Molecular Biology I: Nucleic Acid Metabolism Lecture 03 PDF
Document Details
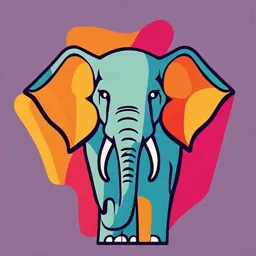
Uploaded by ZippyPelican
2024
Tags
Related
- Molecular Biology I: Nucleic Acid Metabolism Lecture 2 PDF
- 2024S1-Lecture-01-updated PDF: Molecular Biology I
- Molecular Biology I: Nucleic Acid Metabolism Lecture 04 (2024 S1) PDF
- Molecular Biology I: Nucleic Acid Metabolism Lecture 06 (2024 S1)
- Molecular Biology I: Nucleic Acid Metabolism Lecture 07 PDF
- Molecular Biology I: Nucleic Acid Metabolism Lecture 8 Slides 1-55 PDF
Summary
These lecture notes cover nucleic acid metabolism, focusing on DNA topology and topoisomerases. Key concepts include DNA structure, stability, and the role of topoisomerases in maintaining DNA integrity. The material is suitable for undergraduate students.
Full Transcript
MOLECULAR BIOLOGY I: NUCLEIC ACID METABOLISM SC/BIOL 3110, 2024 S1 Lecture 03: DNA topology and Topoisomerases DNA replication 1 Recap of last lecture: Key features of the double helix: Schematic diagram of DNA double helix: Right- vs left-handed helices 2 nm 2 Recap of last lecture: Differences...
MOLECULAR BIOLOGY I: NUCLEIC ACID METABOLISM SC/BIOL 3110, 2024 S1 Lecture 03: DNA topology and Topoisomerases DNA replication 1 Recap of last lecture: Key features of the double helix: Schematic diagram of DNA double helix: Right- vs left-handed helices 2 nm 2 Recap of last lecture: Differences between major and minor grooves: Most DNA sequence-specific binding proteins bind at the major groove of DNA e.g. Lambda phage repressor protein Minor groove binding proteins use positively charged side-chains to fit into the negatively charged minor grooves 3 Recap of last lecture: Distinct forms of DNA helices have unique features: 4 Recap of last lecture: Factors that contribute to the stability of dsDNA: 1. Hydrogen bonding between base pairs 2. Base stacking B-form helix DNA RNA Overall stability = sum of forces that keep strands together and forces that push strands apart A-form helix 5 Recap of last lecture: DNA complementarity and hybridization: Complementarity of the DNA strands allows for the denature and renature properties of ds DNA Many molecular biology techniques are based on the concept of hybridization What other parameters influence the stability of the DNA duplex? Temp Salt concentration pH 6 Recap of last lecture: Properties of DNA: Each nucleotide type has characteristic UV-light absorption profile ss- and ds-DNA absorb different amounts of UV à = hyperchromicity averaged Can use A260 to calculate dsDNA and ssDNA concentrations in solutions 7 Recap of last lecture: Properties of DNA: Transition from dsDNA to ssDNA during denaturation à hyperchromic shift Midpoint of hyperchromic shift = melting temp Tm 8 Recap of last lecture: DNA denaturation or melting: The exact calculation of Tm takes into account of all the physical factors that affects DNA hybridization However, in lab, often use the quick formula: Tm = 4oC x (number of G’s and C’s) + 2oC x (number of A’s and T’s) (note: only count the As, Cs, Gs, and Ts, on one strand of the DNA) You will need to know this formula for all midterms and final exam 9 Recap of last lecture Primary sequence of RNA folds into secondary structures: Note: in RNA, Gs can base pair with Cs or Us à increases the options for base pairing 10 Recap of last lecture Same primary sequence can fold into different theoretical secondary structures: e.g. same RNA sequence (394 nts) à 2 most stable 2o structures based on prediction 11 Recap of last lecture tRNA’s function is dependent on its secondary and tertiary structures: example of G:U base-pairing 12 Recap of last lecture Different types of RNAs: 1. mRNA 2. tRNA 3. rRNA 4. Catalytic RNA Technically, these are all “non-coding” RNAs, but ncRNA term by itself most often refers to long non-coding RNAs (lncRNA) 5. Long ncRNA e.g. XIST 6. siRNA/miRNA 13 Recap of last lecture Transesterification reaction induced by exposing RNA to high pH conditions: Deprotonation 2’, 3’ cyclic phosphate 14 Recap of last lecture RNase P Examples of catalytic RNAs: 1. RNase P 2. Self-splicing introns Group I Group II 15 Recap of last lecture Hammerhead catalytic RNA: Typically catalytic domain made up of 3 stem loops with Stems I and III base-pairing with target sequence flanking the cleavage site 3D architecture of the catalytic site promotes specific interactions with cofactors, especially divalent metal ions, to create optimal condition for 2’OH deprotonation and 16 RNA cleavage RNA structures: Other functional RNAs: A very simplified representation of XIST and X-inactivation: Fluorescent in situ hybridization (FISH) 17 Recap of last lecture Differences between siRNA and miRNA: Endogenous dsRNA (i.e. transcribed from own genome) Exogenous dsRNA (e.g. from RNA virus) siRNAs target mRNAs that have 100% complementarity miRNAs can target mRNAs that are 100% OR partially complementary 18 Recap of last lecture DNA topology: When a DNA double helix is fixed at both ends or closed (without ends), it is under topological constraint. In a “closed system”, such as covalently closed circular DNA, any under-winding, over-winding, knotting, or tangling of DNA changes the topological state of the DNA. CCC = covalently closed circle EM pics of knotty DNA 19 Properties of DNA DNA topology: The problem of topological constraints is especially relevant to processes such as transcription and DNA replication, which require unwinding of the DNA duplex 20 Properties of DNA DNA topology: Covalently closed circular DNA (cccDNA) is often used as a model for understanding properties of DNA topology The mathematical formula that describes DNA topology is: Lk = Tw + Wr Where Lk = Lk = Linking number Tw = Twist Wr = Writhe The number of times one strand would have to be passed through the other strand in order for the two strands to be entirely separated from each other 21 Properties of DNA DNA topology: Covalently closed circular DNA (cccDNA) is often used as a model for understanding properties of DNA topology The mathematical formula that describes DNA topology is: Lk = Tw + Wr Where Lk = Lk = Linking number Tw = Twist Wr = Writhe The number of times one strand would have to be passed through the other strand in order for the two strands to be entirely separated from each other à is always an integer (whole number) value Lk does NOT change unless there is a breakage in the DNA strand(s) 22 Properties of DNA DNA topology: Covalently closed circular DNA (cccDNA) is often used as a model for understanding properties of DNA topology The mathematical formula that describes DNA topology is: Lk = Tw + Wr Where Lk = Lk = Linking number Tw = Twist Wr = Writhe The number of times one strand would have to be passed through the other strand in order for the two strands to be entirely separated from each other à is always an integer (whole number) value Tw = the number of times one DNA strand completely wraps around the other strand Wr = the number of times the DNA duplex crosses over itself (supercoils) 23 Properties of DNA DNA topology: Topoisomerases cut and re-ligate DNA and, therefore; change the Lk A right-handed cross over = negative supercoil 24 Properties of DNA DNA topology: If DNA is in a relaxed state, the strands wrap around each other once every ~ 10.5 bp In the relaxed state, Wr = 0, so Lk = Tw If DNA is negatively supercoiled = Underwound If DNA is positively supercoiled = Overwound 25 Properties of DNA DNA wraps in anticlockwise direction DNA wraps in clockwise direction DNA topology: Two types of Writhe (supercoils): Interwound or Plectonemic Toroid or Spiral 26 Properties of DNA More DNA topology math: Lko = Linking number of the relaxed DNA For cccDNA, Lko = number of base pairs divided by 10.5 27 Properties of DNA More DNA topology math: The extent of supercoiling is measured by the difference between Lk and LkO, which is called the linking difference: DLk = Lk - LkO = Wr For normal B-form DNA, Lko = Tw 28 Properties of DNA More DNA topology math: If the DLk of a cccDNA is significantly different from zero, then the DNA is torsionally strained and hence it is supercoiled If DLk < 0, then the DNA is said to be “negatively supercoiled.” Negative supercoiling unwinds DNA Conversely, if DLk > 0, then the DNA is “positively supercoiled.” Positive supercoiling condenses DNA Most circular DNA isolated from bacteria or eukaryotes are negatively supercoiled Negative supercoiling stores energy to help processes such as DNA replication and transcription by making strand separation easier For animation to explain DNA topology, see: https://www.youtube.com/watch?v=az2c6UbEdug 29 DNA topology Lk = Tw + Wr 378 378 ? ?? ? Lk can only change if there is DNA breakage, such as by the action of topoisomerases 30 DNA topology Lk = Tw + Wr 378 378 Lk does not change because no topoisomerase, but physical removal of the supercoils results in separation of the strands 31 DNA topology Lk = Tw + Wr Separation of the strands, extent dependent on the number of supercoils removed 378 This 1 helical turn covers 52.5 bp; rest of DNA molecule maintains the 10.5 bp/helical turn 378 Because Lk doesn’t change, Tw is changed when Wr à 0 (physical removal of supercoiling) 32 DNA topology Biological relevance of DNA topology: Most organisms maintain their genomes in a net negative supercoiled state: physical removal of negative Wr helps to create DNA strand openings However, there are some thermophile bacteria (ones that grow under high temperature) that have positively supercoiled genomes à why? 33 DNA topology math: Recall Lk = Tw + Wr, so if Lk doesn’t change, then changing Wr normally necessitates a change in Tw to compensate. However, there are circumstances when neither Lk nor Tw can change. For example, when a cccDNA wraps around a nucleosome in a left-handed manner, this simultaneously adds both a negative and a positive supercoil to the system since Tw cannot be increased due to the physical constraints of the normal DNA double helix (need at least 10.5 bp to wrap one strand around the other for one complete helical turn). +ve supercoil Lk = Tw + Wr no change cannot -1 +1 34 DNA topology Importance of relaxing supercoiled DNA in living organisms: Example of when DNA topology is important in real life: 35 DNA topology Ways to relax supercoiled DNA: 1. DNase I: -introduce “nick” in DNA to relieve topological strain -need to limit digestion to break, on average, one phosphodiester bond per DNA molecule. Otherwise, will just chew up (hydrolyze) DNA DNase I can break the phosphodiester bond on one strand of the DNA 36 DNA topology Ways to relax supercoiled DNA: 2. DNA intercalaters (e.g. Ethidium Bromide): Ethidium is a large, flat, multi-ringed cation Its planar shape enables it to intercalate between the stacked base pairs of DNA Ethidium pushes the stacks apart 37 DNA topology Ways to relax supercoiled DNA: 2. Ethidium Bromide (Etbr): When an ethidium ion intercalates between two base pairs, it causes the DNA to unwind by 26 , reducing the normal rotation per base pair from ~34 to ~10 In other words, ethidium decreases the twist of DNA When Etbr is added to cccDNA, Tw decreases with the addition of each ethidium molecule. Since Lk doesn’t change, then Wr must increase to compensate Etbr 38 DNA topology Ways to relax supercoiled DNA: 2. Ethidium Bromide (Etbr): Addition of Etbr to negatively supercoiled DNA will increase Wr and relax supercoiled DNA. Adding more Etbr will bring eventually introduce positive supercoils to the cccDNA 39 DNA topology Ways to relax supercoiled DNA: 3. Topoisomerases: Topoisomerases can change topology of DNA by transiently introducing single-stranded (ss) or double-stranded (ds) breaks into the phosphate backbone of DNA Topoisomerases cut, move one (or two) DNA strand(s) relative to the other, and re-ligate (re-seal) DNA à this will change linking number Generally classified as Type I or Type II topoisomerases: Type I topoisomerases only cut one DNA strand, do not require ATP, and change Lk by increments of 1 Type II topoisomerases cut both strands of DNA, require ATP for the reaction, and change Lk by increments of 2 40 DNA topoisomerases Example of a Type I DNA topoisomerase reaction: 1 1 2 assuming Wr = -4 3 2 4 3 assuming Wr = -4 assuming Tw stays the same now Wr = -3, and Lk = n + 1 41 DNA topoisomerases Example of a Type II DNA topoisomerase reaction: need ATP cut both strands assuming Wr = -4 assuming Wr = -4 now Wr = -2, and Lk = n + 2 42 DNA topoisomerases Another example of a Type II DNA topoisomerase reaction: Wr = +1 now Wr = -1, therefore, Lk = n - 2 43 DNA topoisomerases DNA topoisomerases do more than relaxing supercoils: In addition to relaxing supercoiled DNA, topoisomerases promote several other reactions important for maintaining proper DNA structure Topoisomerases can catenate and decatenate circular DNA. The ability to decatenate DNA is critical for resolving DNA structures produced from DNA replication Topoisomerases can also untangle DNA knots that form in cells 44 DNA topoisomerases Mechanisms of DNA topoisomerases: Both Type I and Type II topoisomerases use a covalent protein-DNA linkage to cleave and rejoin DNA strands DNA cleavage occurs when a tyrosine residue in the active site of the topoisomerase attacks a phospho-ester bond in the backbone of the target DNA This attack generates a break in the DNA, whereby the topoisomerase is covalently joined to one of the broken ends via a phospho-tyrosine linkage, and the other end is held in place non-covalently by another part of the enzyme covalent linkage 45 DNA topoisomerases Mechanisms of DNA topoisomerases: Type I topoisomerases can be further subdivided into Type IA and Type IB: Type IA form covalent linkages to the 5’ end of the DNA break, whereas Type IB form covalent linkages to the 3’ end of the DNA break Type II topoisomerases have 2 subunits (usually dimers) and each subunit form covalent linkages to the 5’ ends (or 3’ ends) of the ds DNA break A 5’ 3’ 5’ 5’ 3’ 3’ 5’ 3’ 46 DNA topoisomerases Classification of DNA topoisomerases: Both Type I and Type II topoisomerases can be further subdivided into Type IA, Type IB, Type IIA, and Type IIB These are found in prokaryotes, eukaryotes, archeal bacteria and some viruses 47 DNA topoisomerases Mechanisms of DNA topoisomerases: The transient cleavage allows passage of one (or two) DNA strands through the break At the DNA level: 48 DNA topoisomerases Mechanisms of DNA topoisomerases: Since the phospho-tyrosine linkage conserves the energy of the phospho-ester bond that was cleaved, the DNA can be resealed simply by reversing the original reaction No ATP required 49 DNA topoisomerases General features of Type IA topoisomerases: Function as monomers Form covalent bonds between 5’-phosphate of DNA and tyrosine of the enzymes Only Relax –ve supercoils Need Mg2+, but not ATP Change Lk in steps of 1 E.g. E. coli Topoisomerase I Typical structures resolved by Type IA topoisomerases 50 DNA topoisomerases Proposed mechanism for E. coli Topoisomerase I: Green globular domain contains the Tyr that becomes covalently attached to the 5’ end of the nicked DNA strand Red and yellow domains non-covalently hold on to the 3’ end of the nicked strand Other parts of the protein pull the intact strand through the break and reversal of the nucleophilic attack reseals the broken DNA strand and releases the Tyr and enzyme 51 DNA topoisomerases General features of Type IB topoisomerases: Function as monomers Form covalent bonds between 3’-phosphate of DNA and tyrosine of the enzymes Relax +ve OR –ve supercoils Does not require Mg2+, nor ATP Change Lk in steps of 1 E.g. Human Topoisomerase I Typical structures resolved by Type IB topoisomerases 52 DNA topoisomerases Proposed mechanism for Type IB Topoisomerases: Eukaryotic TopI-mediated DNA relaxation by controlled rotation In contrast to type IA or II enzymes, this reaction does not require energy cofactor or divalent metal TopI tends to bind DNA crossovers (supercoils) and nicks DNA by transesterification. The enzyme then allows the DNA to swivel by controlled rotation (i.e. allow one duplex end to rotate around the intact strand) Upon DNA alignment by base pairing and stacking across the nick, the DNA 5’ OH removes the tyrosyl linkage by reverse transesterification 53 DNA topoisomerases General features of Type IIA topoisomerases: Function as dimers (can be homodimers or heterodimers) Cleave opposing strands with a 4 bp stagger Form covalent bonds between 5’-phosphate of both DNA strands and tyrosine of the enzymes Need Mg2+ and ATP Lk of DNA change in steps of 2 E.g. Human Topoisomerase II Typical structures resolved by Type II topoisomerases 54 DNA topoisomerases Proposed mechanism for Type II Topoisomerases: Two-gate model of Topo II: Upper gate opens to admit a DNA duplex. The G-segment (Gate) is transported to the enzyme’s core where it is bent ATP binding promotes the capture of a second DNA duplex, the T-segment (Transported), and dimerization of the ATPase domains Closure of the ATPase domains stimulates cleavage and opening of the G-segment After the T-segment is transported through the break, the G-segment is religated, and the T-segment is expelled through the opening of a lower gate 55 DNA topoisomerases E. Coli DNA gyrase is a specialized Type II topoisomerases: Only enzyme that introduces negative supercoils The formation of –ve supercoiled DNA implied that strand passage by gyrase must be directional Directionality of strand passage by gyrase is accomplished by wrapping DNA around the enzyme in a right-handed bend of 135 bp No equivalent of gyrase has been found in higher organisms (e.g. eukaryotes) NOTE: DNA gyrase and reverse gyrase have opposite activities, but reverse gyrase is a type I topoisomerase Typical structures resolved by E. coli DNA Gyrase 56 DNA topoisomerases Proposed mechanism for DNA gryase: Extra globular domains around which DNA is wrapped around Mechanism is almost the same as for other Type II Topoisomerases However, DNA is wrapped around the enzyme, and the G-segment and T-segment are almost contiguous 57 DNA topoisomerases A different view of how DNA gryase adds negative supercoils: This reaction changes the linking number by -2 (adding 2 supercoils) 58 DNA topoisomerases Clinical uses of topoisomerase inhibitors: Topoisomerases are essential enzymes – mutations of genes encoding topoisomerases are usually lethal Because DNA gyrase is only found in bacteria à excellent target for antibiotics For example, coumarins and quinolones are two classes of drugs that target DNA gyrase through different mechanisms: Coumarins inhibit the ATPase activity of gyrase à block gyrase acitivity Quinolones prevent topoisomerase from religating DNA after cleavage à leave cells with double strand DNA breaks that, if unrepaired, will lead to cell death 59 DNA topoisomerases Clinical uses of topoisomerase inhibitors: Eukaryotic topoisomerases have been exploited as molecular targets for a variety of anti-cancer drugs Camptothecin: Inhibitor of human Topo I (Type IB) Binds to the enzyme-DNA complex, disrupts the alignment of the cleaved DNA ends, and prevents efficient religation of the cleaved DNA Camptothecin induced DNA breaks are converted into double strand DNA breaks during DNA replication. Therefore, it converts Topo I into a DNA damaging agent Preferentially target cancer cells that have high proliferation rates As a side note: there are no known Type 1A Topo inhibitors 60 DNA topoisomerases Clinical uses of topoisomerase inhibitors: Camptothecin (and derivatives such as Irinotecan): Passage of DNA replication machinery converts ssDNA break to dsDNA à death 61 DNA topoisomerases Clinical uses of topoisomerase inhibitors: Anti-cancer drugs that target human Topo II (Type II) are some of the most widely used chemotherapeutic agents Include doxorubicin, etoposide, mitoxantrone etc Two types: topoisomerase “poisons” or topoisomerase catalytic inhibitors Topo II poisons increase the steady-state levels of the transient DNA breaks caused by Topo II. This is the result of either increasing the rate of DNA cleavage, or inhibiting the rate of DNA religation Much like the Topo I inhibitors, these drugs convert Topo II into a DNA damaging agent, leading to cell death In contrast, Topo II catalytic inhibitors inhibit the activity of the enzyme, and prevent decatenation of chromosomal DNA strands prior to mitosis, again eventually leading to cell death Choice of topoisomerase poisons or catalytic inhibitors for cancer chemotherapy depends on whether cancer cells have high or low levels of topoisomerases 62 DNA topoisomerases Clinical uses of topoisomerase inhibitors: Type II topoisomerase inhibitors: 63 DNA synthesis and replication DNA synthesis and replication: Watson and Crick’s quote: “It has not escaped our notice that the specific pairing we have postulated immediately suggests a possible copying mechanism for the genetic material.” Copying mechanism = DNA replication Moreover, predicted a semi-conservative method of replication 65 DNA synthesis and replication: Topological concern: Other scientists, Delbruck in particular, raised concerns over the topological constraints of DNA replication à i.e. how to untangle DNA after replication. Delbruck proposed an alternative “breakage-and-reunion” model. 3 formal models of DNA replication 66 DNA synthesis and replication: Meselson and Stahl: Set out to test these models by using non-radioactive “heavy” isotope (15N) to label parental DNA and density gradient centrifugation to separate light vs heavy DNA. Mix DNA with Cesium chloride solution, spin at very high speed à naturally form density gradient. = isopycnic centrifugation 14N/14N 15N/14N 15N/15N 67 DNA synthesis and replication: Meselson and Stahl’s experiment (1958): E. Coli doubles in 20 mins: 14 N: 68 DNA synthesis and replication: Meselson and Stahl’s experiment (1958): Proved that DNA replication occurs in semi-conservative fashion. John Cairns called it “the most beautiful experiment in biology”. 69