generators. The first modern steam turbine was made 1932. The first real run of the experimental engine (shown in England in the 1880s to power a dynamo. below) was in April of 1937. This engine had a centrifugal compressor and axial flow turbine. Dr Hans-Joachim Pabst von Ohain and Sir Frank Whittle are recognised as the co-inventors of the jet engine. Each was The experimental engine’s first successful and practical working separately and knew nothing of the other's works. outcome, the W.1 turbojet, was in May 1941, when powering Although Whittle started first, von Ohain was the first to design the first flight of the Gloster E28/39 test aircraft. and develop a turbojet engine to power an aircraft. Professor Dr Herbert Wagner started the design of the world’s Hans von Ohain started developing the turbojet engine in the first axial gas-turbine at Junkers, Magdeburg, in 1935. He early 1930’s. By 1935, he had developed a test engine (shown oversaw advanced developments at Junkers until 1939. below). He asked Ernst Heinkel, – an aircraft manufacturer, for Anselm Franz continued its development during the war years, support rather than approach the German engine industry. which led to the Jumo 004 axial gas-turbine engine used to Heinkel saw the promise in von Ohain’s invention – a means to power the Messerschmitt ME-262. Wagner is also the father of make the fastest aeroplane in the world. the turboprop engine, which he patented in 1935. 1 (a)-4 FOR TRAINING PURPOSE ONLY Issue 2 – June 2024 Engines – Turbine engines Gas-turbine pioneers 1 (a)-5 FOR TRAINING PURPOSE ONLY Issue 2 – June 2024 Engines – Turbine engines Jet engine classification There are four main types of jet engines: The ramjet The athodyd (aero-thermodynamic duct), ramjet or stovepipe the rocket engine; jet, is the simplest form of all power plants that use the the ramjet; atmosphere to sustain combustion; it has no moving parts and the pulsejet; and is very reliable. the gas-turbine engine. A ramjet uses the engine’s forward motion to compress They are all propelled forward by the rearward emission of a incoming air without a rotary compressor. Ramjets, therefore, gaseous fluid. All devices, except the rocket engine, are air- require forward motion through the air to produce thrust. Fuel breathing engines, meaning they use atmospheric air to is supplied via nozzles to the compressed air, and the mixture sustain combustion. is ignited. The combustion expands the gases, leaving the exhaust duct at a greatly increased speed. The increased The rocket airspeed inside the engine results in a forward thrust. The rocket is a non-air-breathing engine. This means it does not use atmospheric air to support combustion but carries its Ramjets require high forward speed to operate well, working oxidiser and fuel in solid or liquid form. This important property most efficiently at speeds around Mach 3 and can operate up allows the rocket to operate outside the Earth’s atmosphere. to at least Mach 5. Combustion transforms solids or liquids of small volume into Ramjets can be particularly useful in applications requiring a gases of large volume. The gases released by fuel and oxidiser small, simple engine for high-speed use, such as missiles. combustion escape through an exhaust nozzle at an extremely They have also been used successfully, though not efficiently, high velocity. as tip jets on helicopter rotors. The thrust reaction from the exhaust gases drives the rocket at Ramjets should not be confused with pulsejets, which use very high supersonic speeds, enabling it to free itself from the intermittent combustion. Ramjets employ a continuous Earth’s gravitational pull and fly into orbit. The high mass flow combustion process and are a distinct jet engine type. rate is essential for such speeds to be reached. The first rocket to travel into space and the first long-range ballistic missile was designed by Wernher von Braun in 1944. It was named the V2, capable of 5,760 kmph/3,580 mph and carrying a payload of 1,000 kg of high explosives. 1 (a)-6 FOR TRAINING PURPOSE ONLY Issue 2 – June 2024 Engines – Turbine engines 1 (a)-7 FOR TRAINING PURPOSE ONLY Issue 2 – June 2024 Engines – Turbine engines The pulsejet The pulsejet is constructed in the same way as the ramjet. Pulse jets were used to power the German V1 flying bombs However, it has additional spring-loaded valves in the air during WW2. Since then, no commercial pulse jet engine has intake. The pulsejet is an air-breathing jet engine without a been put into production. compressor or turbine, in which intermittent combustion provides the thrust. The low complexity makes it ideal for low-cost projects. However, pulse jets are very noisy and have no civil A typical pulsejet comprises an air intake fitted with one-way application. During WW2, the sound from these jets led to them valves, a combustion chamber, and an acoustically resonant being nicknamed ‘Doodlebugs’; the noise of a V1 scared exhaust pipe. people a lot. This jet type is only used for experimental or hobby do-it-yourself projects. In the simplest form, the intake valving is accomplished using check valves (see figure). Fuel is mixed with the air in the intake or injected into the combustion chamber. Starting the engine usually requires forced air and an ignition method, such as a spark plug, for the fuel-air mix. Once running, the engine only requires an input of fuel to maintain a self-sustaining combustion cycle. The pulsejet combustion cycle comprises six phases: (1) ignition, (2) combustion, (3) exhaust, (4) induction, (5) compression, and (6) fuel injection (in some engines). Starting with ignition “ON” in the combustion chamber, a high pressure is created by the combustion of the fuel-air mixture. The pressurised gas from combustion cannot exit forward through the intake check valve and, therefore, escapes to the rear through the exhaust tube. When the gases leave the combustion chamber, a slight underpressure is created, opening the inlet check valves and allowing atmospheric air to flow into the engine. The pulse jet engine has the potential to be both light and powerful. It can operate from a standstill (static condition) up to supersonic speeds. 1 (a)-8 FOR TRAINING PURPOSE ONLY Issue 2 – June 2024 Engines – Turbine engines German V1 Flying bomb 1 (a)-9 FOR TRAINING PURPOSE ONLY Issue 2 – June 2024 Engines – Turbine engines The scramjet Gas-turbineengines A scramjet is a variation of a ramjet but has a supersonic The gas-turbine engine is far more complicated than the combustion. At higher speeds, it is necessary to combust pulsejet and ramjet. The gas-turbine engine principle can be supersonically to maximise the efficiency of the combustion used for propulsion in several ways. As well as aviation use, process. Ramjets always slow the incoming air to a subsonic gas-turbines also have industrial and naval use as power velocity within the combustor. This deceleration is an essential plants. However, we only focus on the aero-type gas-turbine operating condition. Scramjets, or ‘supersonic combustion engine in the following. ramjets’, are similar to ramjets, but the air goes through the entire engine at supersonic speeds, eliminating the creation of a strong shock wave in the intake. This increases the stagnation pressure recovered from the free stream and improves net thrust. Because of the hypersonic (rather than supersonic) flight speeds experienced and needed, scramjet air intake temperatures are too high for burning kerosene, so hydrogen is usually used as fuel. Fuel injection is often into a sheltered region below a step in the combustor wall. The engine must have a minimum functional speed of Mach 7 to 8. The engine accelerates from this speed to between Mach 12 and Mach 24 (orbital velocity). For example, a NASA aircraft X-43 (scramjet) is launched from a B52 aircraft on the nose of a rocket for initial acceleration and launched from the rocket once functional speed is reached. Although scramjet engines have been studied for many decades, it is only recently that small experimental units have been flight tested and then only very briefly. However, Scramjets will likely be used in future supersonic transport (SST) aircraft. 1 (a)-10 FOR TRAINING PURPOSE ONLY Issue 2 – June 2024 Engines – Turbine engines Jet engine classification – ramjets and scram jets 1 (a)-11 FOR TRAINING PURPOSE ONLY Issue 2 – June 2024 Engines – Turbine engines Gas-turbine engine arrangements According to their task, different types of gas-turbine aero- A gas-turbine engine can be designed to produce the engines exist. A distinction is made concerning design propulsive force (thrust) by using two main principles: characteristics such as the number of spools (single, dual or triple spooled), compression principles, airflow distribution expanding hot gases through a nozzle, the jet principle; within the engine, and utilisation of the exhaust gas. or by converting the hot gases into mechanical shaft There are four main types of gas-turbine engines used in horsepower (SHP), driving a propeller or a rotor system aircraft: that converts power into thrust. the turbojet or straight jets; Turboprops often use a combination of the two principles. the turbofan (low, medium, high, and ultra-high bypass); Turbojets and turbofans are similar in design. A turbojet has the turboprop (free turbine and fixed shaft); and only one gas path, whereas the turbofan has two, divided into the turboshaft. a cold secondary (bypass) airflow and a hot primary (core) airflow. Though all four main types have distinctive features, each includes the same essential components: Shafts connect the turbine to the compressor. Single-spool engines have only one shaft. As their names imply, twin-spool (1) an air inlet; or triple-spool engines have separate shafts where separate (2) a compressor section; turbine sections turn separate compressors. Often, turboprops (3) a combustion section; and turboshafts have additional turbines driving the load. This (4) a turbine section; and separate turbine is called a ‘free power turbine’. A reduction (5) an exhaust section. gearbox is installed between the engine and the consumer, usually integrated into the engine. Each gas-turbine engine section has a dedicated purpose, unlike a piston engine, which uses the cylinders for all work Another distinctive difference between the four main types is processes. how the device is rated and the dimension of the functional outcome, which is either thrust (T) or power (P). The exact gas condition at the exhaust section (5) depends on how much energy the turbine section has extracted. When the Turbojets and turbofans are reaction-type engines and pure exhaust gas generates propulsive force, the exhaust section is thrust producers rated in Newtons or pounds. Turboprop and called a nozzle or jet pipe; when it only expels hot gases, it is turboshaft engines are essentially shaft horsepower (SHP) called an exhaust pipe or tailpipe. producers. SHP is later converted into thrust by either a propeller or rotor system. 1 (a)-12 FOR TRAINING PURPOSE ONLY Issue 2 – June 2024 Engines – Turbine engines Basic gas-turbine engine arrangements 1 (a)-13 FOR TRAINING PURPOSE ONLY Issue 2 – June 2024 Engines – Turbine engines The turbojet engine arrangement Turbojet engines (and turbofan engines) provide propulsive The combustion process leads to a step increase in forces directly by ‘reaction forces’ generated by the hot exhaust temperature, whereas the pressure remains virtually constant. gases. The flame temperature in the combustion chamber reaches up to 2,300 K and can, at the inlet to the first turbine stage, be as Turbojet engines, or straight jets as they are often called, high as 1,900 K. In the combustion chamber, the airflow takes allow all the air that enters the air inlet to flow through the the characteristics of a gas useful for gas-turbine operation, i.e., engine compressor(s) and hot section. The turbine wheel(s) to produce mechanical work efficiently. extract the power, SHP, needed for compressor operation. The remaining hot gases are then expanded through a nozzle and The first section where energy is extracted from the gas is in are converted into a high-speed air stream. This is the pure jet the ‘gas-turbine’, which gave this engine type its name. principle, also called the Whittle principle. A turbine is a complementary part to the compressor, to which To function correctly and produce thrust, an air intake and an it is rigidly linked by a hollow shaft (or spool). exhaust system are required to process the airflow. The turbine can have one or more stages and be divided into The air first enters the air intake section, which must deliver a different sections driving separate compressor sections (multi- smooth and uniform air stream to the compressor inlet. The spool engine). The energy content of the gas is not depleted compressor is a mechanical device, a fast-rotating air pump when the gas discharges from the turbine section. The whose task is to increase the air pressure. The resultant energy compressor, the combusting chamber and the turbine transfer leads to a rise in pressure, density, and temperature. combined have processed the airflow such that the hot gas can do work; therefore, these three units together are called a gas When discharged from the compressor, the air enters the generator, regardless of the type of turbine engine. combustion section via a diffuser duct. In the combustion section, fuel is injected and burned, thus adding energy to the In a turbojet, a large part of the heat and pressure is still airflow. A chemical reaction achieves the energy transfer. A available after the turbine section to be converted into kinetic continuous combustion process is achieved, and ignition is only energy. The converging exhaust nozzle accomplishes the required during the initial start phase. conversion of heat and pressure into velocity. High exit velocity is a prerequisite to the generation of thrust. 1 (a)-14 FOR TRAINING PURPOSE ONLY Issue 2 – June 2024 Engines – Turbine engines 1 (a)-15 FOR TRAINING PURPOSE ONLY Issue 2 – June 2024 Engines – Turbine engines The exhaust velocity may be further increased by ‘afterburning’ (reheating) the gas downstream the turbine section, however, with a significant negative impact on fuel efficiency. A quality of the turbojet is its ability to produce exhaust gases with very high velocities, which is needed for supersonic speed. The turbojet has been outdated by the much more fuel-efficient turbofan engine, but this does not mean that the turbojet cannot be economical in use. It just requires the flight speed to be sufficiently high, e.g. M 2.0, for most of the flight, which was the case for Concorde and many high-altitude intercepting military fighters during the Cold War, like the F104. However, modern multi-role military fighters must be able to cruise at subsonic and supersonic Mach numbers. Hence, the turbofan engine is the best overall option. 1 (a)-16 FOR TRAINING PURPOSE ONLY Issue 2 – June 2024 Engines – Turbine engines 1 (a)-17 FOR TRAINING PURPOSE ONLY Issue 2 – June 2024 Engines – Turbine engines The turboprop engine arrangement A basic turboprop engine consists of an air inlet section, The fact that the exhaust airflow has low speed and low compressor section, combustion section, turbine section, temperature makes the turboprop economically superior to exhaust, reduction gearbox and propeller. similar-sized turbojets and turbofans, simply because turboprops are more energy efficient. But then one must recall The objective is to extract a maximum of heat energy from the that if one needs to fly fast, with high subsonic or supersonic flow of the hot combustion gases and to use them to drive the speeds, it indeed has a price. propeller. There are many distinctive differences between turbojets and This can be achieved through larger turbines or additional turboprops (and turboshafts) when it comes to details. Some turbine stages. Turboprops and turboshafts usually have a are: reverse-flow combustion chamber to make the engine as short and compact as possible. the fuel regulation principles; the air intake; The propeller can be driven by the same main shaft that drives the exhaust nozzle; the engine compressor, a coupled or compounded engine. the thrust or power setting parameters displayed for the pilot; and An additional but separate turbine section often provides the cockpit control handles, thrust levers, power, propeller, power to drive the propeller. Because this turbine turns free of and condition levers. the gas generator turbine, it is called a free-power turbine or a power turbine. The Pratt & Whitney PT-6 turboprop engine Thrust reverse can be provided by many turboprops simply by below has a free-power turbine. turning the propeller blades to a negative pitch, reversing the propulsion force. This effectively reduces the load on the wheel Typical for the turboprop is the utilisation and integration of a brakes during retardation (braking) after landing. reduction gear, lowering the speed of the output shaft driving the load. This tends to complicate such engine designs. The residual jet thrust of a turboprop engine is only 10 – 15% of a turbojet engine of similar size. The nozzle, therefore, was replaced by an exhaust pipe. 1 (a)-18 FOR TRAINING PURPOSE ONLY Issue 2 – June 2024 Engines – Turbine engines A free power turbine turboprop engine A coupled (or compounded) turboprop engine Turboprop engines 1 (a)-19 FOR TRAINING PURPOSE ONLY Issue 2 – June 2024 Engines – Turbine engines The turboshaft engine arrangement The turboshaft is similar to the turbojet. Most of the usable hot gas energy is extracted and converted into SHP driving anything other than propellers. Turboshaft engines will typically not produce any nozzle thrust. This engine type is used by turbine-engine-powered helicopters. However, it is similarly employed in auxiliary power units (APU) to provide electricity and pneumatic power for commercial jet aircraft during ground operation and as an in-flight backup power source. Also, the ‘load’ can be driven by the engine’s main shaft (coupled type) or a free-power turbine. The turboshaft engine shown below, the Turbomeca Ariel, has a free-power turbine. The Allied Signal APU shown has a single main shaft with a gas generator section (power section) at one end and a power extracting load section at the other. The load section consists of a load compressor with an adjustable air supply to the aircraft’s pneumatic system. A starter generator provides 115 V AC 400 Hz for the aircraft’s electrical system. 1 (a)-20 FOR TRAINING PURPOSE ONLY Issue 2 – June 2024 Engines – Turbine engines Turboshaft engines 1 (a)-21 FOR TRAINING PURPOSE ONLY Issue 2 – June 2024 Engines – Turbine engines The turbofan engine arrangement Turbofan (TF) engines are classified according to the portion of After the turbine sections, a nozzle converts the remaining the mass flow bypassed around the basic gas generator, also pressure energy in the core airflow into velocity. When the fan called the core engine. has a separate nozzle, the engine is said to be ‘unmixed’; if the two nozzles are combined, the engine is said to be ‘mixed’. Turbofan engines are also called bypass engines. They are available at many different bypass ratios (BPR), depending on Instead of the mixed and unmixed classifications, turbofan the engine generation and usage. The bypass ratio and its engines are also referred to as ‘short ducted’ or ‘long ducted’. advantages are explained more thoroughly in Module 15.2, Engine Performance. Turbofans are today used by commercial jets cruising with high-subsonic Mach numbers and military jets cruising below The turbofan can crudely be regarded as a mixture of a turbojet or above the sonic speed. and a turboprop, where the best qualities of each have been utilised. This engine type literally handles a hot air mass and a Commercial turbofans are capable of tremendously high thrust cold air mass. production, from a few thousand pounds of thrust up to more than 100,000 lbs of thrust. The bypass airflow passes through the fan (consisting of one or more stages) and does not participate in the combustion The fan airflow can on most commercial turbofans be ‘reversed’ process; therefore, it remains ‘cold’. This airflow can be by the thrust reverser, called a cold steam reverser or fan regarded as propeller airflow. The fan section handles the cold reverser. Some engines also feature ‘hot stream reversers’, air mass, accelerates it and expels it through a separate nozzle reversing hot and cold air streams. or mixes it with the hot gases from the engine core and expels these through a common (or combined) nozzle. The bypass The figure below shows the principal schematic of a ‘cold airflow is often referred to as the ‘secondary airflow’. stream/fan reverser’ through blocker doors. Cascades, or guide vanes, make sure that the airflow is directed in the proper The core airflow passes through the engine compressor(s), direction. takes part in the combustion process and delivers part of its energy content to separate turbine sections. The turbines drive The percentage thrust distribution between the fan and core the compressor(s) and the fan. thrust primarily depends on the bypass ratio. The fan drive may be part of the low-pressure compressor drive In modern commercial applications (e.g. CFM56, IAE V2500, or have a separate turbine section (like a free-power turbine). RR Trent, PW4084, GE CF-6 and GE90), the fan typically The core airflow is sometimes referred to as the ‘primary delivers between 75% and 85% of the total thrust. airflow’. 1 (a)-22 FOR TRAINING PURPOSE ONLY Issue 2 – June 2024 Engines – Turbine engines Turbofan engine (commercial use) 1 (a)-23 FOR TRAINING PURPOSE ONLY Issue 2 – June 2024 Engines – Turbine engines Operation − thermodynamic cycles Heat engines run on a specific thermodynamic cycle. Heat C to D engines are often named after the thermodynamic cycle they Expansion (power) stroke – adiabatic expansion of the hot are modelled by. gases in the cylinder. A cycle describes what happens to air as it passes in, through, D to A and out of the turbine. The cycle usually describes the Exhaust stroke – ejection of the spent, hot gases. relationship between the space occupied by the air in the system (volume, V) and the pressure (P) it is under. By drawing Induction stroke – intake of the next air charge into the a graph with related pressure (P) and volume (V), the total work cylinder. The volume of exhaust gases is the same as the air (W) of a heat engine can be estimated. The total work involved charge. with the process is proportional to the total area of the PV diagram. A cycle can also be expressed in a pressure- temperature (PT) diagram. The Otto cycle Usually, piston engines operate as an ‘Otto Cycle’ (after Nikolaus Otto). This cycle is also known as a ‘constant volume’ cycle (or process). Constant volume refers to how the combustion appears. The Otto cycle is the standard open cycle used in the four- stroke petrol (gasoline) fuelled internal combustion engine using spark ignition. The Otto cycle uses the following processes: A to B Compression stroke – adiabatic compression of air/fuel mixture in the cylinder B to C Ignition of the compressed air/fuel mixture at the top of the compression stroke while the volume is essentially constant. 1 (a)-24 FOR TRAINING PURPOSE ONLY Issue 2 – June 2024 Engines – Turbine engines Otto heat cycle PV diagram The gasoline internal combustion engine works on the Otto cycle 1 (a)-25 FOR TRAINING PURPOSE ONLY Issue 2 – June 2024 Engines – Turbine engines The Brayton cycle Also known as the ‘gas-turbine cycle’. This process is known as heating at constant pressure. Under these conditions, no peak or fluctuating pressures are to be The gas-turbine engine is essentially a heat engine using air as withstood, as is the case with the piston engine with its peak a working fluid to provide thrust. To achieve this, the air passing pressures of more than 1,000 lb/in2. These peak pressures through the engine must be accelerated; this means that the make it necessary for the piston engine to employ cylinders of velocity or kinetic energy of the air is increased. To obtain this heavy construction and working cycle and airflow to use high- increase, the pressure energy is first increased, followed by the octane fuels, in contrast to the low-octane fuels and the light addition of heat energy before final conversion back to kinetic fabricated combustion chambers used on the turbine engine. Energy in the form of a high-velocity jet efflux. The working cycle of the gas-turbine engine is similar to that of the four-stroke piston engine. However, combustion occurs at a constant pressure in the gas-turbine engine. In contrast, in the piston engine, it occurs at a constant volume. Both engine cycles show induction, compression, combustion and exhaust. These processes are intermittent in the case of the piston engine, whilst they occur continuously in the gas-turbine. In the piston engine, only one stroke is utilised in producing power, the others being involved in the charging, compressing and exhausting of the working fluid. In contrast, the turbine engine eliminates the three idle strokes, thus enabling more fuel to be burnt in a shorter time, producing a greater power output for a given engine size. Due to the continuous action of the turbine engine and the fact that the combustion chamber is not an enclosed space, the pressure of the air does not rise, like that of the piston engine, during combustion, but its volume does increase. 1 (a)-26 FOR TRAINING PURPOSE ONLY Issue 2 – June 2024 Engines – Turbine engines Comparison of turbine engine with piston engine 1 (a)-27 FOR TRAINING PURPOSE ONLY Issue 2 – June 2024 Engines – Turbine engines The ‘Brayton cycle’ is the basis for the operation of a gas- turbine and can be used to approximate the cycle of all such units. The Brayton cycle is a ‘constant pressure’ cycle because the combustion process occurs at near-constant pressure. The Brayton cycle can be open to the atmospheric air or sealed and closed off to the outside (called open or closed cycles). Industrial gas-turbines are sometimes using a closed cycle with regenerative heat exchangers. Aviation gas-turbine engines are open-cycled, which means fresh gas is drawn into the compressor and the products are exhausted from the turbine and not reused. A typical ideal gas- turbine cycle is illustrated below. WIN and WOUT express, respectively, if work is applied or extracted. The compressor(s) uses work in the compression process. The turbine(s) extract power from the hot gas, which is used to run the compressor via a connecting shaft. The residual energy in the gas is the available ‘useful’ work. In a thrust-producing jet engine, the residual work is expended in the exhaust nozzle, converting pressure into velocity. The residual work can also be extracted by a larger or free-power turbine to turn a generator, a ship’s propeller, an aircraft propeller, or a helicopter rotor. 1 (a)-28 FOR TRAINING PURPOSE ONLY Issue 2 – June 2024 Engines – Turbine engines Brayton heat cycle PV diagram 1 (a)-29 FOR TRAINING PURPOSE ONLY Issue 2 – June 2024 Engines – Turbine engines Brayton cycle 1 (a)-30 FOR TRAINING PURPOSE ONLY Issue 2 – June 2024 Engines – Turbine engines “THIS PAGE INTENTIONALLY LEFT BLANK” 1 (a)-31 FOR TRAINING PURPOSE ONLY Issue 2 – June 2024 Engines – Turbine engines Constructional arrangements summary Examples of gas-turbine configurations: (1) turbojet, (2) turboprop, (3) turboshaft (electric generator), (4) high-bypass turbofan, (5) low-bypass afterburning turbofan 1 (a)-32 FOR TRAINING PURPOSE ONLY Issue 2 – June 2024 Engines – Turbine engines Turbojet engine arrangement Pure turbojet engine 1 (a)-33 FOR TRAINING PURPOSE ONLY Issue 2 – June 2024 Engines – Turbine engines Turbofan engine arrangement Fan engine 1 (a)-34 FOR TRAINING PURPOSE ONLY Issue 2 – June 2024 Engines – Turbine engines Turboprop engine arrangements 1 (a)-35 FOR TRAINING PURPOSE ONLY Issue 2 – June 2024 Engines – Turbine engines “THIS PAGE INTENTIONALLY LEFT BLANK” 1 (a)-36 FOR TRAINING PURPOSE ONLY Issue 2 – June 2024 MODULE 14 PROPULSION Licence category B2 14.1 (b) Engines – Auxiliary power units (APU) Engines – Auxiliary power units (APU) Certification statement and objectives These Study Notes comply with the syllabus of EASA Regulation (EU) No. 1321/2014 Annex III (Part-66) Appendix I, including the amendment Regulation (EU) 2023/989, and the associated Knowledge Levels as specified below: Knowledge Part-66 Objective Levels Ref. B2 Engines 14.1 Auxiliary power units (b) 1 Constructional arrangement and operation of auxiliary power units (APU); 1 (b)-2 FOR TRAINING PURPOSE ONLY Issue 2 – June 2024 Engines – Auxiliary power units (APU) Table of contents (b) _____________________________________________ 4 Introduction ___________________________________ 4 APU general arrangements _______________________ 6 Example system − Airbus A330/Garrett GTCP331-350 12 Power section ________________________________ 14 Engine compressor____________________________ 14 Combustion chamber __________________________ 14 Turbine _____________________________________ 14 Load compressor _____________________________ 14 Inlet guide vanes assembly _____________________ 14 Load compressor impeller ______________________ 14 Load compressor diffuser _______________________ 14 Accessory drive gearbox _______________________ 16 Mounts system _______________________________ 16 Exhaust _____________________________________ 22 1 (b)-3 FOR TRAINING PURPOSE ONLY Issue 2 – June 2024 Engines – Auxiliary power units (APU) (b) Introduction The auxiliary power unit, or APU as it is commonly known, is a small gas-turbine engine, as shown below, fitted to aircraft to provide: electric power from shaft-driven generators; pneumatic duct pressure for air conditioning; and engine starting purposes. It is called an auxiliary power unit since it is not the primary power source for the aircraft and is mainly used on the ground when the aircraft engines are not running. The APU provides the above two services but can also, on certain occasions, be used in the air. 1 (b)-4 FOR TRAINING PURPOSE ONLY Issue 2 – June 2024 Engines – Auxiliary power units (APU) APU components (B737) 1 (b)-5 FOR TRAINING PURPOSE ONLY Issue 2 – June 2024 Engines – Auxiliary power units (APU) APU general arrangements The basic arrangement of the APU is shown below. Here, we have a small turbine engine, known as the power section, driving a load compressor to produce pneumatic power. The load compressor also drives the accessory gearbox containing the electrical generator. Consider the schematic diagram of an APU below. The layout is similar to a basic gas-turbine engine. This configuration shows air is taken from the compressor when pneumatic power is required. Although such an APU layout is acceptable on smaller aircraft where pneumatic power demand is small, it was found to be unacceptable on larger aircraft as the air being drawn from the compressor for pneumatic purposes reduces the air going to the turbines for cooling purposes. This reduction of cooling air leads to a reduction in the life of the turbine. 1 (b)-6 FOR TRAINING PURPOSE ONLY Issue 2 – June 2024 Engines – Auxiliary power units (APU) APU schematic 1 (b)-7 FOR TRAINING PURPOSE ONLY Issue 2 – June 2024 Engines – Auxiliary power units (APU) On later models of APU, this problem has been eliminated by including a load compressor. In this configuration, the inlet air is directed into the load compressor and the power section compressor. The load compressor now satisfies all pneumatic loading requirements without extracting air from the power section. The figure below represents a typical cross-section of an APU with a load compressor. As you can see, the power section with two centrifugal compressor stages drives a centrifugal load compressor, producing pneumatic pressure when a demand is made on the system. 1 (b)-8 FOR TRAINING PURPOSE ONLY Issue 2 – June 2024 Engines – Auxiliary power units (APU) APU arrangement with load compressor 1 (b)-9 FOR TRAINING PURPOSE ONLY Issue 2 – June 2024 Engines – Auxiliary power units (APU) The manufacturer’s requirements generally dictate the location of the APU on the aircraft. Because of the noise factor and the problem of hot exhaust gasses, it is located as far away from ground servicing areas as possible. The usual place for it to be fitted is in the tail section of the aircraft; however, this may be impracticable due to the location of a tail-mounted engine. The APU may be fitted into landing gear bays or wing structures on some aircraft. Wherever the APU is located, ducting will be required to bring the air to the APU inlet and to vent exhaust gasses overboard. 1 (b)-10 FOR TRAINING PURPOSE ONLY Issue 2 – June 2024 Engines – Auxiliary power units (APU) APU arrangement with load compressor 1 (b)-11 FOR TRAINING PURPOSE ONLY Issue 2 – June 2024 Engines – Auxiliary power units (APU) Example system − Airbus A330/Garrett GTCP331-350 The auxiliary power unit (APU) is in the unpressurised tail cone. The APU is designed to operate throughout the entire flight The APU is a self-contained unit, which lets the aircraft be envelope. Electrical power is available whenever the APU independent of external pneumatic and electrical power operates, but bleed air is limited by the demand and is shut off sources. The APU is mounted in a fireproof compartment in the above 25,000 ft. fuselage tail cone. Two access doors give access to the APU compartment for inspection and maintenance. The APU is The auxiliary power unit (APU) GTCP331-350 is divided into suspended in its compartment by shock-mounts. An exhaust different sub-systems, which are: duct releases the APU gas into the atmosphere. the engine system, including three basic modules: the The APU is a constant-speed gas-turbine engine. The power section, the load compressor, and the accessory constant-speed gas-turbine engine drives the accessory drive gearbox; gearbox and a load compressor. The APU provides: the APU mounts system; the air intake system; electrical power for the aircraft systems; the draining system; and bleed air for engine starting and air conditioning on the the exhaust system. ground; and bleed air for air conditioning/pressurisation and wing anti-ice in flight. The APU operation is controlled and monitored by the electronic control box (ECB). The ECB has full authority over the following APU functions: starting; acceleration; speed governing indication; fault monitoring; and interface with aircraft systems. 1 (b)-12 FOR TRAINING PURPOSE ONLY Issue 2 – June 2024 Engines – Auxiliary power units (APU) Airbus A330/Garrett GTCP331-350 1 (b)-13 FOR TRAINING PURPOSE ONLY Issue 2 – June 2024 Engines – Auxiliary power units (APU) Power section Turbine The power section of the GTCP331-350 system supplies the The turbine of the GTCP331-350 power section is of the three- shaft power to drive: stage axial design. The turbine rotors are the rear part of the main shaft. Their 100% operation speed is 41,730 RPM. the load compressor; the accessory drive gearbox; and Load compressor the electrical generator. The load compressor module supplies compressed air to the AIRCRAFT pneumatic system. It is installed between the power It is built up by the static structure and the rotating group. The section and the accessory drive gearbox. The load compressor static structure comprises the engine compressor case and the components are: combustion chamber. The rotating group, including the centrifugal compressor and turbine, is a single shaft assembly the inlet guide vanes (IGV) assembly; supported with a ball bearing at the FWD end and a roller the load compressor impeller; bearing at the aft end. Both bearings are designed with a the load compressor diffuser; and lubricated outer race to damp vibrations. Their 100% operation the scroll. speed is 41,730 RPM. An FWD roller bearing and an aft ball bearing support the Engine compressor rotating components of the load compressor. Both bearings are The engine compressor is a two-stage centrifugal compressor. designed with a lubricated outer race to damp vibrations. Each compressor stage has an impeller and a diffuser. The role of the engine centrifugal compressor is to increase and guide Inlet guide vanes assembly the air pressure through de-swirl vanes into the combustion The variable IGV assembly supplies the correct amount of air chamber. to the APU load compressor by modulating the vane position angles. It comprises IGVs on a cylindrical rack mechanically Combustion chamber connected to a fuel-operated actuator by an adjustable linkage The combustion chamber is reverse-flow annular and rod. surrounds the turbine hub containment. While the compressed air is in the combustion chamber, fuel is atomised and mixed Load compressor impeller into the air. During combustion, the expanded gas stream is The load compressor impeller is a single-stage impeller. directed to the turbine, changing its energy into rotational movement. Load compressor diffuser Downstream of the load compressor impeller is the radial diffuser. Multiple openings in the diffuser throat are used to collect the static pressure. 1 (b)-14 FOR TRAINING PURPOSE ONLY Issue 2 – June 2024 Engines – Auxiliary power units (APU) Airbus A330/Garrett GTCP331-350 1 (b)-15 FOR TRAINING PURPOSE ONLY Issue 2 – June 2024 Engines – Auxiliary power units (APU) Accessory drive gearbox The accessory drive gearbox is the forward APU module. It is connected directly to the load compressor module, which transmits the shaft power from the power section. The gearbox transmits the shaft power to the APU accessories and the APU electrical generator installed on the gearbox pads. It is also the oil reservoir for the APU oil system. The APU driven accessories are: the APU generator; and the lubrication module and the fuel control unit (FCU). Mounts system The APU is installed on a three-point vibration-insulated mounting system. This system comprises: seven structure brackets; seven rod assemblies; three APU mount brackets; three vibration isolators; and two bonding straps. 1 (b)-16 FOR TRAINING PURPOSE ONLY Issue 2 – June 2024 Engines – Auxiliary power units (APU) Airbus A330/Garrett GTCP331-350 – mounts system 1 (b)-17 FOR TRAINING PURPOSE ONLY Issue 2 – June 2024 Engines – Auxiliary power units (APU) Intake system The air intake system supplies ambient air to the plenum chamber of the APU. The main components of the air intake system are: the air intake diverter; the air intake assembly with an air intake nose, an air intake flap and an air intake flap actuator; and the air intake duct. The electronic control box (ECB) supplies the necessary electrical power to operate the air intake flap actuator. The two limit switches and the two position switches control the position of the air intake flap actuator. When the aircraft is on the ground with no electrical power available or in case of flap actuator failure, the air intake flap can be operated manually by a manual override device. 1 (b)-18 FOR TRAINING PURPOSE ONLY Issue 2 – June 2024 Engines – Auxiliary power units (APU) Airbus A330/Garrett GTCP331-350 – intake system 1 (b)-19 FOR TRAINING PURPOSE ONLY Issue 2 – June 2024 Engines – Auxiliary power units (APU) Airbus A330/Garrett GTCP331-350 – intake system 1 (b)-20 FOR TRAINING PURPOSE ONLY Issue 2 – June 2024 Engines – Auxiliary power units (APU) Garrett GTCP331 AUP 1 (b)-21 FOR TRAINING PURPOSE ONLY Issue 2 – June 2024 Engines – Auxiliary power units (APU) Exhaust The APU exhaust system ducts the APU GTCP331-350 exhaust gases overboard; its secondary function is to decrease the exhaust noise level. The exhaust system is made of two main components: the exhaust muffler assembly; and the suspension. The exhaust muffler assembly directs the exhaust gas flow to the ambient air, reducing the exhaust noise. It is composed of a flexible connection, compensating for expansion and contraction between the APU and the exhaust muffler caused by changes in temperature. The exhaust muffler suspension has two guide rails, which are part of the exhaust muffler compartment structure. They have a U shape. On the exhaust muffler, there are four mount brackets. These fit into guide rails, allowing thermal expansion. The exhaust muffler has thermal insulation. It keeps the temperature at the external surface of the exhaust muffler to a limit of 200°C. 1 (b)-22 FOR TRAINING PURPOSE ONLY Issue 2 – June 2024 Engines – Auxiliary power units (APU) Airbus A330/Garrett GTCP331-350 – exhaust 1 (b)-23 FOR TRAINING PURPOSE ONLY Issue 2 – June 2024 Engines – Auxiliary power units (APU) "THIS PAGE INTENTIONALLY LEFT BLANK" 1 (b)-24 FOR TRAINING PURPOSE ONLY Issue 2 – June 2024 MODULE 14 PROPULSION Licence category B2 14.1 (c) Engines – Piston engines Engines – Piston engines Certification statement and objectives These Study Notes comply with the syllabus of EASA Regulation (EU) No. 1321/2014 Annex III (Part-66) Appendix I, including the amendment Regulation (EU) 2023/989, and the associated Knowledge Levels as specified below: Knowledge Part-66 Objective Levels Ref. B2 Engines 14.1 Piston engines (c) 1 Constructional arrangement and operation of piston engines; 1 (c)-2 FOR TRAINING PURPOSE ONLY Issue 2 – June 2024 Engines – Piston engines Table of contents (c) _____________________________________________ 4 Engine configurations __________________________ 44 Introduction ___________________________________ 4 General_____________________________________ 44 Type of engines _______________________________ 4 Inline engines ________________________________ 44 History of aero-gasoline engines __________________ 4 Opposed or O-type engines _____________________ 44 Examples of modern aero-gasoline engines ________ 12 V-type engines _______________________________ 46 History of Diesel aero engines ___________________ 14 X-type engines _______________________________ 46 Examples of modern aero-Diesel engines __________ 18 H-type engines _______________________________ 46 Piston engine world records _____________________ 20 Radial engines _______________________________ 50 Operating principles ___________________________ 22 Rotary engines _______________________________ 52 General _____________________________________ 22 Wankle engines ______________________________ 54 The 4-stroke cycle engine ______________________ 26 Firing order ___________________________________ 56 The Otto cycle _______________________________ 32 General_____________________________________ 56 The Diesel cycle ______________________________ 32 Radial engines _______________________________ 56 The 2-stroke cycle engine ______________________ 33 Inline and V-engines___________________________ 58 Diesel engines _______________________________ 34 Horizontally-opposed engines ___________________ 60 Engine displacement ___________________________ 36 Glossary of piston engine terms _________________ 62 Compression ratio _____________________________ 38 Efficiencies ___________________________________ 40 Mechanical efficiency __________________________ 40 Thermal efficiency ____________________________ 40 Volumetric efficiency___________________________ 42 1 (c)-3 FOR TRAINING PURPOSE ONLY Issue 2 – June 2024 Engines – Piston engines (c) Introduction Type of engines History of aero-gasoline engines Several methods can classify aircraft engines. They can be Pistons in cylinders first saw use in steam engines. Scotland’s classed by operating cycles, cylinder arrangement, or the James Watt crafted the first good ones during the 1770s. A method of thrust production. century later, the German inventors Nicolaus Otto and Gottlieb Daimler introduced gasoline as the fuel, burned directly within All are heat engines that convert fuel into heat energy, which is the cylinders. Such motors powered the earliest automobiles. converted to mechanical energy to produce thrust. Most current They were lighter, more mobile than steam engines, more aircraft engines are of the internal combustion type because the reliable, and easier to start. combustion process occurs inside the engine. Aircraft engines come in many different types: Some single-piston gasoline engines entered service, but for use with aeroplanes, most had several pistons, each shuttling reciprocating-piston; back and forth within its cylinder. Each piston also had a rotary; connecting rod, which pushed on a crank that was part of a two- or four-cycle; crankshaft. This crankshaft drove the propeller. spark-ignition; Diesel; and Engines built for aeroplanes had to produce plenty of power air- or water-cooled. while remaining light in weight. The first American aeroplane builders – Wilbur and Orville Wright, and Glenn Curtiss – used Reciprocating engines also have subdivisions based on the motors resembling car engines. They were heavy and complex type of cylinder arrangement. because they used water-filled plumbing to stay cool. Manufacturers have developed some designs that are used A French engine of 1908, the Gnome, introduced air cooling to more commonly than others and are, therefore, recognised as eliminate the plumbing and lighten the weight. It was known as ‘conventional’. Reciprocating engines may be classified a rotary engine. The Wright and Curtiss motors had been according to the cylinder arrangement, inline, V-type, radial, mounted firmly in supports, with the shaft and propeller and opposed, or the cooling method, liquid-cooled or air- spinning. Rotary engines reversed that, holding the shaft tightly cooled. and the engine spinning. The propeller was mounted to the rotating engine, which stayed cool by whirling its cylinders in the open air. 1 (c)-4 FOR TRAINING PURPOSE ONLY Issue 2 – June 2024 Engines – Piston engines Numerous types of Gnome engines were designed and built, one of the most famous being the 165-hp 9-N Monosoupape (one valve). It was used during the first world war, primarily in the Nieuport 28. The engine had one valve per cylinder. Having no intake valves, its fuel mixture entered the cylinders through circular holes or ports cut in the cylinder walls. The propeller was bolted firmly to the engine and it, along with the cylinders, turned as a single unit around a stationary crankshaft rigidly mounted to the fuselage of the aeroplane. The rotary engine used castor oil for lubrication. 1 (c)-5 FOR TRAINING PURPOSE ONLY Issue 2 – June 2024 Engines – Piston engines During the First World War, rotaries attained tremendous Thus, the air-cooled radial engine emerged. This type of air- popularity. They were less complicated and easier to make cooled engine arranged its cylinders to extend radially outward than the water-cooled type. They powered such outstanding from its hub, like the spokes of a wheel. The US Navy became fighter aeroplanes as Germany’s Fokker DR-1 and Britain’s an early supporter of radials, which offered reliability and Sopwith Camel. They used castor oil for lubrication because it lightweight. This was an essential feature if aeroplanes were to did not dissolve in gasoline. However, they tended to spray this take off successfully from an aircraft carrier’s flight deck. oil all over, making a smelly mess. Worse, they were limited in power; the best reached 190 to 210 kW (260 to 280 hp). With financial support from the Navy, two American firms, Wright Aeronautical and Pratt & Whitney, began building air- Thus, in 1917, a group of American engine builders returned to cooled radials. The Wright Whirlwind, in 1924, delivered water cooling as they sought a 300-kW (400-hp) engine. The 164 kW (220 hp). The Pratt & Whitney Wasp was tested at engine that resulted, the Liberty, was the most powerful aircraft 306 kW (410 hp) a year later. engine of its day, with the US car industry building more than 20,000 of them. Water-cooled engines built in Europe also Aircraft designers wanted to build aeroplanes that could fly at outperformed the air-cooled rotaries and lasted longer. With the high altitudes. High-flying aeroplanes could swoop down on war continuing until late 1918, the rotaries lost favour. their enemies and were harder to shoot down. Bombers and passenger aircraft flying at high altitudes could fly faster In this fashion, designers returned to water-cooled motors that because air is thin at high altitudes, and there is less drag in again were fixed in position. They stayed cool by having water the thinner air. These aeroplanes also could fly farther on a tank or antifreeze flow in channels through the engine to carry away of fuel. the heat. A radiator cooled the heated water. In addition to offering plenty of power, such motors could be enclosed But aircraft engines produced much less power because the air entirely within a streamlined housing to reduce drag and thus was thinner at high altitudes. They needed air to operate, and produce higher speeds in flight. Rolls Royce, Britain’s leading they could not produce power unless they had sufficient. engine builder, built only water-cooled motors. Designers responded by fitting the engine with a supercharger. This was a pump that took in air and compressed it. The extra Air-cooled rotaries were mostly out of the picture after 1920. air, fed into an engine, enabled it to continue to put out full Even so, air-cooled engines offered tempting advantages. power even at high altitudes. They dispensed with radiators that leaked, hoses that burst, cooling jackets that corroded, and water pumps that failed. 1 (c)-6 FOR TRAINING PURPOSE ONLY Issue 2 – June 2024 Engines – Piston engines Sopwith camel A Caudron seaplane being craned onto La Foudre in 1914 1 (c)-7 FOR TRAINING PURPOSE ONLY Issue 2 – June 2024 Engines – Piston engines Early superchargers underwent tests before the end of the First Leaded gasoline improves an aircraft engine’s performance by World War, but they were heavy and offered a slight advantage. enabling it to use a supercharger more effectively while using The development of superchargers proved to be technically less fuel. The results were spectacular. The best engine of the demanding. However, by 1930, the best British and American First World War, the Liberty, developed 300 kW (400 hp). engines had installed such units routinely. The Army funded During the Second World War, The Rolls-Royce Merlin engine supercharger work in the United States at another engine was about the same size – and put out 1,640 kW (2,200 hp). builder, General Electric. After 1935, engines fitted with GE’s Samuel Heron, a long-time leader in developing aircraft superchargers gave full power at heights above 9,000 m engines and fuels, writes, “It is probably true that about half the (30,000 ft). gain in power was due to fuel”. Fuels for aviation also demanded attention. When engine These advances in supercharging and knock-resistant fuels designers tried to build motors with greater power, they ran into laid the groundwork for the engines of the Second World War. the problem of ‘knock’. This had to do with the way fuel burned In 1939, the German test pilot Fritz Wendel flew a piston- within them. An aeroplane engine had a carburettor that took in powered fighter to a speed record of 755 KM/H (469 mph). US fuel and air, producing a highly flammable mixture of gasoline bombers used superchargers to carry heavy bomb loads at vapour with air, which went into the cylinders. There, this mix 10,000 m (34,000 ft). They also achieved a long-range; the was supposed to burn very rapidly but in a controlled manner. B-29 bomber had a range of 9,000 km (5,600 miles). Fighters Unfortunately, the mixture tended to explode, which damaged routinely topped 640 KM/H (400 mph). Airliners, led by the engines. The motor was then said to knock. Lockheed Constellation, showed that they could fly non-stop from coast to coast in the USA. Poor-grade fuels avoided knock but produced little power. Soon after the First World War, an American chemist, Thomas By 1945, the jet engine was drawing both attention and Midgely, determined that small quantities of a suitable chemical excitement. Jet fighters came quickly to the forefront. However, added to high-grade gasoline might help it burn without knock. while early jet engines dramatically increased speed, they He tried several additives and found that the best was tetraethyl showed poor fuel economy. It took time before engine builders lead. The US Army began experiments with leaded aviation fuel learned to build jets that could sip fuel rather than gulp it. Until as early as 1922; the Navy adopted it for its carrier-based that happened, the piston engine retained its advantage in aircraft in 1926. Leaded gasoline became standard high-test bombers and airliners, which needed to fly a great distance fuel, used widely in cars and aircraft. without refuelling. 1 (c)-8 FOR TRAINING PURPOSE ONLY Issue 2 – June 2024 Engines – Piston engines Messerschmitt Bf109 Supermarine Spitfire 1 (c)-9 FOR TRAINING PURPOSE ONLY Issue 2 – June 2024 Engines – Piston engines Rolls Royce Merlin engine – the powerplant of the Supermarine Spitfire and Avro Lancaster 1 (c)-10 FOR TRAINING PURPOSE ONLY Issue 2 – June 2024 Engines – Piston engines A modern-day horizontally opposed gasoline aero-engine 1 (c)-11 FOR TRAINING PURPOSE ONLY Issue 2 – June 2024 Engines – Piston engines Examples of modern aero-gasoline engines Rotax two-stroke engines Rotax build engines for several speciality markets, including Continental IOF-240 engine motorcycles, watercraft, and snowmobiles. The single- When introduced in 2002, the engine was rare because it cylinder two-stroke model 277, an early Rotax aircraft had neither magnetos nor mixture control. Instead, the engine, put out 26 hp, weighed just 30 kg (65 lbs) with ignition and fuel flow are controlled electronically. In addition reduction drive and exhaust, and cost just over $1,000. to providing the optimal fuel/air mixture – the IOF-240 burns From 1975 through today, Rotax has produced more than about 19 l (5 gal) per hour – the electronic system allows for 170,000 aircraft engines, most of them two-stroke more accurate engine analysis and troubleshooting. The lightweight models. From the 277, Rotax progressed to IOF-240 was first introduced in the Liberty XL2, a two-seat building ever more capable and powerful models, including carbon fibre aeroplane developed from the Europa kit the popular 447 and 503 models up to the 582, one of the aeroplane, which became the first piston-engine aeroplane most technologically advanced two-stroke engines, with certified with FADEC. rotary valves, oil injection, dual carbs and electronic ignition. The 582, still in production today, powers dozens of light- aircraft models. 1 (c)-12 FOR TRAINING PURPOSE ONLY Issue 2 – June 2024 Engines – Piston engines Lycoming O-540 engine Continental TSIO-550 engine As had been done by other manufacturers – sometimes to good effect – Lycoming expanded the horsepower range of The six-cylinder, fuel-injected TSIO-550 is the turbocharged its four-cylinder aircraft engines by adding another pair of version of the IO-550, first introduced by Continental in opposed cylinders, creating a six-cylinder engine with 50% 1983. Engines in the IO-550 series produce anywhere from more potential power. The 540 series engines started in 110 to 270 kW (280 to 360 hp). In the 1990s, at Raytheon’s 1957 as new, larger personal and charter aeroplanes request, Continental tweaked the IO-550 to optimise the pressed the need for more power. Piper made great use of performance of the 225 kW (300 hp) engine that powers the the engine, most notably in its Navajo line-up, PA-32s, Beechcraft Baron 58. The result was a smooth, reliable Aztecs, Comanches and Mirages. Still, numerous other engine that was hard to beat in its class. With even better manufacturers opted for 540-power as well, including Aero performance at higher altitudes thanks to its dual Commander, Pitts and Robinson Helicopter’s R44. As with turbochargers, the TSIO-550 powers the three top- the four-cylinder version, the 540 is available in various performing modern-day single-engine certified piston models, with turbocharging a popular option. aeroplanes: the Cessna TTx, Cirrus SR22 and Mooney Acclaim. 1 (c)-13 FOR TRAINING PURPOSE ONLY Issue 2 – June 2024 Engines – Piston engines History of Diesel aero engines The aircraft Diesel engine or aero Diesel has not been widely The first successful flight of a Diesel-powered aircraft was used. Diesel engines were used in airships and were tried in made on 18 September 1928 in a Stinson model SM-IDX aircraft in the late 1920s and 1930s but were never widely Detroiter, registration number X7654. Around 1936, the heavier adopted. Their main advantages are their excellent specific fuel but less thirsty Diesel engines were only preferred over consumption and higher fuel density. Still, these have been gasoline engines when flight time was over 6-7 hours. outweighed by a combination of inherent disadvantages compared to gasoline-fuelled or turboprop engines. Entering service in the early 1930s, the two-stroke Junkers Jumo 205 opposed-piston engine was much more widely used The ever-rising cost of AVGAS and doubts about its future than previous aero Diesels. It was moderately successful in its availability have spurred a resurgence in aircraft Diesel engine use in the Blohm & Voss Ha 139 and even more so in airship production in recent years. use. In Britain, Napier & Son license-built the larger Junkers Jumo 204 as the Napier Culverin. However, it did not see Several manufacturers built Diesel aero engines in the 1920s production use in this form. A Daimler-Benz Diesel engine was and 1930s; the best known were the Packard air-cooled radial also used in Zeppelins, including the ill-fated LZ 129 and the Junkers Jumo 205, which was moderately successful Hindenburg. This engine proved unsuitable for military but proved unsuitable for combat use during the Second World applications, and subsequent German aircraft engine War. However, the Blohm & Voss Bv 138 trimotor maritime development concentrated on gasoline and jet engines. patrol flying boat was powered by the more advanced Junkers Jumo 207 powerplant. Its trio of Diesel Jumo 207s was more successful, conferring a maximum 2,100 km (1,300 miles) combat radius. Nearly 300 examples of the Bv 138 were built during the Second World War. The first successful Diesel engine explicitly developed for aircraft was the Packard radial Diesel of 1928–1929, which was laid out in the familiar air-cooled radial format similar to Wright and Pratt & Whitney designs and was contemporary with the Beardmore Tornado used in the R101 airship; the use of Diesel had been specified for its low-fire-risk fuel. 1 (c)-14 FOR TRAINING PURPOSE ONLY Issue 2 – June 2024 Engines – Piston engines The Centurion line of Diesel engines found early success on the Diamond DA-42 twin 1 (c)-15 FOR TRAINING PURPOSE ONLY Issue 2 – June 2024 Engines – Piston engines The Soviet Second World War era four-engine strategic Several factors have emerged to change this equation. Several bomber Petlyakov Pe-8 was built with Charomskiy ACh-30 new manufacturers of general aviation aircraft developing new Diesel engines. However, Diesels were replaced with radial designs have emerged. In Europe, in particular, AVGAS has gasoline engines later in the production run because of become very expensive. AVGAS is harder to obtain in several efficiency concerns. The Yermolaev Yer-2 long-range medium (particularly remote) locations than Diesel fuel. Finally, bomber was also built with Charomskiy Diesel engines. automotive Diesel technologies have significantly improved in recent years, offering higher power-to-weight ratios that are Other manufacturers also experimented with Diesel engines in more suitable for aircraft applications. this period, such as the French Bloch (later Dassault Aviation), whose MB203 bomber prototype used Clerget Diesels of radial Certified Diesel-powered light aeroplanes are currently design. The Royal Aircraft Establishment developed an available, and several companies are developing new engine experimental compression ignition (Diesel) version of the Rolls- and aircraft designs for the purpose – many of these run on Royce Condor in 1932, flying it in a Hawker Horsley for test readily available jet fuel (kerosene) or conventional automotive purposes. Diesel. Interest in Diesel engines in the post-war period was sporadic. The lower power-to-weight ratio of Diesels, particularly compared to turboprop engines, weighed against the Diesel engine. With fuel available cheaply and most research interest in turboprops and jets for high-speed airliners, Diesel-powered aircraft virtually disappeared. The near-death of the general aviation market in the 1990s saw a massive decline in developing new aircraft engine types. Napier & Son in Britain had developed the Napier Culverin, a derivative of the Junkers Jumo 205, before the Second World War and took up aero Diesel engines again in the 1950s. The British Air Ministry supported the development of the 2,200 kW (3,000 hp) Napier Nomad, a combination of piston and turboprop engines, which was exceptionally efficient in terms of brake-specific fuel consumption but judged too bulky and complicated and cancelled in 1955. 1 (c)-16 FOR TRAINING PURPOSE ONLY Issue 2 – June 2024 Engines – Piston engines Thielert TAE 125-02-99 4-cylinder Diesel engine Hybrid Air Vehicles HAV 304 Airlander 10, aerostatic aerodynamic lift has four Thielert Centurion 325 hp V8 diesel engines Cessna 172, with Centurion 120 engine Diamond DA42 twinstar powered by Austro-Diesel engines 1 (c)-17 FOR TRAINING PURPOSE ONLY Issue 2 – June 2024 Engines – Piston engines Examples of modern aero Diesel engines 1 (c)-18 FOR TRAINING PURPOSE ONLY Issue 2 – June 2024 Engines – Piston engines 1 (c)-19 FOR TRAINING PURPOSE ONLY Issue 2 – June 2024 Engines – Piston engines Piston engine world records First Most common The Wright brothers made the first controlled, sustained flight Lycoming Engines is a major American manufacturer of aircraft of a powered, heavier-than-air aircraft with the Wright Flyer on engines. With a factory in Williamsport, Pennsylvania, 17 December 1903, four miles south of Kitty Hawk, North Lycoming produces a line of horizontally opposed, air-cooled, Carolina, USA. four-, six- and eight-cylinder engines, including the only FAA- certified aerobatic and helicopter piston engines on the market. Fastest The company has built over 325,000 piston aircraft engines and On 2 September 2017, Steve Hinton Jr. established a new powers more than half the world’s general aviation fleet, both speed record for a propeller-driven piston-engine aircraft by rotary- and fixed-wing. Lycoming is an operating division of flying the heavily modified Second World War North American Avco Corporation, a subsidiary of Textron. P-51 Mustang, Voodoo, at an average speed of 855.41 km/h (531.53 mph). A Hawker Sea Fury holds the unofficial record for the fastest piston-engine aeroplane in level flight at 880 km/h (547 mph). Highest The Grob Strato 2C set the world altitude record for crewed piston-engine aircraft of 18,552 m (60,897 ft) on 4 August 1995. It was powered by two Teledyne Continental TSIO-550 turbocharged piston engines with Pratt & Whitney PW127 gas generator to provide a constant supply of pressurised air to the piston engine at high altitudes. This had the advantage of maintaining power at high altitudes. Each engine drove a 6 m (19’ 8") diameter five-bladed propeller. Largest The Lycoming XR-7755 was the largest piston-driven aircraft engine ever produced, with 36 cylinders totalling about 127 L (7,750 in³) of displacement and a power output of 3,700 kW (5,000 hp) for 2,740 kg (6,050 lbs). It was initially intended to be used in the ‘European bomber’ that eventually emerged as the Convair B-36. Only two examples were built before the project was terminated in 1946. 1 (c)-20 FOR TRAINING PURPOSE ONLY Issue 2 – June 2024 Engines – Piston engines Grob G-850 Strato 2 North American P51 Mustang Voodoo Wilbur Wright adjusts the engine of his aircraft, France 1908 Lycoming engine Lycoming XR7755 1 (c)-21 FOR TRAINING PURPOSE ONLY Issue 2 – June 2024 Engines – Piston engines Operating principles General The power of an internal combustion engine comes from When the piston slides downward because of the pressure of burning a mixture of fuel and air in a small, enclosed space. the expanding gas in the cylinder, the upper end of the When this mixture bums, it expands considerably, and the push connecting rod moves downward in a straight line. The lower or pressure created is used to move the piston, thereby rotating end of the connecting rod moves down and in a circular motion the crankshaft. This motion is eventually sent to the wheels that at the same time. This moves the throw, and, in turn, the throw move the vehicle. Since similar action occurs in each cylinder rotates the crankshaft; this rotation is the desired result. The of an engine, we will use one cylinder to describe the steps in crankshaft and connecting rod combination is a mechanism to the development of power. change straight-line or reciprocating motion to circular or rotary motion. The one-cylinder engine consists of four basic parts, as shown below. Each piston movement from top to bottom or from bottom to top is called a stroke. The piston makes two strokes (an upstroke First, we must have a closed cylinder at one end; this cylinder and a downstroke), as the crankshaft makes one complete is similar to a tall metal can that is stationary within the engine revolution. block. Inside this cylinder is the piston – a movable plug. It fits snugly into the cylinder but can still slide up and down easily. This piston movement is caused by fuel burning in the cylinder. The up-and-down movement of the piston is called reciprocating motion. This motion must be changed into rotary motion so the wheels or tracks of a vehicle can rotate. This change is accomplished by a throw on the crankshaft and the connecting rod, which connects the piston and crankshaft throw. The throw is an offset section of the crankshaft that scribes a circle as the shaft rotates. The top end of the connecting rod is connected to the piston and must, therefore, go up and down. The lower end of the connecting rod is attached to the crankshaft. The lower end of the connecting rod also moves up and down, but because it is attached to the crankshaft, it must also move in a circle. 1 (c)-22 FOR TRAINING PURPOSE ONLY Issue 2 – June 2024 Engines – Piston engines Conversion of reciprocating motion into rotational motion 1 (c)-23 FOR TRAINING PURPOSE ONLY Issue 2 – June 2024 Engines – Piston engines The piston is connected to the rotating crankshaft by a connecting rod. In view (A), the piston is at the beginning or top of the stroke. As the crankshaft rotates, the connecting rod pulls the piston down. When the crankshaft has rotated one-half turn, the piston is at the bottom of the stroke (green ghost). As the crankshaft rotates in view (B), the connecting rod pulls the piston down. The piston continues moving downward until the motion of the crankshaft causes it to begin moving up. The position of the piston at the instant its motion changes from down to up is known as the bottom dead centre (BDC). The position of the piston at the instant its motion changes from up to down is known as the top dead centre (TDC). The term ‘dead’ indicates where one motion has stopped (the piston has reached the end of the stroke) and its opposite turning motion is ready to start. These positions are called ‘rock’ positions. 1 (c)-24 FOR TRAINING PURPOSE ONLY Issue 2 – June 2024 Engines – Piston engines Top dead centre (TDC) and bottom dead centre (BDC) Basic piston engine 1 (c)-25 FOR TRAINING PURPOSE ONLY Issue 2 – June 2024 Engines – Piston engines The 4-stroke cycle engine In this type of engine, four strokes are required to complete the Two complete crankshaft revolutions (720°) are required for the required series of events or the operating cycle of each four-strokes. Thus, each cylinder in an engine of this type fires cylinder. once in every two revolutions of the crankshaft. In the following discussion of the four-stroke cycle engine operation, note that 1 – Induction (first down-stroke) the timing of the ignition and the valve events vary considerably The inlet valve opens, the piston moves down the cylinder (from in different engines. Many factors influence the timing of a TDC to BDC), and the fuel/air mixture is drawn into the cylinder specific engine, and the engine manufacturer’s (the charge). recommendations in this respect must be followed in maintenance and overhaul. The timing of the valve and ignition 2 – Compression (first upstroke) events is always specified in the degrees of crankshaft travel. Both valves are closed, the piston moves back up the cylinder Remember that a certain amount of crankshaft travel is (from BDC to TDC), and the fuel/air mixture is compressed into required to open a valve fully; therefore, the specified timing the combustion chamber. represents the start of opening rather than the valve’s fully open 3 – Power (second down-stroke) position. An example of the four-stroke cycle can be seen Both valves remain closed, and a spark ignites the compressed below. fuel/air mixture. The rapid expansion of the burning mixture forces the piston back down the cylinder (from TDC to BDC). 4 – Exhaust (second upstroke) The exhaust valve opens, and the piston moves back up the cylinder (from BDC to TDC). After performing their work on the power stroke, the burnt gases are expelled into the atmosphere. 1 (c)-26 FOR TRAINING PURPOSE ONLY Issue 2 – June 2024 Engines – Piston engines The gasoline internal combustion e