Lecture Notes on Genetics PDF
Document Details
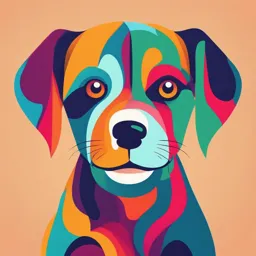
Uploaded by .keeks.
Marian University
Tags
Summary
This document provides an overview of genetic concepts including the differences between qualitative and quantitative traits, and explores the concepts of inheritance, dominance, and co-dominance using examples.
Full Transcript
Okay. It is one o'clock. Let's go ahead and get started. So happy Monday. happy Monday. Yay, nay. We got through exam block three and now we're into block four, right? got through exam block three and now we're into block four, right? Okay. So I like to start this block with some things that feel...
Okay. It is one o'clock. Let's go ahead and get started. So happy Monday. happy Monday. Yay, nay. We got through exam block three and now we're into block four, right? got through exam block three and now we're into block four, right? Okay. So I like to start this block with some things that feel like in a continuation of the past block and then really start hitting home that human message. I looked at some of the things that we were doing and I decided to completely revamp what should have been lecture one. And so now they're in a different order. The order still makes sense. It still makes a logical progression. But what this also means is that some of the more difficult pieces won't be on an asynchronous day. So as we know that Friday is an asynchronous day to encourage you to go to research day and focus on your attention on all those lovely research projects happening here at new WCOM. But I don't like having this complicated inheritance piece be part of that day. So moving things around made much more sense, giving us a much more human focus to everything we're doing moving forward, which was the plan. So I like the plan now. All right. So we have in this section a couple of terms and I like to think of this lecture as all those things we've taught you, here's all the ways we're going to break those rules. So all the ways that we talked about basic inheritance, now we're in people. And people do not lend themselves well to simple concepts of dominance and recessive. So one of the things to think about when we talk about genetic traits in people is we have to remember that there is no real such thing as a monogenic trait. Because none of your traits exist in a vacuum. All of your genetic material is interactive. It is talking to each other. It is engaging with each other. Everything okay? Okay. Just a second. Sorry. IT. They are our friends. Super helpful. So what we have in terms of us is even those traits that technically if you inherit and you will have the phenotype response, the level of response can be different. And so we're going to start from the concept of qualitative versus quantitative traits. A qualitative trait is really variation at a given locus. So we can really think of this as having unexpected representation in terms of Mendelian genetics. In Mendelian genetics, it is all of those ratios that we talked about before. The idea that if you inherit this version, you get this phenotype. And if it is recessive, you need two copies. need two copies. All of that. How do we define dominance under a Mendelian situation? a Mendelian situation? Mendelian a quantitative trait is actually going to be a compilation trait. Many of our human features, height, eye color, hair color, are actually the interaction of multiple genes where intensity of color, any sort of hyper or hypopigmentation is going to be affiliated with varying degrees of interaction across those genes and those gene versions. with varying degrees of interaction across those genes and those gene versions. Does this make sense? this make sense? So it is important to note that unlike our Mendel where we had a white flower and a red flower and all we could get was white or red, we can now get stages of outcomes. And the more genes involved, the more variation we can produce. So if you have five genes, you are going to have a lot more phenotypic outcomes than if you have two. If you have ten genes, you have even more. Complicated interactions. This is one of my favorite type of genetic interactions and it is incomplete dominance where we have variants of the same gene, however, they don't actually have full recessivity or dominance. Instead of having white versus red, you can now have pink where the heterozygote actually induces an in-between or intermittent phenotype. Sort of an amalgam of the two. Keynote here, this is stepping back to variants of a single gene. This is not multiple genes yet. Variants of a single gene. When you have multiple variants that are not capable of masking each other where versions are both held completely visible, you have co-dominance. you have co-dominance. And so what that looks like is imagine a flower that has purple and yellow. It is not a mixture of some weird brown between the purple and the yellow. It is purple and yellow. That is co-dominance. And the human example of that are, of course, our blood groups. This is particularly important in situations where we have more than just two variants of a particular trait. So we know our blood groups are A, B, O, right? So that is three instead of two, traditionally. And we always said before, you can only compare one thing to one other thing. We are still doing that. Your choices are still A, B, AB, or O, right? And any of the A's or B, you could be double A, double B, or A, O, B, O, right? Because really what you are talking about when it comes to those blood groups is what you produce on your surface and what you release, right? This is not new. You did this in immuno, right? immuno, right? So our perspective on this is the genetic interaction. genetic interaction. If you have a gene for type A, you are type A because you are going to be producing material correlated with being type A. You are literally making antigens and antibodies. Does this make sense? sense? When you have a situation in genetics where you are talking about the production of something versus the absence of something, you can get a wide range of phenotypes based on the level of production. You are going to have a much stronger presentation of material from an IAIA instead of an IAO individual. So we do have a wide range of possibilities here. So this is just a nice summary of our both antibody production and presence of antigens. So if we are looking at examples of this and we are trying to figure out how is this variance of a single gene affecting outcomes, we want to look at the product. We have to look at phenotype. to look at phenotype. And so here you can see we have three options and three distinct phenotypes. If the product will ultimately make a structure, it is likely to be presence absence, right? it is likely to be presence absence, right? Full compound that is regulatory, prevents expression, or ensures the activity is something else, that is also going to be presence absence, right? It is when we start getting into situations where we have interactions of pigments or production of a product that is visual in some way, that is going to give you the potential to see both, see a mixture, see neither. neither. Does that make sense? And we can use the paint example to help us understand that, right? So when you mix a really, really dark color with a really light color, sometimes all you can see is a really dark color, right? a really dark color, right? But sometimes when you mix the really dark color with a lot of a really light color, you can lighten the color and get somewhere in between. somewhere in between. Those are examples, the first one of being normal expected dominance, the second of being incomplete dominance. And then if you somehow have two colors you can't mix at all and they both show up, that is co-dominance. Does that make sense? make sense? So this example over here is for an enzyme. enzyme. What do you see? When you are WW, what are you? are you? Big capital W, capital W. Large and round, right? In your heterozygous state, what do you look like? Large and irregular and then in our homozygous recessive state, small and irregular. state, small and irregular. So this, am I seeing both phenotypes or something that is kind of in between? between? It is much more in This is an example of incomplete dominance. Neither trait is fully displayed. fully displayed. It is a mixture of both. Make sense? What does the central dogma of molecular genetics tell us? genetics tell us? That we hated block three? No. No. That DNA exerts its effects indirectly through RNA and protein, but that protein can influence things like gene expression and behavior, right? When it comes to functional consequences, we are playing in the realm of protein and product. And so level of protein, amount of material available, directly correlates with functionality. We have whether or not I make the right protein, of course, but then its availability matters. And we will talk about that a little bit more with additional gene regulation. Part of what we are looking at when we look at dominant disorders, dominant traits or multiple gene interactions is do I have haploinsufficiency? gene interactions is do I have haploinsufficiency? Does having one copy still give me enough function to have a normal or expected phenotype or does losing one copy create an adverse phenotype? And so this is that gene dosage we have talked about so many times in past blocks. I know you have slept since then, but we are still considering that. And it becomes more and more complicated when you add more and more genes to the mixture. Complex and multifactorial traits are easily complicated by genetic variation. How does a variation in one gene impact the overall process? Is it different than a variation in another gene? And we can use our DNA repair example for this if we look at the array of XP proteins, for example. We have a complex that is formed by multiple XP proteins. Some of them are more scaffold in nature. Others are more important in recognition. Losing a recognition molecule is going to have a more significant impact than a scaffold one. Makes sense? So when it comes to that haploinsufficiency, if I lose half the material and I have a phenotype, that is considered a dominant situation. Many of our cancer risk variants fall into this category because what you are inheriting is the risk, the increased risk of the cancer. the increased risk of the cancer. In terms of having an intermediate phenotype, we are going to be looking at whether half gives you sufficient product. Why would a genetic condition that is lethal in the recessive state be perpetuated in a population of humans, or any organism for that matter? So we are an organism that can carry out selective breeding, right? that can carry out selective breeding, right? That is one way in which we can decide what traits we want to perpetuate and that we do not. we want to perpetuate and that we do not. Why would we have historically reached a point where a lethal recessive condition can still occur? What does that imply? In order for there to be lethality, what has to happen? Two carriers have to create an offspring where they inherit both recessive forms of the trait, right? So why would that status continue? would that status continue? Go for it. Yeah, that is a huge part of this. The ability to achieve reproductive age or reproductive fitness is not impacted by the condition. The second part of that is there is a benefit to the heterozygous state. Even when we see some level of phenotype in the heterozygous state, there is some sort of advantageous consequence. advantageous consequence. This is the great example of malaria and sickle cell anemia. Mosquitoes had an incredible selective pressure on us. They are one of the driving forces of our changes, which is just strange, right? But it turns out that being heterozygous for the conditions associated with sickle cell anemia was advantageous, remains advantageous, even though you see cell sickling. And I will throw that caveat out there. We're going to get to it again in a later lecture or later today. But I'm going to throw that caveat. It's not a truly recessive condition, okay? Just throwing that out there. All right. So what does it mean to have genetic variation? We talked about variance, right? We talked about the concept of variation. What does it actually mean? mean? I feel like a lot of people are avoiding my eyes now. my eyes now. What does it mean to have genetic variation? I mean, phenotypically it makes sense, right? Phenotypic variation is literally seeing different things, right? different things, right? Genetic variation is a difference in base sequence. So how does that correlate with variation at the protein level? Well, at the protein level, our variation is going to be structural or melt. And this can lead to a variable outcome in that phenotypic expression. I could theoretically have the same genes as another person, theoretically the same genes variance for a trait and not have the same phenotypic outcome because of the complicated genetic interactions that is me versus that other person. There are shades of brown hair. There are shades of blonde, right? The strawberry blonde. But in theory, the gene could structurally be the same. And so these are the expressivity and penetrance issues. and penetrance issues. I like to think of them as whether and to what degree. Can I see it? That's penetrance. Can it penetrate the surface and be seen? But expressivity is the level of expression. There's a difference between pink and fuchsia, right? right? No, just me. Nobody love the 80s hot pinks out there. Oh wait, I'm old. Sorry, I forgot. Anyway, being able to have the trait is not the same as being able to see the phenotype from the trait. So I can have the genes, but they're silenced by the lack of another gene, right? And this is good in terms of disease states. If I have three genes that are necessary to confer a particular adverse phenotype, I need to have all three for it to happen, and I only have one. I could have a much milder form of the disorder or no phenotype at all. And so when we're looking at pathogenic variation, when we're looking at human disease, we have to pay attention to these wide-ranging specific differences. Overall, this difference in expected phenotype is epistasis. We expect when you cross a heterozygote to a heterozygote, we expect a nine to three to three to one, right? Any deviation in that is epistasis. And there are multiple ways to deviate. This chart sums up all the different ways that we can deviate, but we're not going to delve too much into many of them. We are just going to focus on a few examples. When both show complete dominance, we have actually nine possible dihybrid ratios. And so it's how we are deviating in terms of masking availability that confers those different ratios. So if we kind of toggle back and forth for a second, we have the nine to seven, the 12 to three to one. And we're going to want to use these color boxes to sort of help you see that. So up at the top, you have what the actual genotypes are. And if you look here at the 12 to three to one, you can see that this nine and this three, these genotypes all correlate with the same dominant, dominant phenotype. And so if we look here, where we deviate, what do you see? Just having A is enough to cause that phenotype. Losing the dominant version of A, and it doesn't matter to produce that phenotype. and it doesn't matter to produce that phenotype. Does that make sense? that make sense? If we look at the nine to three to four, which is this one here. Oh, where are you? There you go. The nine to four to three, or the, or the three to four. It just depends on which one you're looking at, which, whether it's A or B. or B. This one here, what do you see? As long as you have a dominant allele of both, you're dominant, dominant, right? dominant, right? But once you lose the dominant allele of one, you have the same phenotype. And there's only another phenotype for the dominant allele being lost of the other. So complex interactions can create epistatic consequences. Make sounds? I see faces, but we're going to move on. We are going to practice that one, I promise. We are going to practice that one, I promise. So if I have traits where it's quantifiable, I've got complex interactions, amounts that I'm paying attention to. I can have what is called phenotypic plasticity, where I can have multiple phenotypes encoded by the same genotype. This very much ties to that idea of whether I can see it and to what degree. If I have to have a pile of X to this height and a pile of Y to this height, and only together can I get this phenotype, then any deviation in those piles, even if they're much lower or much higher, is going to change my phenotype. Does that make sense? So even though X and Y have not been altered, the amount of product is directly influencing the phenotypic outcome. This is some of that translational regulation that we are going to be talking about. What is the main way in which we regulate our genes? Transcriptionally, but it's not the only way, is it? is it? And since we can have post-transcriptional processing, translational regulation, and post-translational modifications all playing a role, we can actually get a wide range of outcomes. That's just from our genetics. Now we get environment and lifestyle coming into play as well. Environmental factors can increase or decrease genetic expression. Exposures to chemicals, we already understand. We can have genetic mutation. We can have base deamination, which can affect DNA and RNA. But we can also just have toxic byproducts of metabolism. We can have metabolic dysfunction. And of course, what is a heritable form of change that doesn't affect sequence? Epigenetics, where we can actually turn on and off and regulate at the level of the DNA and pass that on to future cells or offspring. So this is a great example of how environment and epigenetic signatures come into play. And the best example of that is our hydrangea, blue versus pink. Acidic soil giving you one outcome versus another, which ties directly to epigenetic inactivation of key genes. epigenetic inactivation of key genes. Yes. What was that? was that? Sorry, that's from the recording. I apologize. apologize. Okay. So we want to move on to humans, but finishing up the epigenetic piece. Lifestyle and environment can mitigate or enhance an adverse phenotype. If I have many positive environmental and lifestyle experiences, I can actually reduce the phenotypic expression of certain traits, right? I can mitigate the adverse outcomes of a sickle cell by not having certain levels of activity where it could plummet my oxygen, right? The answer to that is yes, that can happen. But that is just one example of a lifestyle change where you control and regulate exercise to ensure that you are not causing oxygen depletion or cell accumulation, right? No, I lost you. Okay. Other epigenetic considerations can actually improve a phenotype, right? improve a phenotype, right? Where I have inherited a risk for cancer, so I avoid carcinogens. I eat healthy. I exercise. Those can help lower my risk. So even though I have this inherited predisposition, I can somewhat mitigate some aspects of that. And so all of human genetics is a complex interaction of environment, lifestyle, and genetics. So let's talk about pedigrees. Pedigrees are how we study people. This is not pre-sequencing. This is very much what we do as a first line to decide if you qualify for testing. You start by looking at a family history. A pedigree is just a drawing of a family history. Things to pay attention to is this is an evolving representation. We have many different shapes and structures to be more encompassing of the human experience, but there are a couple of rules that are important. Matings between relatives are represented by double lines because that consanguinity is an important consideration. All pedigree analysis is a risk assessment, and much of the risk assessment is based on Mendelian inheritance. And as we just mentioned, much of human inheritance is not fully Mendelian. So this is always a first-stop analysis. When we do these analyses, we have rules. When we do these analyses, we have rules. Why do you think it's important if you're looking for a familial inherited trait? Why would we need three generations? three generations? What do we think? Go for it. Great. So that's actually one of the best answers for that because recessive traits, by their nature, what happens to a recessive gene if you are not homozygous recessive? It's masked by the heterozygous state, the definition of recessive, right? So what would that look like if you just did a pedigree of one person? Like, oh, that was my parents, and here I am. There would be no way of knowing. You would just assume de novo, right? You'd assume it's you because your parents don't have it. But if you look back to their parents and someone in that generation has it, then you know it's fully recessive. Does that make sense? It gives you the ability to identify what the risk to the next person is. What's the risk of you being a carrier for a recessive? In the case of something like Huntington's, a dominant, what's your risk of having inherited the dominant allele? So when it comes to analyses and things like cancer, you're actually really interested in age of onset. And also, Huntington's becomes a good example of that. The earlier a condition manifests, the more likely you are to have inherited a more significant pathogenic variant. So in the case of Huntington's, being homozygous dominant, because it is a dominant condition, is far worse and has an earlier correlation of disease manifestation, earlier age of onset, than the heterozygote. So those are important assessments you want to be able to make. In terms of who we are going to look at, we are really going to look at first, second, and third degree. First degree relatives are those with the closest relationship you have and up to 50% of the second degree is going to have up to 25% in common. Cousin, aunt, uncle. Third degree relatives are going to have less. Now we are going to divide in half again and get to 12.5%. When you look at a pedigree from that perspective, you are trying to determine what the probability of having inherited the pathogenic variant is. This can get extremely complicated for multifactorial disorders. So starting with monogenic. I have already kind of tried to lay the groundwork of not really a thing. And part of the reason why monogenic is not really a thing is because these are rare and severe. If you have a very clear cut inheritance patterns, chances are, especially for the recessive conditions that have an incomplete dominance, it can have lethality. And so then your entire risk assessment is based on risk of being a carrier. When dominant, and this is why I was so glad that somebody said this, when dominant, it is because you manage to not manifest until after reproductive success. Adult onset, late onset. Developmental concerns that occur as a regression after sexual maturity. Recessive conditions manifest because of that benefit. That somewhere there is an incomplete dominance. There is a beneficial gain to having the allele be present. Truly, monogenic recessive conditions can be incredibly significant in terms of their systemic effects. We only use the word carrier when you do not have a phenotype. If it is a dominant condition, you are not a carrier, you have it. Whether you have it to the same expression or penetrance is irrelevant. You have the allele, you have the risk, you have the condition. Even if you are barely manifesting it. You can only be a carrier if you are not affected by having that allele. You are carrying it without consequences. Make sense? We have some fun little rules. If it is recessive and you are a carrier, your risk of passing it down increases with matings between close relatives. That is consanguinity. First cousins, siblings, these kinds of matings increase the propensity and probability of recessive condition and inheritance within families. condition and inheritance within families. We are going to take a look at the picture first. What do you see? Yellowish white circles are unaffected. Red is affected. Circles are squares. Squares are males. Circles are females. What do you see? What do you see? Let us look at the first line. Generation 1. What do you see? One affected male and a non-affected female. Next line. What happened to their kids? Three of them are affected and their kids. Three of them are affected and their kids. Do we have affected individuals? We kind of got it right. Do you see an abnormal number of males being affected compared to females? Not really. But what do you see in every single generation? But what do you see in every single generation? Affected individuals. That is one of the hallmarks of dominance. Having affected individuals in every generation and having clear, direct patterns of parent to child inheritance. Huntington's disease is our example of this. I do not know why this is clicking out of order because we are just going to roll with it. It was working fine, but now it is not. This happened again last year as well. It is really weird. Huntington's has a lot of the hallmarks of a dominant disorder. It has adult onset. Homozygous dominant individuals have an earlier age of onset. Severe complications. It is very monogenic in that it is rarity and severity. But back to what I was actually trying to do. Dominant appear in every generation. You would expect typically half. If only one of the individuals was affected, half of the offspring. If both individuals were affected, you would have a significant increase in that potential. In this example of generation one to two, three out of the four were affected because each individual offspring is a 50-50 chance. each individual offspring is a 50-50 chance. Does that make sense? So looking at the probability from that one, what was the probability that they would have four children and three out of the four were affected? four children and three out of the four were affected? It is a pretty low probability that an unfortunate one. It is one half times one half times one half. The best way to discern autosomal from *** link is to look at mothers to sons, fathers to daughters. We should see a discrepancy in the sexes when there is a *** linked trait. Huntington's affects both sexes equally. And every single person that we have tested to date has had an affected parent, though the degree of affected could be variable. It is very unusual for this to be de novo. And of course about half of the offspring of an affected individual run the risk. Huntington's disease is actually incredibly rare and it does result in severe cognitive decline. result in severe cognitive decline. Significant impacts on the brain, neurological function, you end up with movement disorders, psychiatric disorders, and it affects about 1 in 10,000 people. There are about 16% of the cases that are considered juvenile Huntington's. That got serious fast, didn't it? That got serious fast, didn't it? Some of these are really not fun. fun. Let's do recessive. What do we see? Sorry, I really should have remembered the trigger warning. I apologize. We doing okay? Recessive, what do you see? How is it different? is it different? Generation one, what do you see? Weird dashed lines that you wouldn't put on a pedigree. a pedigree. It's just an instructional tool. What about the next generation? about the next generation? Two, nobody is affected, right? But then all of a sudden we get to three and we have half the children of one mating and then we have a consanguinity and the next generation affected. and the next generation affected. What can't you tell from this? tell from this? You can't really tell who's a carrier. You have to actually do some considerations and some probability analysis there. What else? What can't you tell? It's really hard to discern *** linked versus autosomal in a recessive condition. It's where you have to pay attention to father to daughter, mother to son. Make sense? In the case of this, we are autosomal recessive and it's going to do the same thing where it brings up an example before we actually talk about the features. What would be nice to have would be some information from these individuals' offspring. Tell me why I would want information from 3-1 and 3-3. and 3-3. What information might be useful? If they are with an unaffected individual, do we have affected offspring? Because if you are a recessive condition, two unaffected individuals can have an unaffected or unaffected offspring, but two affected individuals should not be able to have unaffected offspring. They only have the little allele to pass down. What would it mean if you saw an unaffected individual from two affected individuals? an unaffected individual from two affected individuals? It's a couple of interesting... but also some interesting concepts of penetrance there. More mild forms from the combination of allele variants, but also possible that parentage is questioned. Let's look at my little note there to remind you. If you have a dominant allele, you have it. You're not a carrier. Only carriers exist as a terminology associated with recessive traits. Albinism is a great example of recessivity because you have either no phenotype or the phenotype. It's a very clear cut. You need both copies to be altered to exhibit any of the phenotypic symptoms. That being said, the degree of severity of the albinism is very much tied to what combination of variants you inherit. Most of the individuals who have this condition actually come from carrier parents, but it would still be very interesting in any sort of analysis to be able to look for those, what does the offspring of two affected individuals look like? For conditions that are monogenic recessive, you do tend to see a higher degree of prevalence within the family when consanguinity is present because in order to have the condition, you must inherit both altered copies, and it is more probable to find carriers within the same family. This can also give you this false impression of dominance, so we must always look out for concepts of consanguinity. For those of you who might think this is really uncommon, it is marriages for situations in which you didn't know you were related. Does this make sense? So this is that direct phenotype, carriers. The other example we like to use for this is cystic fibrosis because now we are talking about a specific mutation in a key protein, and so we have the ability to look at this from the perspective of, I have an altered version of this. However, I can mitigate the physical symptoms of the condition by being in places that make it easier to breathe in the presence of this thicker mucus. The CFTR gene is, of course, the one we are interested in, this one, and this abnormal mucus is something that affects multiple systems because every mucus membrane is affected. So we will affect the respiratory, gastrointestinal, and reproductive systems. This is one of the conditions in which we pay attention to carrier rates. Individuals who are carriers for the condition, about 1 in 19 chance for a Caucasian individual to be a carrier. And so if you don't have a family history, that knowledge of carrier incidence helps you to get a better sense of how likely it is for a particular mating to create an individual with this condition as a concern of genetic counseling for prospective parents. This carrier status can, of course, be identified through carrier testing. But if you are not yet qualified for carrier testing, a simple assumption of a 1 in 19 chance can give you a better idea of what your risk is, particularly if the mating is between someone who is not affected by the condition with someone who is. So an individual who is affected with the condition has a what percentage chance of passing on the allele? 100%. It's recessive, right? So you are getting an allele, and now it becomes what is your chance of the other parents passing that on. And if neither knows their risk, then you have a 1 in 19 times a 1 in 19 for a Caucasian family. Does that make sense? Some of those risks are very, very small. Others can get more complicated. Family history can raise those risks. I know I was saying the presumption of the 1 in 19 is because we have a higher than presumed. 1 in 29 is the official. The 1 in 19 is that higher than presumed. I apologize. I apologize. I meant to change that number. Does that make sense? that make sense? Oh, I'm sorry. 1 in 19 is Caucasian. That's 1 in 29. Yeah, it's Monday. 1 in 19 for Caucasians, 1 in 29 for all U.S. citizens. citizens. My apologies. Think that through. So what would that look like for someone with unknown familial inheritance, right? First, how would we have an individual with an unknown familial inheritance pattern? Adoption. So we have an unknown pattern. What would be a useful number? number? In some of those cases, you want to presume the higher number. You want to presume the higher risk so that you can give a worst-case scenario assessment. If the average American is using a number like 1 in 19 for our Caucasian individuals can be much more beneficial. And I do believe some of those numbers have some updates, but these overall incidences should be pretty accurate. pretty accurate. Does that make sense? So incidence of the condition versus carrier status. I want to make sure we were clear. Those numbers are incidence. The carrier status that I was talking about is different. So now we have another dominant condition. How do we know it's dominant? it's dominant? Look at our pedigree. What is sticking out the most in this pedigree? Every generation is affected. Direct lines of inheritance to children where we have matings between affected and unaffected individuals producing affected individuals. Anything else that stands out. It's not really easy to see a discrepancy between males and females here, is it? This looks very much like an autosomal dominant, and so you need to pay attention to mother, to sons, fathers, to daughters. mother, to sons, fathers, to daughters. For dominant, father to daughter tells us a lot more. Every father on here who fathered a daughter did what to his daughters? did what to his daughters? Gave them the condition, right? Look at our generation one. What do you see? Who was affected? The male. What does generation two look like? All of the female offspring are affected. Do we have any alternative options there? No, just all of the females were affected, and that is frequently what we will see. So you're going to look for that father to daughter relationship for an assessment of dominance. When it comes to a dominant condition that is sex-length, affected males cannot have penetrance in expressivity, but they will have the allele. the allele. Males should only be able to show the condition if inherited from, where did they get the X? From mom. And so our example here is X-length lisencephaly. What about recessive? We are out of time. It's just I really got to learn to recess. It's 1350. We are out of time. This happens every time I get into this lecture because I get so excited to talk about this stuff. All right, so we will come back at X-length recessive on Wednesday. Questions or concerns? Aren't we happy this wasn't pre-recorded? Say hi to Dr. Skinner. Thank you. If you have any questions, let me know. But we will remember class does meet Wednesday. Class does not meet Friday.