DT011-1 Aircraft Engines 1 - Gas Turbine Engines PDF
Document Details
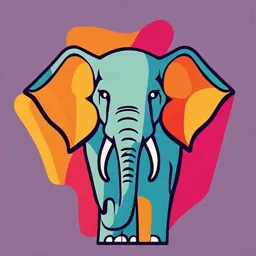
Uploaded by GreatestFarce
Tags
Summary
These notes provide an overview of gas turbine engines, including their fundamentals, physics, and different types. The document covers topics such as the Physics of Motion, Propulsion principles, the Brayton Cycle, and Types of Gas Turbine Engines, such as Ramjet, Pulse-Jet, Turbojet, Turboprop and Turboshaft.
Full Transcript
DT011-1 Aircraft Engines 1 - Gas Turbine Engines Module 15 Gas Turbine Engines...
DT011-1 Aircraft Engines 1 - Gas Turbine Engines Module 15 Gas Turbine Engines Contents 15.1 Fundamentals Thrust Specific Fuel Consumption (TSFC)......................... 7 Factors Affecting Thrust......................................... 9 Physics....................................................... 2 Exhaust Nozzle Jet Velocity.................................... 9 Mass...................................................... 2 Airspeed................................................... 9 Force...................................................... 2 Mass Airflow................................................ 9 Work...................................................... 2 Ram Effect................................................ 10 Power..................................................... 2 Engine Efficiency.............................................. 11 Energy..................................................... 3 Propulsive Efficiency......................................... 11 The Physics of Motion........................................... 5 Speed..................................................... 5 Velocity.................................................... 5 15.3 Inlet Momentum................................................. 5 Air Inlet Duct................................................... 2 Acceleration................................................ 5 General.................................................... 2 The Physics of Gas Flow...................................... 5 Subsonic Inlet Ducts.......................................... 2 Pressure................................................... 6 Supersonic Inlet Ducts........................................ 5 Heat and Temperature........................................ 7 Ice Protection.................................................. 7 Newton’s Laws of Motion...................................... 8 Hot Air System.............................................. 7 Propulsion Principles........................................... 9 Compressor Inlet Screens, Sand and Ice Separators................. 8 Brayton Cycle................................................. 11 Types of Gas Turbine Engines................................... 13 15.4 Compressors Ramjet.................................................... 13 Types of Compressors.......................................... 2 Pulse-Jet.................................................. 13 Centrifugal Compressors...................................... 2 Turbojet Engines............................................ 14 Axial-Flow Compressors....................................... 4 Turboprop................................................. 14 Guide Vanes and Stator Vanes................................. 5 Turboshaft................................................. 15 Compression Ratio........................................... 6 Turbofan.................................................. 16 Compressor Design Features..................................... 8 Turbine Engine Terms and Definitions............................ 18 Convergent Airflow Path....................................... 8 Blade Attachment............................................ 9 15.2 Engine Performance Types of Axial-Flow Compressors................................ 11 Pressure-Velocity-Temperature through the Engine.................. 2 Single-Spool Axial-Flow Compressors........................... 11 The Production of Thrust........................................ 3 Dual-Spool Axial-Flow Compressors............................ 11 Net Thrust.................................................. 3 Triple-Spool Axial-Flow Compressors............................ 12 Gross Thrust................................................ 4 Airflow Control and Anti Stall Systems............................ 13 Turbofan Engine Thrust....................................... 5 Surges and Stalls........................................... 13 Distribution of Thrust.......................................... 5 Angle of Attack............................................. 13 Flat Rated Thrust............................................ 6 Compressor Bleed Valves.................................... 14 Measurement of Thrust........................................ 6 Variable Inlet Guide Vanes.................................... 16 15.Contents - I Module 15 Gas Turbine Engines Contents Variable Bypass Valves and Bleed Ring.......................... 18 Engine Vibration............................................... 20 Fan Balancing.............................................. 20 Balancing Methods.......................................... 22 Installing Weights........................................... 22 Diffuser Section............................................... 25 15.5 Combustion Section Principles of Operation.......................................... 2 Multiple-Can Combustors...................................... 4 Can-Annular Combustors...................................... 5 Annular Combustors.......................................... 6 Reverse-Flow Annular Combustors.............................. 7 15.6 Turbine Section Turbine Section Elements........................................ 2 Turbine Design and Construction................................ 4 Turbine Failures................................................ 8 Materials................................................... 8 Nozzle Guide Vanes.......................................... 8 Turbine Discs............................................... 8 Compressor-Turbine Matching.................................. 9 15.7 Exhaust Turbine Engine Exhaust......................................... 2 Noise Suppressors............................................. 5 Thrust Reversers............................................... 6 15.Contents - II Module 15 Gas Turbine Engines 15.1 Fundamentals 15.1 Fundamentals 15.1 - 1 Module 15 Gas Turbine Engines 15.1 Fundamentals Physics We can say: For a clear understanding of jet propulsion principles it is necessary to understand F = pA the applicable principles of physics. These are the physical principles which gov- ern the action of mass or matter. The physics described here, however, are not intended to be complete in this regard but rather to present the basic ideas neces- Where: F=Force (N), P=Pressure (N/m2), A=Area (m2) sary for understanding of the physical relationships of gases and the turbo-ma- chinery within a gas turbine engine. Work The mass-flow of gases referred to is atmospheric air which is compressed and accelerated in the gas turbine engine to create useful work at the turbine wheel Work is the transfer of energy to a body by the application of a force that moves and ultimately, thrust. The thrust is created from either pure reaction to the flowing the body in the direction of the force. gases or from a propeller or fan driven by a turbine. Some of the most important physical properties that apply to the gas turbine en- W = Fs gine are: Weight, Density, Temperature, Pressure, and Mass. These properties are useful when applied to formulas such as: Force, Work, Ac- celeration, Thrust. Where: F=Force (N), s=Distance (m), W=Work (Joules) Force: Force is defined as the capacity to do mechanical work. Power Work: Mechanical work is present when a force acting on a body causes it to move Power is the rate at which work is done. It is the number of Joules (foot-pounds) through a distance. of work done in a specified length of time. Power is expressed in Watts (foot- Acceleration: In physics, acceleration is defined as a change in velocity with re- pounds per minute or foot-pounds per second). spect of time. Thrust: Newtons‘s second law states that force is proportional to the product of W mass and acceleration. P = ---- t Mass Where: W=Work (Joules), t=Time (secs.), P=Power (Watts) Mass is a measure of the amount of matter an object contains. The mass of the object is not dependent upon gravity and is therefore different from, but relates to, the weight of the object. It is common practice to express both mass and weight in terms of pounds. The thrust produced by a gas turbine engine is determined by the mass flow of air through the engine. Force Force is a vector quantity (a quantity that has both direction and magnitude) that causes an object to accelerate in the direction of its application. In practical me- chanics such as this study of gas turbine engines, force is normally expressed in Newtons (pounds). 15.1 - 2 Module 15 Gas Turbine Engines 15.1 Fundamentals Horsepower nozzle is placed in each sprinkler arm, 180° apart. If a constant fluid supply is pres- The horsepower is a commonly used measure of power. One horsepower is equal ent, at the outlets, there is a reduced pressure as the fluid escapes. This creates to 33’000 foot-pounds of work done in one minute, or 550 foot-pounds of work a relative higher pressure on the inside wall opposite the opening. The prinkler done in one second. One horsepower is also equal to 745.7 Watts of power. head will rotate as a result of its motive power. Kinetic energy in the air flowing through a jet engine relates to its velocity. This en- Table 1: Unit Conversions ergy is called the dynamic pressure energy and is created by the extra pressure caused by the movement of the fluid flow. 1lbf = 4.45 N Force 1N = 0.225 lbf 1 ft lbf = 1.356 J Work 1J = 0.737 ft lbf 550 ft lbf 1 hp = ---------------- sec Power 1 hp = 745.7 W 1W = 0.00134 hp Energy Is the ability to produce useful work. Considering the gas turbine engine, it means producing motion out of heat. The two forms of energy which best describe the propulsive power of jet engine are potential and kinetic. Potential Energy Potential or stored energy is the energy in an object derived from its position or condition. Figure 1 on page 4 illustrates that pressure is equal in all parts of the sprinkler head (outlets closed) and no motive power is present. In this manner po- tential or stored energy is present. In a fluid or gas flow the pressure energy is called the static pressure and would be the pressure experienced by a body submerged in the fluid flow and moving with it at the same velocity. Kinetic Energy Kinetic energy is the energy possessed by an object because of its motion. Figure 1 on page 4 demonstrates how kinetic energy is formed. An outlet or jet 15.1 - 3 Module 15 Gas Turbine Engines 15.1 Fundamentals Figure 1: Energy Axis Fluid Supply Potential Energy Direction of Movement Jet Axis Jet Direction of Fluid Supply Movement Kinetic Energy Jet Axis Jet Fluid Supply 15.1 - 4 Module 15 Gas Turbine Engines 15.1 Fundamentals The Physics of Motion Where: v2=Final velocity (m/sec)., V1=Initial velocity (m/sec), t=time in sec., a= Ac- celeration (m/sec2) Many important aspects of the practical physics of gas turbine engines relate to motion and the forces, which cause or change it. The Physics of Gas Flow The principle upon which a gas turbine engine operates is that of accelerating a Speed mass of air as it passes through the engine by the addition of energy from burning Speed is a measure of the rate of motion and is normally considered to be the dis- fuel. Understanding the things that happen to the air as it passes through the en- tance travelled divided by the time used to travel. Speed does not take direction of gine helps us understand the way thrust is developed. travel into consideration and is expressed in such terms as feet per second. Bernoulli’s Principle Velocity We can best understand the important relationship between the two basic forms Velocity is a vector quantity whose magnitude is the speed of the object and whose of energy (kinetic and potential) by studying Bernoulli’s principle. It states that the direction is the direction in which the object is moving. total energy in a column of moving fluid remains constant throughout the column. This total energy is made up of potential energy in the form of pressure, and kinetic This is calculated as energy in the form of velocity. s Figure 2 on page 6 shows a duct carrying a flow of fluid. V = - At point 1, the fluid has the velocity v1 and pressure p1. As the fluid moves through t the duct, it reaches the orifice. Where: V=Velocity (in m/s.), s=Distance (m), t=Time (sec) At point 2, where the velocity increases to v2, and the pressure decreases to p2. The product of v2p2 is equal to that of v1p1. In other words, the total energy is the Momentum same at both locations. As the flow continues, the duct widens to its original dimen- Momentum is a measure of the motion of an object equal to the product of its mass sions. The fluid slows to its original velocity and its pressure rises to its original val- times its velocity. In the study of gas turbine engines, the momentum of air flowing ue. The total energy has not changed. through the engine is found by dividing the weight of the airflow, in kg per second, The relationship stated by Bernoulli’s principle is that when the total energy in mov- by the acceleration due to gravity (9.81m/sec2). This is then multiplied by the ve- ing fluid is not changed, any increase in the fluid’s velocity will result in a corre- locity of the air in feet per second. sponding decrease in its pressure. Acceleration Acceleration is the amount the velocity of an object (measured in metres per sec- ond) is increased by a force during each second it is acted upon by that force. Ac- celeration is normally expressed in terms of metres per square second, (m/sec2.). Deceleration is similar to acceleration, but is a measure of the decrease of an ob- ject’s velocity. v2 – v1 a = ---------------------- t 15.1 - 5 Module 15 Gas Turbine Engines 15.1 Fundamentals Figure 2: Bernoulli Duct Figure 3: Pressure Measuring p p p Vacuum 1 2 3 v v v 1 2 3 Atmospheric 760mm Pressure (29.92in) at Sea Level 1 2 3 When energy is neither added to nor taken from fluid in motion, any restriction causes the fluid to speed up and its pressure to decrease. Pressure The pressure of a fluid is the amount of force it applies to a given area. Pressure Water is normally measured in pounds per square inch or in inches of mercury. A pres- p Manometer atm sure of one inch of mercury is the pressure that will support a column of mercury one inch high. There are three types of pressure commonly used in gas turbine engine technology: absolute pressure, gage pressure, and differential pressure. Pressure must be measured from some reference level. Absolute pressure is ref- erenced from zero pressure, or a vacuum. Gage pressure is referenced from the p Tot p existing atmospheric pressure, and differential pressure is the difference between Static p two pressures. Two additional types of pressure important in the study of gas tur- Ram bine engines are static pressure and total pressure. Static pressure is the pressure p of still air, but when air is in motion, its pressure increases because of the ram ef- Static fect. The total pressure is therefore the sum of the static pressure and the pressure that results from ram effect, or ram pressure. p +p Ram Static P Total = P Static + P Ram Duct 15.1 - 6 Module 15 Gas Turbine Engines 15.1 Fundamentals Heat and Temperature changes from a liquid into a solid, and the temperature at which pure water chang- es from a liquid into a gas. A gas turbine engine is a heat engine. Its purpose is to convert the chemical ener- gy in the fuel into heat energy, and then to convert this heat energy into useful work Two types of temperature are important in the study of gas turbine engines. These or thrust. Temperature is a measure of the effect of heat energy, and it is ex- are static temperature and total temperature. Static temperature is the tempera- pressed in terms of degrees as measured on four different scales. The two most ture of the ambient or surrounding air that is still, or not moving. Total temperature commonly used scales are Celsius (°C) and Fahrenheit (°F). As their reference is the temperature a column of moving air has when stopped. Total temperature is point, both scales use the temperatures at which pure water freezes and boils. The the sum of the static temperature and the temperature rise caused by the ram ef- Celsius scale divides the temperature spread between these points into 100 equal fect. degrees. Water freezes at 0°C and boils at 100°C. The Fahrenheit scale divides the spread into 180 equal degrees. Water freezes at 32°F and boils at 212°F. Ab- solute zero is -273°C and -460°F. Absolute temperature is measured in Kelvin and Rankine degrees. The Kelvin scale uses the same divisions as the Celsius scale. All molecular motion stops at 0K; water freezes at 273K and boils at 373K. Figure 4: Temperature Scales K °C °F Water Boils 373° 100° 212° Water Freezes 273° 0° 32° 0° Absolute Zero 0° -273° -460° Kelvin Celsius Fahrenheit The Three temperature scales use the same three reference points: the tempera- ture at which all molecular motion ceases, the temperature at which pure water 15.1 - 7 Module 15 Gas Turbine Engines 15.1 Fundamentals Newton’s Laws of Motion Figure 5: Newton’s Third Law Many events that take place in a gas turbine engine may be explained by one or more of Newton’s laws of motion. Newton’s first law is called the law of inertia. It states that an object at rest will re- main at rest, and an object in motion will continue in motion in a straight line and will not change its velocity unless it is acted upon by an outside force. Pressure Newton’s second law is called the law of acceleration. It states that the amount an object will accelerate is inversely related to its mass and directly related to the amount of force acting on it. It is expressed by F = ma Action - Reaction (Thrust) 2 Where: F = Force (N), m = Mass (kg), a = Acceleration (m/sec ) Newton’s third law is the law of action and reaction. It states that for every action there is an opposite and equal reaction. Velocity Acceleration 15.1 - 8 Module 15 Gas Turbine Engines 15.1 Fundamentals Propulsion Principles Newton’s third law of motion, which states that every action, has an equal and op- posite reaction, is the basic explanation for all aircraft propulsion. An aircraft power The principle of thrust produced by jet propulsion was known as early as the first plant, whether it be a reciprocating engine and a propeller, or a turbojet or rocket century A.D. Hero, or Heron, a mathematician of Alexandria in Egypt, is credited engine, produces thrust in basically the same way, by changing the momentum of with inventing a steam engine called an aeolipile. Steam generated in a boiler was a mass of air. There are two basic methods of producing thrust to propel an air- directed into a hollow sphere mounted above the boiler on an axle. Bent nozzles craft: aerodynamic action and jet reaction (or jet propulsion). protruded from the periphery of the sphere in such a way that steam escaping from 1. Aerodynamic action produces thrust when an engine turns a propeller, a ro- the nozzles produced a reaction force that rotated the sphere. The principle is tating airfoil that produces lift along a horizontal plane. The wash from this lift much the same as that of the rotating lawn sprinkler, which is spun by the reaction accelerates a large mass of air rearward through a small change in velocity. of water as it leaves the nozzles. The Chinese made the first practical applications of jet propulsion when they devised solid-fuel rockets. A rocket engine contains 2. Jet reaction engines, commonly called jet engines, produce thrust by heating chemicals that, when ignited, release gases and a tremendous amount of heat. a mass of air inside the engine and discharging it at a high velocity through a The heat expands the gases, which are ejected at a high velocity from a nozzle at specially shaped nozzle. The amount of thrust is determined by the mass of the rear of the rocket. the air and by the amount it is accelerated. Jet engines accelerate a small mass of air through a large change in velocity (see Figure 4 below). Figure 6: The Principle of Hero’s Aeolipile Basically we accelerate a mass of air toward the rear of the engine. This gives us a reaction on the opposite direction called thrust (Laws of Motion). Different types of propulsion systems provide different mass airflows and flow ve- locities(see “Figure 7” on page 10). It is possible to have quite different massflows and accelerations values and still have the same thrust, whether the engine is a piston engine propeller combination, a turbo-prop, a turbojet, or a turbofan. Based on Newton’s second law, a mathematical comparison of Static thrust can be made as follows (see “Figure 7” on page 10): Ground runup => v1=0 m: Air mass, a: acceleration, F: static thrust, v: speed, t: time v2 – v1 v kg a = ------------------- - => a = --- (t=1s) => Mass flow = airflow ( ------- ) = m t t s Turboprop Turbojet F = ma m m F = 385kg 70 ------- F = 55kg 490 ------- 2 2 s s = 26.95kN = = 26.95kN 15.1 - 9 Module 15 Gas Turbine Engines 15.1 Fundamentals Figure 7: Comparison of Thrust Producing Methods Acceleration of large mass Acceleration of small mass through through small increase in velocity large increase in velocity Turboprop Turbojet Thrust Thrust 15.1 - 10 Module 15 Gas Turbine Engines 15.1 Fundamentals Brayton Cycle Figure 8: The Bryton Cycle At the end of the 18th century, George Brayton a Boston engineer, scientifically de- scribed the continuous combustion cycle. This name, the Brayton cycle, has been B Combustion Pressure (heat energy added) given to the continuous thermodynamic cycle of the gas turbine engine. The Bray- ton cycle is also widely known as a “constant pressure cycle”. The same events, C intake, compression, expansion, power, and exhaust that occur in the constant- volume Otto cycle used by reciprocating engines, take place in a gas turbine en- gine. The basic difference between the two cycles is that in an Otto-cycle engine, the events take place in the same location, in the engine cylinder, but at different times. In the Brayton cycle, the events occur at the same time, but at different Expansion through turbine and nozzle locations within the engine. Figure 9 on page 12 explains how the Brayton cycle operates: Compression In the Brayton cycle energy from the fuel is released at a relatively constant pres- (pressure sure. The addition of heat energy causes the volume of the air to increase. energy added) 1. Air enters the inlet duct and flows to the inlet of the compressor, point A, at ambient pressure. 2. The air passes through the compressor, which raises its pressure and de- creases its volume to that represented by point B. Ambient air A D 3. The air then passes into the combustion chamber where fuel is injected into it and burned. The pressure between points B and C remains relatively constant Volume as the heat energy is added, but the volume and temperature increase. 4. The hot gases leave the combustion chamber and pass through the turbine where energy is extracted and the pressure drops to near ambient at point D. The pressures between points A and D are nearly the same, but at D the vol- A-B B-C C-D ume and thus the velocity are much greater. The air has expanded at a rela- tively constant pressure. Air Inlet Compressor Burner Section Turbine Exhaust 15.1 - 11 Module 15 Gas Turbine Engines 15.1 Fundamentals Figure 9: Working Cycle Continuous Air Intake Compression Combustion Exhaust Intermittent 15.1 - 12 Module 15 Gas Turbine Engines 15.1 Fundamentals Types of Gas Turbine Engines Figure 10: Ramjet Engine Reciprocating engines have several built-in limitations that have been over-come Fuel by the gas turbine: Nozzles Combustion The reciprocating action of the pistons sliding back and forth in the cylinders Chamber Jet Nozzle causes vibrations and significant internal stresses for which the engine must be designed. As a result, they are heavy for the power they produce. Reciprocating engines have a large number of moving parts that require the engine to be overhauled when a relatively few hours of operation have been Air Inlet accumulated. The only way a reciprocating engine can produce thrust is by driving a propel- ler. Fuel Line A gas turbine engine, on the other hand, is extremely light for the amount of power Venturi it produces. It has few moving parts, and with the development of new materials, has achieved an exceptionally high TBO (time between overhauls). Its high versa- tility lies in the fact that it can be used to produce either thrust or torque to power Fuel Nozzle Combustion Chamber aircraft. Ramjet The aerodynamic duct is the simplest of all power plants that uses the atmosphere Inlet to support combustion. It is a duct with few component parts, designed to receive Air inlet air and change its velocity to static pressure. Fuel is added to the compressed Igniter air with the resultant combustion and expansion of gases. This combustion causes Jet Exhaust the mass airflow to quickly exit the engine. The change of velocity of inlet and ex- haust air results in reactive thrust. The ramjet engine is seen today in many military Pulse-Jet pilotless weapons delivery vehicles. A ramjet engine depends upon high-velocity air entering the engine to produce a It is similar to a ramjet except that the pulse-jet inlet is fitted with a system of air barrier that forces the expanding gases to exit the rear of the engine and generate inlet flapper valves. These valves are closed during combustion and provide the a forward thrust. device with a moderate static thrust that the ramjet does not have. This thrust is One limitation of a ramjet engine is that the airflow inside the engine must be not sufficient to enable a pulse-jet to take off under its own power and, therefore, slowed to a subsonic velocity in order to sustain combustion of the fuel-air mixture. must be rocket boosted for initial flight. The scramjet (supersonic combustion ramjet) engine has overcome this limitation Major development of the pulse-jet engine seems to have ceased with the German by its design which allows the fuel to be ignited and burn in the supersonic air trav- V-1 rocket of World War II, due to the poor performance of this engine design (see elling through the engine. “Figure 11” on page 14). Afterburners, used to augment the thrust of some turbojet and turbofan engines, are a form of ramjet. 15.1 - 13 Module 15 Gas Turbine Engines 15.1 Fundamentals Figure 11: Pulse-Jet Engine not expand enough to do useful work. Air under pressure must be mixed with the fuel before the gases produced by combustion can be successfully employed to make a piston engine or turbojet operate. The more air that an engine can com- Shutter Valves open Jet Nozzle press and use, the greater is the power or thrust that it can produce. Finding a sat- isfactory way to accomplish the difficult task of compressing the air was the biggest stumbling block for designers during the early years of turbojet engine develop- ment. Air Inlet Figure 12: Basic Components of a Turbojet Engine Compressor Combustors Tail Pipe and Jet Nozzle Combustion Chamber Diffuser Turbine Air Inlet Duct Shutter Valves closed Turboprop A turbine engine can drive a propeller by extracting most of the energy that re- mains in the exhaust gases after they have driven the compressor. This can be Turbojet Engines done by connecting the propeller to the compressor through a set of reduction gears. But the propeller can be more efficiently driven through appropriate reduc- The turbojet, as first patented by Sir Frank Whittle, had an impeller compressor, tion gears by a turbine separate from the core engine, the portion of the engine annular combustor, and a single stage turbine. Today it is possible to see many that drives the compressor. An engine that uses a separate turbine to drive the varieties of turbojet engine designs, but the basic components are still the com- propeller is called a free-turbine engine. pressor, combustor, and turbine. A turboprop engine such the one shown in Figure 13 on page 15 uses a free tur- The turbojet gets its propulsive power from reaction to the flow of hot gases. Air bine to drive the propeller through a series of planetary reduction gears. enters the inlet and its pressure is increased by the compressor. Fuel is added in the combustor and the expansion created by the heat forces the turbine wheel to The total power of the turboprop is the sum of propeller thrust and exhaust nozzle rotate. The turbine in turn drives the compressor. The energy remaining down- thrust, with the exhaust thrust contributing from 5% to 25%, as seen in various en- stream of the turbine in the tail-pipe accelerates into the atmosphere and creates gines today. the reaction we refer to as thrust. The physical dimensions of a turboprop engine equals only about two third of that Today some large turbojets remains in use in military aviation, and in the last su- of a turbojet of comparable thrust, because the mass airflow for thrust is handled personic Concorde airliner. However, most supersonic aircraft now use turbofans. by the propeller rather than the core engine. The secret of why a turbojet will run as it does lies in the compressor. The gases created by a fuel and air mixture burning under normal atmospheric pressure do 15.1 - 14 Module 15 Gas Turbine Engines 15.1 Fundamentals Figure 13: Free Turbine Turboprop Engine Figure 14: Turboshaft Engine Propeller Free Turbine Reverse-Flow Combuster Fuel Nozzle Inlet Air Exhaust Planetary Reduction Reduction Gears Gear Case CL Turboshaft Turbine engines are ideally suited for powering helicopters, because they operate Gas Generator Power Output Free Power most efficiently at the constant RPM required by a helicopter. Most turboshaft en- Shaft Turbines gines drive their output shaft with a multistage free turbine that extracts as much energy as possible from the exhaust gases. The gas generator’s function is to produce the required energy to drive the power Turboshaft engines are widely used in such industrial applications like electrical turbine system. The gas generator extracts about two-third of the combustion en- power producing plants and surface transportation systems. In aviation, Turbos- ergy, leaving approximately one-third to drive the power turbine, which drives the hafts engines are used to power many modern helicopters and auxiliary power transmission. units. A turboshaft engine uses a multistage free turbine to extract as much energy as possible from the exhaust gases before they leave the engine. 15.1 - 15 Module 15 Gas Turbine Engines 15.1 Fundamentals Turbofan Figure 15: Low Bypass Turbofan (2:1) The turbofan, in effect, is a ducted, multi-bladed propeller driven by a gas turbine Low-pressure High-pressure Compressor Compressor High-pressure Turbine engine. Generally, turbofans contain 20 to 40 fixed pitch blades. They were devel- oped to provide a compromise between the best features of the turbojet and the turboprop. That is, with its ducted design, the turbofan has turbojet type cruise speed capability and yet retains some of the short field takeoff capability of the tur- boprop. Exhaust By comparison, the fan diameter of a turbofan engine is much less than that of the propeller on a turboprop engine, but it contains many more blades and moves the air with a greater velocity from its convergent exhaust nozzle. Turbofans in civil aircraft are generally divided into three classifications: Low bypass: turbofan engine whose bypass ratio is less than 2:1 Low-pressure Turbine Medium bypass: bypass ratio is between 2:1 and 4:1 Fan Fan Bypass Duct High bypass: bypass ratio is 4:1 or greater The amount of thrust produced by the fan varies between 30% and 85% of the total Figure 16: High Bypass Ratio Turbofan Engine (5:1) thrust, depending upon the bypass ratio. The bypass ratio is the ratio of the mass Fan of air moved by the fan to the mass of air moved by the core engine. High bypass engines boast the lowest fuel consumption of the various turbofan engines. The Fan Dischcarge Duct PW-4000 is a 5:1 bypass ratio engine with 80% of the thrust produced by the fan and 20% by the core engine. Low-pressure Compressor The high bypass turbofan engine today has become the most widely used engine High-pressure type for medium to large airliners because is offers the best fuel economy. This Compressor conversation of energy keeps more power within the engine to drive the fan and leads to a considerable increase in propulsive efficiency. This conservation of en- ergy keeps more power inside the engine to drive the fan and leads to a consider- able increase in propulsive and thermal efficiency. Core Engine In fact, the aviation industry today has, for the most part, turned to the various fan Exhaust engines and away form the turbojet engines for high performance business, com- mercial and military aircraft. Fan High-pressure Exhaust Turbine Low-pressure Turbine The actual development of turbofans leads to higher bypass ratios. actual engines like the Rolls Royce Trent 500 have bypass ratio higher than 7:1 or the Pratt & Whitney PW8000 with a bypass ratio of around 11:1(see “Figure 17” on page 17). 15.1 - 16 Module 15 Gas Turbine Engines 15.1 Fundamentals Figure 17: Actual High Bypass Engines was developed. The Unducted Fan” and the Prop-Fan” drive scimitar-shaped, multiblade, contrarotating, variable-pitch propellers. Their bypass ratios exceed 30:1 and they were capable of powering aeroplanes in the speed range of Mach Fan RR Trent 500 0.8. In order to propel an aircraft at such speed, the propeller speed had to travel at supersonic speeds. The prototype in service surpassed the high bypass engine in fuel efficiency by 15%-20%. The projects were abandoned since noise generated was at about 30-40 decibel higher than a high bypass turbofan engine of comparable size with. Figure 18: UDF Engine (GE Prototype) CL Low Pressure High Pressure Spool Spool Intermediate Pressure Spool Fan (Bypass Ratio 11:1) PW6000 Low Pressure Spool High Pressure Spool CL Gearbox (3:1) Ultra High Bypass Turbofan Engines During the 80 decade a special type of ultra-high-bypass (UHB) or unducted fan engine (UDF) engine with characteristics more like a turboprop than a turbofan 15.1 - 17 Module 15 Gas Turbine Engines 15.1 Fundamentals Turbine Engine Terms and Definitions Numerical station designations are assigned to the various sections of gas turbine engines to enable specific locations within the engine to be easily and accurately An aircraft gas turbine engine is divided into two sections: the cold section and the identified. hot section. The cold section includes the inlet air duct, the compressor, and the Note: For configurations other than the one shown, reference should be diffuser. The hot section includes the combustion section, the turbine, and the ex- made to manuals published by the engine manufacturer. haust system. The air inlet duct is technically a part of the airframe, but it is so im- portant in the development of thrust that it is included with the engine as a part of the cold section. In order to compare gas turbine engines, the industry has agreed upon certain standard abbreviations and symbols. A gas turbine engine is divided into two sections: the cold section and the hot sec- tion. Figure 19: Engine Stations Cold Section Hot Section FAN Stations 12 14 2 2.5 3 4 4.5 5 Core Stations 6 Gear Box 15.1 - 18 Module 15 Gas Turbine Engines 15.2 Engine Performance 15.2 Engine Performance 15.2 - 1 Module 15 Gas Turbine Engines 15.2 Engine Performance Pressure-Velocity-Temperature through the Engine Figure 1: P-V-T Graph A B C D The events that take place in the Bryton thermodynamic cycle occurs at the same time but at different locations within the engine. The changes in pressure, temper- ature and velocity are shown as they relate to the Bryton Cycle, see title "Bryton Cycle" in sub module 1. Velocity Temperature Pressure for Generating Thrust Burner Manifold Pressure Ambient Pressure Air Inlet Compressor Diffusor Burner Turbine Exhaust 15.2 - 2 Module 15 Gas Turbine Engines 15.2 Engine Performance The Production of Thrust The thrust developed by a turbojet results from the unbalanced forces created within the engine itself. When the static pressure at the nozzle exit exceeds the Horsepower, which is commonly used as a measure the output of a reciprocating static pressure of the ambient air, an additional force (thrust) is developed at the engine, is not an appropriate measure for the output of a turbojet or turbofan en- nozzle. gine, because time and distance are not always involved. When a turbojet engine Thrust produced by a turbojet or turbofan engine is determined by three things: is running but the aeroplane is stopped on the runway, the time and distance val- ues required for measuring power are not applicable. Torque and RPM are pro- 1. The change in momentum experienced by air flowing through the engine duced by the turbine, but the power developed is being used within the engine 2. The momentum of the fuel itself, primarily to turn the compressor. The engine is developing thrust and is 3. The force caused by the difference in pressure across the exhaust nozzle pushing against the aircraft, even when it is not producing external power. When multiplied by the area of the nozzle the brakes are released, the aeroplane moves forward. Thrust in pounds, rather than horsepower, is used as a measure of the propulsive force developed by a tur- There are two types of thrust: net thrust and gross thrust. bojet or turbofan engine. An other way to express thrust would be to say that a turbojet is essentially a ma- Net Thrust chine that increases the momentum of the mass of air and fuel that passes through When an aeroplane is flying, consider any unit of mass airflow has initial momen- it. tum at the engine inlet. Its velocity change across the engine will be greatly re- Momentum is the impulse imparted to the air, fuel and products of combustion that duced as compared to an engine at rest. pass through the engine. The thrust discussed far is net thrust. It is the thrust that results from the change The incoming momentum of air and the momentum of fuel entering a turbojet must in momentum of the mass of air and fuel that passes through the engine. Net thrust be subtracted from the outgoing momentum of the exhaust gases to arrive at the includes also the extra thrust at the nozzle exit when the discharge static pressure over-all change in momentum, which represents the force generated within the en- is higher than ambient pressure (chocked nozzle). gine. The opposite reaction to this force is the engine thrust. Fuel flow is usually neglected when computing net thrust, because the weight of air that leaks from various sections of the engine is approximately equivalent to the outgoing exhaust incoming air incoming fuel weight of fuel consumed. Considering this statement, net thrust can be expressed Fn = – + gas momentum momentum momentum as follows: Where: Fn = Net Thrust (Newtons) Outgoing exhaust Incoming air Fn = – gas momentum momentum Figure 2: Thrust Description through Momentum Theory Figure 3: Simplified Net Thrust Incoming Fuel Incoming Air Momentum Momentum Incoming Air Outgoing Exhaust Momentum Momentum Outgoing Exhaust Fn Momentum Vi Ve Fn Vi Ve Thrust Thrust 15.2 - 3 Module 15 Gas Turbine Engines 15.2 Engine Performance g m- ), Applying Newton’s third law and neglecting efficiency coefficients, net thrust can Where: Fg = Gross Thrust (N), m· = Mass Flow ( -s ), vE = Exhaust Speed ( -- s be simplified as follows: kg Ae = Exhaust Area (m2), pe = Exhaust Static Pressure ( ------2 ), F n = m· v e – v i + A e p e – p amb m g pamb = Ambient Pressure ( ------2 ) g m Where: Fn= Net Thrust (N), m· = Mass Flow ( -s ), vI= Inlet Speed ( m --s- ), Remember: when the term, thrust is used by itself in discussing a gas turbine vE= Exhaust Speed ( m ---s- ), Ae= Exhaust Area (m2), pe= Exhaust Static Pressure engine, the reference is only to net thrust unless otherwise stated. The thrust produced by a turbo jet results from the unbalanced forces and kg kg ( ------2 ), pamb= Ambient Pressure ( ------2 ) momentum created within the engine itself and not all developed at the jet m m nozzle. Gross Thrust Thrust with Chocked Nozzle It is the thrust developed at the engine exhaust nozzle. This includes both the thrust generated by the outgoing momentum of the exhaust gases and the addi- Many turbojet engines are equipped with a device called chocked exhaust nozzle. tional thrust resulting from the difference between the static pressure at the nozzle From cruise to takeoff power, pressure in the exhaust duct is pushing the gas with and the static pressure of ambient air. Gross thrust does no take the incoming mo- such force that the gas velocity reaches the speed of sound. Pressure at the noz- mentum of air and fuel into consideration. This means only when the engine is stat- zle does not return to ambient with a chocked nozzle but stays some were in ex- ic (ground run). cess of ambient.This pressure across the exhaust nozzle opening creates additional thrust by the principle of F = p A , or force equals pressure times ar- Outgoing exhaust ea. Fn = As chocking occurs, pressure starts to build in the tail pipe between the wall of gas momentum flowing gases in the constricting exhaust nozzle opening at the rear. As pressure builds above ambient pressure across the opening, a forward push is said to be Figure 4: Simplified Gross Thrust created. Outgoing Exhaust Momentum Fn Ve Thrust Without considering fuel flow, the equation for gross thrust is: F g = m· v e + A e p e – p amb 15.2 - 4 Module 15 Gas Turbine Engines 15.2 Engine Performance Figure 5: Comparison between Chocked and Unchocked Nozzle Turbofan Engine Thrust When computing the thrust produced by a turbofan engine, the thrust of the core Convergent Duct Convergent Duct engine is first computed and then the thrust produced by the momentum change Subsonic Flow Sonic Flow of air passing through the fan is computed. The total thrust is the sum of the thrust from the core engine plus the thrust from the fan. W X Y Z Thrust total = Thrust core + Thrust fan Distribution of Thrust 1) Speed W 1) Speed Y The net thrust in an engine is made up of several components, as seen in (see Becomes X Y Becomes Z “Figure 6” on page 5). 2) Pressure 2) Pressure Decrease Increases Figure 6: Distribution of Thrust through a Turbojet Engine 3) Axial Velocity 3) Axial Velocity Increase Stops 152'212N HI HI Increasing 182'978N Combustion Thrust p Turbines Force Static Pressure Pressure p 9'734N Static Chocked 24'879N Diffuser Thrust Unchocked Ambient Nozzle Force Ambient 84'825N 10'772N LO Compressor Thrust Inlet Exit Inlet Exit Chock Force Note: such kind of chocked exhaust nozzles are not used on modern high bypass jet engines. Subsonic exhaust gas path enables better propulsive ef- 49'686N ficiency and less noise levels. As the gas flow passes through the exhaust Resultant Thrust system a small forward force (thrust) acts on the exhaust cone, but in general only rearward forces are produced due to the drag of the gas flow at the noz- When momentum is added to the mass of air flowing through the engine, the thrust zle. is forward (pointing left on figure), and when momentum is lost, the thrust is rear- ward (pointing right on figure). The air flowing into the engine through the intake duct produces no thrust of either type, but as the air is compressed, its momentum 15.2 - 5 Module 15 Gas Turbine Engines 15.2 Engine Performance is increased by energy taken from the turbine, and a forward thrust is produced. Figure 7: EPR Measurement Example (PW 4000) As air passes through the combustion section, energy is added by the burning fuel and another increment of forward thrust is added. When the hot air leaves the combustion section, it flows through the turbine where much of its energy is ex- tracted to turn the compressor. This results in a rearward thrust. As exhaust gases expand through the convergent exhaust duct, more rearward thrust is produced. When all rearward components of thrust are subtracted from the forward compo- nents, the resultant thrust is the thrust available for propulsion. Flat Rated Thrust There are two definitions for this, the first one is associated with throttle settings at low altitude and the second one is associated with throttle settings at low temper- atures. In each case the engine is throttled or otherwise restricted in power output. Pressure Total Low altitude settings: the engine is able to produce a constant and predict- able power output at all flight levels up to a given altitude. Low temperature settings: the engine is able to produce a constant and pre- EPR dictable power output at all temperatures up a given limit. Ambient Pressure Measurement of Thrust The amount of thrust produced by a gas turbine engine is determined by the change in the momentum of air flowing through the engine. Thrust produced by a turbojet or turbofan engine may be measured on the ground in an engine test stand. The engine is mounted on a floating mount that pushes Compressor Turbine Discharge Inlet Total against a calibrated scale that has a remote indicator in the control booth. The in- Pressure Total Pressure dicator is calibrated in pounds or Newtons of thrust. It is necessary to know the amount of thrust an engine develops when operating in the aircraft. Some smaller CL jet engines, especially those with centrifugal compressors, use the compressor speed in % RPM as an indication of the thrust. RPM can be used because it is ap- proximately proportional to the thrust the engine is producing. Almost all-larger en- gines indicate the amount of thrust produced either by measuring the engine pressure ratio (EPR). It is a ratio of two engine pressures: Turbine discharge total The following example is of a Pratt & Whitney engine pressure ratio cockpit indi- pressure and compressor inlet total pressure. Each manufacturer uses a slightly cation. When turbine discharge pressure is 28.52 pounds per square inch- abso- different engine station numbering system, and engine stations are a means of lute and compressor inlet pressure is 14.7 pounds per square inch - absolute. identifying, engine pressure ratio tap-off points. For example: the Pratt & Whitney Company uses station 2 and 4.95, to identify the engine pressure ratio tap-off points of dual-spool engines. Pt5 28.52psia EPR = --------- = ------------------------ = 1.94 Pt2 14.7psia 15.2 - 6 Module 15 Gas Turbine Engines 15.2 Engine Performance Thrust Power The formula for ESHP is: When a jet-powered aircraft is moving, time and distance are relevant, then engine produces power (work). Thrust power can be computed when the thrust produced Fn by the engine in Newtons (pounds) and the speed of the aeroplane in metres per ESHP static = SHP + ------- second (foot per second) are known. Power is computed in Watts (horse power). 2.5 When an aeroplane is flying at 375 miles per hour, each pound of thrust is the equivalent of one horsepower. When the speed of the aeroplane is doubled, the amount of horsepower produced by each pound of thrust is also doubled. In flight, the ESHP considers the thrust produced by the propeller, which is found by multiplying the net thrust in pounds by the speed of the aircraft in miles per hour. Figure 8: Turbojet Engine Power Divide this by 375 times the propeller efficiency, which is considered to be 80%. Figure 9: Equivalent Shaft Power Net Thrust Speed/Distance Power Exhaust Fn Time Thrust Thrust Equivalent Fm Shaft Power Shaft Power Thrust power also provides for comparison between gas turbine engines with ref- erence to fuel consumption rates. The thrust horsepower produced by an engine and propeller can be approximated by multiplying the brake horsepower the engine is producing by an industry-ac- Thrust Specific Fuel Consumption (TSFC) cepted standard for propeller efficiency of 80%. If a reciprocating engine is produc- It is a ratio of fuel consumption to engine thrust. This ratio is usually included in any ing 1’000 brake horsepower, it is producing 800 thrust horsepower. set of engine specifications and affords a mean of comparing the fuel consumption Thrust horsepower = Brake horsepower* Propeller efficiency= 1’000*0.8= 800HP or economy of operation of one engine to an other regardless of its thrust rating. Specifically it is the amount of fuel in kilograms (pounds) consumed by an engine Equivalent Shaft Power while producing one Newton (lb.-force) of thrust. Turboprop engines are rated in their equivalent shaft power. This rating takes into consideration both the shaft power delivered to the propeller and the thrust devel- Total weight of fuel consumed oped at the engine exhaust. TSFC = ---------------------------------------------------------------------------- Newtons of thrust (net or gross) Under static conditions, one shaft horsepower is equal to approximately 2.5 pounds of thrust. 15.2 - 7 Module 15 Gas Turbine Engines 15.2 Engine Performance The comparison of a low bypass turbofan (JT8 D) with a modern high bypass tur- bofan (the CFM56-3), best indicates the current state of the art in engine fuel effi- ciency. Table 1: TSFC Comparison Engine Thrust TSFC (N ) kg ( -------------- ) kN h JT8 D 82.3 kN 51.47 CFM56-3 89.4 kN 39.25 TSFC in Flight The reason why TSFC is higher at operating altitude than at ground operating con- ditions is that, in order to keep engine power up when inlet density is dropping, more RPM (increasing fuel flow) is needed to maintain correct mass airflow. The increase in fuel flow per Newton (pound) of thrust results in higher TSFC. Thrust remains fairly constant as airspeed increases, therefore, as fuel flow in- creases for a given thrust, the TSFC value increases. 15.2 - 8 Module 15 Gas Turbine Engines 15.2 Engine Performance Factors Affecting Thrust Mass Airflow The basis of the thrust produced by a turbojet or turbofan engine is the change in Gas turbine engines operate under widely varying conditions of airspeed and alti- momentum of air flowing through the engine. Anything that increases the mass of tude that affect the amount of thrust the engine produces. These conditions affect air increases the thrust. Two factors that affect the mass of air are its density and either the momentum of the air and fuel flowing through the engine or the pressure differential across the exhaust nozzle. the ram effect. Air density has a profound effect on the thrust produced. The vol- ume of air flowing through the engine is relatively fixed for any particular RPM by All calculations of thrust made by the manufacturers are based on standard atmos- the size and geometry of the inlet duct system. But since thrust is determined by phere and all performance calculations must be adjusted for non-standard atmos- mass, not the volume of air, any increase in its density increases its mass and thus phere. the thrust. As the temperature of the air increases, its density decreases, and the Remember: at any given throttle setting, engine thrust will vary as the tem-