Lecture 3 Inheritance of Qual-Quanti Traits PDF
Document Details
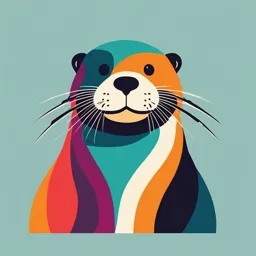
Uploaded by BelievableSelenium
University of Southern Mindanao
Efren E. Magulama, PhD
Tags
Summary
This document provides a lecture on inheritance, focusing on qualitative and quantitative traits, and their interactions with the environment. The document describes Mendelian genetics concepts such as phenotype, genotype, genes, and alleles, and also introduces the concepts of independent assortment, monohybrid crossings, and the law of independent assortment.
Full Transcript
Inheritance and Genetic Improvement of Quantitative Characters Efren E. Magulama, PhD Professor VI Department of Crop Science College of Agriculture University of Southern Mindanao Trait Expression and Sources of Variation The phenotype of an...
Inheritance and Genetic Improvement of Quantitative Characters Efren E. Magulama, PhD Professor VI Department of Crop Science College of Agriculture University of Southern Mindanao Trait Expression and Sources of Variation The phenotype of an individual is determined by its genotype and the influence of environment, expressed as phenotype = genotype + environment. Phenotype = Genotype + Environment Phenotype – physical or outward appearance of an individual or a group of individuals Genotype – underlying genetic makeup of an individual Environment – any factor related to the growing environment Trait Expression, Sources of Variation Genotypic variation – portion of phenotypic variation which is due to genetic causes, and thus may be transmitted to progeny (e.g., is heritable) Environmental variation – portion of phenotypic variation which is not due to genetic causes and thus is not transmitted to progeny Trait Expression, Sources of Variation Origin of Genetic Variation: due to recombination chromosomal variation mutations genetic engineering Gene editing Qualitative versus quantitative inheritance In general, properties of qualitative and quantitative traits may be characterized as follows: Qualitative versus quantitative inheritance Both qualitative and quantitative traits are objects of plant improvement efforts. Examples of some of these traits include: Qualitative – various color traits many disease resistance traits many insect resistance traits plant height (e.g., “Green Revolution” semid dwarfing genes) Quantitative – yield many quality traits environmental stress tolerance (heat, drought, cold) some disease resistance traits Qualitative Traits Definitions and Concepts: Plant breeders select plants with traits or characters that are superior to those previously found in the population. These traits arise from heritable variations (such as mutation) that can then be "manipulated" by the plant breeder to produce a superior cultivar. When we talk about Qualitative Inheritance or genetics, we are talking about characters for which the phenotypic variation among genotypes is not continuous and can be separated into discrete classes. Definition and Concepts Qualitative traits are also called major genes, single genes etc. Most often, every trait you can think of (such as seed color, height, disease resistance etc.) has a contrasting or alternate form. Thus, we have a distinction between a gene and an allele. A gene is the unit of inheritance for a particular trait. Alleles are alternate forms of a gene. For example, we have a gene that controls seed color in beans. Alternate forms of that gene, or alleles, give us different seed colors, such as brown, black, red, and white. The gene in this case is seed color, and white, black, yellow etc. are the different alleles. Definition and Concepts Each gene occupies a specific place on a chromosome called its locus. Contrasting alleles are located on homologous chromosomes Genes are commonly represented by a letter or combination of letters such as AA or aa or Aa. In Mendelian and qualitative inheritance, we refer to genes as being either dominant or recessive. Definition and Concepts Dominant - a trait that is expressed in a hybrid phenotype to the exclusion of the contrasting (recessive) character. Recessive - a trait that is not expressed when in the presence of the contrasting (dominant) allele. When we use the letter notation, capital letters refer to the dominant allele, and lower-case letters recessive allele. Homozygous Dominant - AA -Heterozygous - Aa -Homozygous Recessive - aa Definition and Concepts One of the tools in manipulating major genes, or simply inherited genes is the Monohybrid Cross. Monohybrid Cross = A cross involving segregation of Only a single pair of alleles at a given locus. (One trait) To demonstrate the principles of a monohybrid cross, we'll examine the example below. Definition and Concepts Crosses often involved more than one single pair of alleles. A cross that involves two pairs of contrasting traits, is a dihybrid cross. The dihybrid cross is a simple extension of the monohybrid cross. This is true because of the Law of Independent Assortment which states: “During gamete formation, segregating pairs of unit factors(alleles) assort independently of each other.” What this law is saying is that, for instance, if we take two traits, flower color and flower size, the chance of any plant having large or small flowers is not at all influenced by the color of the flower. Predicting Genetic Ratios Based on Mendel's Laws of Independent Assortment and segregation for individual loci, phenotypic and genotypic ratios can be predicted. Formulas: Number of phenotypes: 2ⁿ, where n is the number of segregating gene pairs assuming complete dominance. -Monohybrid Cross = 2 phenotypes -Dihybrid cross = 4 phenotypes -Trihybrid Cross = 8 phenotypes and so on Predicting Genetic Ratios Number of Genotypes: 3ⁿ, again where n is the number of segregating gene pairs. -Monohybrid Cross = 3 genotypes such as AA, Aa, aa -Dihybrid Cross = 9 genotypes -Trihybrid Cross = 27 genotypes Minimum number of individuals to obtain the homozygous recessive individual: 4ⁿ, where n is number of segregating gene pairs. -Monohybrid Cross = 4 -Dihybrid Cross = 16 -Trihybrid Cross = 64 Predicting Genetic Ratios Continued Determining the phenotypic ratio of a dihybrid cross: Key is recognizing independent assortment and using the product law. The Product Law = When two INDEPENDENT events occur simultaneously, the combined probability of the two outcomes is equal to the product of their individual probabilities of occurrence. Let's use Mendel's famous dihybrid cross of Yellow, Round (GGWW) peas and Green, Wrinkled (ggww) Peas Predicting Genetic Ratios Continued F1: Yellow, Round x Yellow, Round The probability of each plant being yellow or green is INDEPENDENT of the probability of the plant being round or wrinkled. Predicting Genetic Ratios Continued To determine the genotypic ratio, we must recognize that it results from the combined matings of two INDEPENDENT, heterozygous gene pairs (Gg x Gg and Ww x Ww). And from our monohybrid cross, we know that the genotypic ratio for a single gene pair is 1:2:1. So from this we can deduce the genotypic ratio. Predicting Genetic Ratios Continued Genotypic ratio of a dihybrid cross involving pea color and morphology And we arrive at a genotypic ratio of 1:2:1:2:4:2:1:2:1 for the dihybrid cross. Predicting Genetic Ratios Continued Predicting Genetic Ratios Continued What is the genotypic ratio of a trihybrid cross? Use the formula 3ⁿ to determine the number of genotypes you should have. Then, use the forked diagram as above, and you should arrive at the correct answer! Four Gene Pairs The Testcross The testcross is a technique used by plant breeders to help determine the genotype of a particular plant. Recall from our monohybrid cross, that 3 barley plants were hooded. We know that 2 were heterozygous, Kk. To determine which plant is homozygous, KK, we perform a test cross A test cross is always done with a tester that is homozygous recessive, in this case, kk. The Testcross So we take our three plants (ideally) from our monohybrid cross and cross each one to the tester kk. Testcrosses are also used in dihybrid and trihybrid crosses as well. The Progeny Test The progeny test is another basic tool used by plant breeders to determine the genotype of plants they are working with. This time, we do not use a tester, we simply allow the F2 individual to self, then we examine the F3 generation for segregation. If the individual was homozygous dominant, KK, all the F3 progeny from that plant should be KK, hooded. However, if the individual was heterozygous, selfing will produce our familiar 3:1 phenotypic ratio of hooded: awned plants, i.e. we will see segregation in the progeny for the trait of interest in the F3 generation. The Progeny Test The progeny test is also often used to prove that a trait is indeed a single, major gene that is inherited in a simple Mendelian fashion. Modification of Mendelian Ratios Traits may be single gene but not segregate according to Mendelian ratios. Incomplete or Partial Dominance: Observed when the offspring generated by parents with contrasting traits is of an intermediate phenotype. Example = Flower Color in Snapdragons Modification of Mendelian Ratios So, we get the normal 1:2:1 genotypic ratio from a monohybrid cross, but not the expected phenotypic ratio 3:1. Modification of Mendelian Ratios When two alleles are responsible for the production of two, distinct, detectable gene products, we get a situation called codominance. Codominance is different from incomplete dominance. Recall, incomplete dominance leads to an intermediate phenotype in the heterozygote. In codominant situations, both alleles express their gene products in the heterozygote. Example: Flower Color Flowers of two distinct colors are often the result of a codominant situation. Modification of Mendelian Ratios Multiple Alleles: It should be clear to you by now that we can have more than two forms of a gene. Example = Seed Coat Color in beans. Although a diploid organism, such as a bean plant, can only possess two alleles of a gene, in a population of bean plants the total number of different alleles for Seed Coat Color is quite large. The existence of these many alleles for a single gene is called multiple allelism, and the set of alleles itself is called an allelic series. Modification of Mendelian Ratios Within the allelic series, each member can show any type dominance to another member. Example = Clover Chevrons There are 7 alleles that determine "chevron“ pattern on the clover leaf (genus = Trifolium) v, V1, Vh, Vf, Vba, Vb, Vby Looking at the phenotypes, we can list the allelic series for the chevron pattern in Clovers. Epistasis: Often genes (gene products) interact together to produce the phenotype of an organism. Example: Hilum Color of Soybean Seed involves the interaction of 5 genes. Epistasis = The interaction of genes at different loci that affect the same character. Genes involved in epistasis are inherited independently but interact to form one phenotype. Epistasis: Epistasis involves the masking or modifying of one gene by another. The gene that does the masking is said to be epistatic The gene that is masked is said to be hypostatic In the soybean example, expression of the W1, T, and O alleles is masked by the recessive allele r in the presence of I, to produce a yellow hilum regardless of the genotype at the O, T and W1 loci. Epistasis: As can be seen, epistasis causes deviations from the phenotypic ratio in the F2 of 9:3:3:1 that results from segregation of two independent genes, with complete dominance. There are many forms of epistasis. Epistasis Forms: Complementary Action - Two nonallelic genes interact of produce a single phenotype. Example: Rust genes in Oats. AB = resistant, Ab, aB, ab = susceptible Epistasis: Modifying Action: One gene produces an effect only in the presence of a second gene at another locus. Example: Aleurone color in corn. PrR = purple, prR = red, Prr, prr = colorless. Inhibiting Action: One gene inhibits the expression of another gene. Example: Corn = Ri = red, RI, rI, ri = white Masking Action: One gene hides the effect of another when both are present. Example: Oat seed coat Color BY, By = black; bY = Yellow; by = white Epistasis: Duplicate Action: Either of two genes may produce the same effect, or the same effect is produced by both of them together. Example = common shepherd's purse seed capsule Cd, cD, CD = triangular shaped; cd = ovoid shaped Additive Effect: Two genes produce the same effect, but when together, the effects are additive. Example: Barley awn length Ab, aB = medium length; AB = long awns, ab = awnless. How do these different forms of epistasis affect the genotypic ratio? Epistasis: Sometimes, a single gene may affect the expression more than one phenotype. These genes are called pleiotropic. Pleiotropic Genes = Genes that control the expression of more than one trait. This is a common occurrence. Usually, you have one gene that produces an enzyme that is involved in multiple biochemical pathways with each pathway leading to a unique phenotype. Example = 'uzu' gene in barley. In its recessive condition, uzu may shorten stem and rachis internodes, reduce seed size, and produce an erect coleoptile leaf. Epistasis: Phenotypes that involved epistasis can be difficult to manipulate for the plant breeder for obvious reasons. Linkage: So far, we've just been dealing with genes that are inherited independently of one another. Often these genes are located on different chromosomes or very far apart on the same chromosome. However, one can imagine a situation when two genes are right next to each other on the same chromosome causing them to be inherited together like a single gene. This can be problematic for the plant breeder. Linkage: For example, let's say you have a gene that you know increases sugar content in grapes. A grape breeder notices, however, that just about every grape variety with this increased sugar content also has increased susceptibility to a certain fungal pathogen, downy mildew. This type of situation occurs often in plant breeding where a trait of interest is linked to a trait, we would like to get rid of. Fortunately, through the process of crossing over, recombination of linked genes can occur. Linkage: Crossing Over = process by which segments of chromatids of homologous chromosomes are exchanged as they synapse during meiosis. The crossover value for any two genes is equal to the percent of recombination between them. The greater the distance between the two genes, the greater the percentage of recombination. Mather 1951 developed one way to calculate recombination frequency: Linkage: Two loci are considered independent if the recombination percentage between them is 50%. Recombination frequency also referred to as the number of map units between the two loci in map units (centimorgans), i.e. 15% RF is a distance of 15 cM. Linkage: Genes can be linked in Coupling or Repulsion. Coupling = present when the dominant or favorable alleles at two loci are on one chromosome, and the recessive or unfavorable alleles are on the other, AB/ab. Repulsion = present when a dominant or favorable allele at one locus is on the same chromosome as a recessive or unfavorable allele at another locus, Ab/aB. How can we determine if our genes are linked in coupling or repulsion?? Linkage: Procedures for evaluation of linkage: 1. Cross an individual that is heterozygous at two loci AaBb to a homozygous recessive tester, aabb. If the two loci are not linked what will the genotypic frequency of our progeny be? If the two loci are not linked, the genotypic frequency of the progeny should average 1/4 AaBb. 114 Aabb, 114 aaBb, and 1/4 aabb. If the loci are linked, the frequency of the four genotypes will deviate significantly from the 1: 1: I: 1 ratio. 2. Self-Pollinate individuals that are heterozygous at two Loci. If there is complete dominance at each locus and no epistasis, the average ratio of the progeny will be 9A_B_: 3A_bb: 3 aaB_:1 aabb Linkage: Linkage in coupling or repulsion will cause significant deviations from the 9:3:3:1 ratio. (Fehr Table) Another statistical test that we use to confirm whether our not our data occurred by chance, is the Chi-Square test. The chi-square is a statistical test that examines whether any deviations from an expected ratio occurred to due to chance. Testcross Quantitative Traits Johannsen's Studies: genetic and environmental factors affect expression Nilsson-Ehle's Studies: multiple genes affecting a single trait Types of Gene Action Additive effects Dominance effects Epistasis effects Overdominance effects Quantitative Traits Components of Phenotypic Variation Analysis of quantitative traits is generally done using statistics, where means and variances of test populations are examined. Partitioning of variances in test populations allows estimation of the degree to which phenotypic variation is due to genetic variation, environmental variation, and genotype by environment interaction. Phenotype = genotype + environment + genotype * environment interaction VP = VG + VE + VGE VP = (VA + VD + VI) + VE + VGE VP = VG + VE + VGE VP = (VA + VD + VI) + VE + VGE VGE= genotype by environment interaction the proportion of phenotypic variance that is due to the inconsistency of genotypic expression in different environments. VA – additive genetic variance the proportion of genetic variance that is due to additive effects of alleles at loci controlling the quantitative trait VD – dominance genetic variance the proportion of genetic variance due to transient interactions between alleles at loci controlling the quantitative trait VI – epistatic genetic variance the proportion of genetic variance due to interactions among alleles at difference loci controlling the quantitative trait The effectiveness of selection efforts in plant breeding (e.g., for improved yield, quality, or environmental stress tolerance) depends on the degree to which phenotypic variability is controlled by genetic variation as opposed to environmental effects. Heritability and Selection Response Heritability – a measure of the degree to which the phenotype is controlled by genetic factors and thus amenable to genetic improvement in breeding. Two main types of heritability: 1. broad sense heritability: (VG/VP) x 100 The proportion of phenotypic variability that is due to all types of genetic causes. 2. narrow sense heritability: (VA/VP) x 100 The proportion of phenotypic variability that is due to heritable genetic factors (e.g., may be passed from parents to progeny). Heritability and Selection Response Principal uses of heritability 1. determine the relative importance of genetic effects in expression of a character. 2. to evaluate the relative effectiveness of different selection procedures. 3. to estimate the expected gain resulting from selection (genetic advance). Heritability and Selection Response Genetic gain – the percent improvement in trait expression with a single cycle of selection