BIOL 366 Lecture 3: Protein Structure & Purification Lecture Notes PDF
Document Details
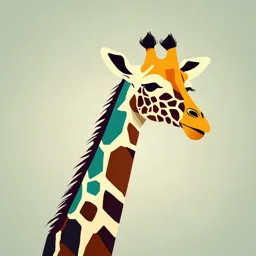
Uploaded by NeatGrace
Tags
Summary
This document is lecture notes on protein structure and purification. It covers topics like the central dogma, protein and nucleic acid structures, and discusses various protein classes. The lecture notes include figures, diagrams, and questions, and might be useful for undergraduate-level biochemistry courses.
Full Transcript
BIOL 366 Lecture 3 (Protein Structure and purification) Readings: • Text sections 4.1 – 4.3 of the text • Separation techniques: Chromatography (by Ozlem Coskun) Crystal structure of bovine coronavirus hemagglutinin-esterase and MERS-CoV S structure Source article: Evaluation of Coronavirus Familie...
BIOL 366 Lecture 3 (Protein Structure and purification) Readings: • Text sections 4.1 – 4.3 of the text • Separation techniques: Chromatography (by Ozlem Coskun) Crystal structure of bovine coronavirus hemagglutinin-esterase and MERS-CoV S structure Source article: Evaluation of Coronavirus Families & Covid-19 Proteins: Molecular Modeling Study 1 DOI: https://doi.org/10.33263/BRIAC105.60396057 Review: • Recall the central dogma of molecular biology: DNA → RNA → Protein • So far we discussed: - Structure of nucleic acids Natural and Chemical synthesis of nucleic acids Extraction and purification and quantitation of nuclei acids Brief utility in biotechnology of nucleic acids • Today we will discuss protein synthesis, structure and purification Text Fig. 24 2 Covid-19 proteins and their structure (Monajjemi et al., 2020) 1. What are elements of protein structures? 2. Why do proteins form structures they have? One of Covid-19 proteins. Fig. 5, Monajjemi et al., 2020. Fig. 1, Huang, 1996. (Figure 1. Huang, 1996) 3 Proteins are made up of amino acids. The General structure of amino acids. • In amino acids, a central carbon atom (Cα) bonds to an amino group, a carboxyl group, a hydrogen, and a side chain (R). • A covalent bond can form between the “C” from COO- of one aa and the N from another to produce a “peptide bind” Text Fig. 4-2. Proteins (or polypeptides) • Proteins are polymers of amino acids • Peptide bonds connect the amino acid residues in the polypeptide chain • Polypeptide chains are directional, with a free amino group at the N-terminus and a free carboxyl group at the C-terminus. Text Fig. 4-4c KEY CONVENTION: When an amino acid sequence is given, it is written and read from the N-terminus to the C-terminus, left to right. 5 Detailed structures of the 20 common amino acids found in proteins. Notes: • Charges shown are true at pH 7.0. • COO, N and H groups are common to all amino acids . • Each amino acid has a unique R group. • Amino acids are grouped based on their properties of the R groups 6 Nonpolar, Aliphatic R Groups - Have hydrocarbon chains (–CH2–); are nonpolar and quite hydrophobic - Tend to cluster inside proteins and stabilize the structure through hydrophobic effects. Notes: - Gly is nonpolar, but contributes little to hydrophobic effects. Why? - Pro limits possible structural conformations. Why? 7 Polar, Uncharged R Groups: - Interact extensively with water through hydrogen bonds. - Two Cys residues brought in close proximity may be oxidized to form a disulfide bond. 8 Polar, Charged R Groups: - Two are negatively, and three are positively charged - Can form H-bond with water - Can form ionic interactions with amino acid of opposite charge. 9 Nonpolar, Aromatic R Groups: - Are generally hydrophobic, and impart hydrophobicity - Phe is the most hydrophobic of these - The OH group of Tyr, and the N group of Trp can form H-bonds and impart some polarity to these residues. 10 A few notes on amino acids in proteins: • AA with Hydrophobic R groups are normally buried inside the protein core: Ala, Ile, Leu, Met, Phe, Val, Pro - Gly is an exception. Due to H as R group, it can be found on the surface as well. • AA with polar R groups (charged or not) are usually found on the surface as well. These are Ser, Thr, Cys, Asn, Gln, and Tyr. • AA with amphipathic R groups are often found at the surface of proteins • AA with charged R groups can form salt bridges: Arg, Lys, Asp, Glu • Cys can form disulfide bridges Fig 4-10 of Text 11 Four levels of structure in proteins. ▪ Proteins must fold to form various sites (e.g., active site, cofactor binding site, etc.) to become functional. ▪ Protein have a “native conformation”, maintained by both strong (e.g., disulphide bonds) and weak (e.g., hydrogen bonding) forces Fig 4-1 13 Primary protein structure. • The aa sequence is the primary protein structure. • Folding creates additional levels. 14 Secondary Protein Structures • Secondary structure refers to a local spatial arrangement of the polypeptide chain The figure shows two secondary structures (circled red) within the tertiary structure of a folded protein. (Adapted from Text Fig 4-11) 15 Secondary Protein Structures Three regular arrangements are common: I. The helix II. The sheet III. Turns A loop Notes: a) Portions of the protein molecule that do not have a “pattern” and connect two regular secondary structures are known as “loops”. b) Part of the polypeptide in which amino acid arrangement is random and irregular is called the “random coil”. They are found in loops and terminal ends. 16 I: The α helix. ▪ The helix is stabilized by intrachain hydrogen bonds (a) ▪ Helices may be left- or right-handed (b) FIGURE 4-6b FIGURE 4-6a ▪ The helix has an electric dipole established through intrachain hydrogen bonds of the amino and carbonyl constituents of each peptide bond (a) 17 II- The β sheet FIGURE 4-8 The β sheet is another component of protein secondary structure. There are two types: (a) Antiparallel: The β strands are in opposite “N to C” orientations. i. Requires 3 – 4 aa (b) Parallel: The β strands are parallel “N to C” orientation i. Requires longer aa loop 18 III. Turns • Due to folding space limitation within folded proteins, sheets and helices often make reverse turns (Turns). • There are two major turns - The The β turn - The γ turn 19 Turns: I. The β turns About β turns: • Hydrogen bond forms between amino acid residues 1 and 4. • There are various types, classified based on the angles between the inner two amino acid residues. • Most β turns contain Pro at position 2 and Gly at position 3. FIGURE 4-9a. - The small R group (H) in Gly allows it to accommodate many conformations. 20 Turns: II: The γ turn: - Is much less common than β turns - Is made up of three amino acids - The first and third amino acid residues form a hydrogen bonds FIGURE 4-9b. 21 Protein Tertiary Structure: Is referred to the three-D orientation of all different secondary structures as well as the turns and loops that connect them. Fig 4-11 a There are two major classes of proteins: Globular proteins (e.g., hemoglobin / myoglobin) • May have multiple secondary structures • May be water-soluble (all hydrophilic OR hydrophilic surface & hydrophobic interior) • May lipid-soluble membrane protein • Have mostly functional roles, e.g. enzymes Fibrous proteins (e.g., keratins, collagen, Silk Fibroin) • typically elongated and insoluble in water • made from a single secondary structure • often have structural roles 22 Domains (in proteins): • Independent, stable folding units within a protein • Contain multiple supersecondary structural elements also known as Motifs or Folds Example: • DNA-binding domain • Catalytic domain • Substrate-binding domain • Etc. Proteins can have one or more domains, depending on size. (a) One domain: sperm whale myoglobin. (b) Two domains: human γ crystallin. (c) Three domains: Aquifex aeolicus DnaA protein (a Gram-negative bacterium) Fig 4-11 a-c Different domains can have different activities within a protein: • E. coli Pol I Klenow fragment has 4 domains • Domains 1, 2, and 3 have DNA polymerase activity • Domains 4 has exonuclease activity Fig 4-11 d Supersecondary structures (motifs/folds) of antiparallel β sheets. The motif can also contain β turns, or γ turns, or both. Examples of more complex motifs: (a) The β-α-β motif contains two parallel β strands connected by an α helix. (b) The α/β barrel domain, which is made of four β-αβ motifs interconnected by α helices. Some motifs are made of only α helices (see Fig 4-16 ac) Example 1: the helix-turn-helix motif - Found in proteins that bind specific DNA sequences - Commonly found in bacterial and eukaryotic transcription factors Fig 4-16 a What amino acids are common in secondary structures? FIGURE 4-7: The relative frequency of amino acids in secondary structural elements. The plot shows the observed relative frequencies of the 20 common amino acids in three types of Quaternary structure: The connections between two or more polypeptide chains. (e.g., association of two identical subunits) FIGURE 4-10: Different representations of tertiary and quaternary structure of the E. coli DNA polymerase β subunit. 30 Proteins are often studied by column chromatography. • One common method is High-Pressure Liquid Chromatography (HPLC). Protein mixtures are dissolved in a liquid and forced (using high pressure) through a column than contain a particular matrix. Reference: Separation techniques. 32 Examples of HPLC i) Ion-exchange chromatography - Relies on the interaction of the protein with a charged matrix in the column ii) Gel/Size-exclusion chromatography - Relies on movement of protein based on its size Proteins can also be separated from one another by Affinity Chromatography - Does not require much pressure - Relies on the “affinity” of a given protein to another molecule (e.g., His6 Tag) Fig. 1, Highlight 4.1 of Text 33 Proteins are often studies by Sodium Dodecyl Sulfate– Polyacrylamide Gel Electrophoresis (SDS-PAGE). In SDS-PAGE: • Proteins are separated from one another based on size (How???) • Proteins are visualized by staining with a dye such as Coomassie Blue Fig. 2, Highlight 4.1 of Text 34