BIOL371: Microbiology Lecture 3 – Microbial Metabolism PDF
Document Details
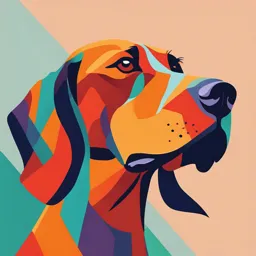
Uploaded by GoldenDeciduousForest
Tags
Related
- Lec 12: Microbial Metabolism & Diversity (PDF)
- Microbial Metabolism & Catabolism Lecture Notes PDF
- BIO-205 Chapter 8 F24 RMB Revisions Version 1 Microbiology OpenStax PDF
- Microbial Nutrition, Ecology, and Growth Lecture Notes PDF
- Microbial Metabolism - Lecture Notes PDF
- BIOL 351 Microbiology Lecture 20 PDF
Summary
This document is a lecture on microbial metabolism. It covers topics such as fundamentals of metabolism, and catabolism by chemoorganotrophs and catabolism by electron transport and metabolism. Suitable for students.
Full Transcript
BIOL371: Microbiology Lecture 3 – Microbial metabolism 1 Topics of today 1. Fundamentals of metabolism 2. Catabolism: chemoorganotrophs 3. Catabolism: electron transport and metabolism Materials covered: Chapter 3.1-3.11 Figures 3.1-3.6, 3.8-3.12, 3.14-3.22 2 1. Fundamentals of metabolis...
BIOL371: Microbiology Lecture 3 – Microbial metabolism 1 Topics of today 1. Fundamentals of metabolism 2. Catabolism: chemoorganotrophs 3. Catabolism: electron transport and metabolism Materials covered: Chapter 3.1-3.11 Figures 3.1-3.6, 3.8-3.12, 3.14-3.22 2 1. Fundamentals of metabolism 1. 2. 3. 4. Defining the Requirements for Life Electron Transfer Reactions Calculating Changes in Free Energy Catalysis and Enzymes 3 What is metabolism Life requires: 1) liquid water, 2) source of energy to do work, 3) source of electrons to perform biochemical reactions, and 4) nutrients to build macromolecules Metabolism – the sum total of all chemical reactions needed for life Catabolism – reactions used to break complex molecules to obtain energy Anabolism – reactions used to synthesize cellular materials Relies of electron donors directing electrons to electron acceptors Energy is neither created nor destroyed Cells conserve energy (for growth) by converting energy from their surroundings to a form that do the work By generating energy rich compounds (e.g., adenosine triphosphate (ATP)) that can be released to drive cellular processes 4 Types of metabolism defined by source of energy Metabolic classes of microorganisms Autotrophs obtain carbon from CO2 Heterotrophs obtain carbon from organic matters Chemoorganotrophs (heterotrophic) obtain energy and reducing power from organic matter Chemolithotrophs (autotrophic) obtain energy and reducing power from inorganics 5 Energy conservation and electron flow Catabolism uses exergonic (release of free energy) reaction to drive the synthesis of ATP Anabolism uses endergonic reactions, which consume ATP, to drive the biosynthesis of cellular material Reducing power, in the form of a reduced electron donor, is required to carry out both catabolic and anabolic reactions Energy conservation Electron flow 6 Principles of energy conservation - bioenergetics Free energy (G) – the energy released during a chemical reaction that is available to do work, measured in kilojoules (kJ) ΔG0’ – change in free energy during a reaction at standard conditions (pH 7, 25oC, 1 atm, 1M reactant and products) Exergonic: reactions with –ΔG0’ , release of free energy Endergonic: reactions with +ΔG0’ , require energy 7 Free energy Catabolic pathways: exergonic processes that generate free energy Free energy produced is conserved by synthesizing energy-rich molecules, e.g., ATP ATP requires ΔG0’ = 31.8 kJ/mole Aerobic respiration of 1 mole of glucose could produce 91 moles of ATP under standard conditions, though 38 moles actually produced; some lost as heat Anabolic pathways: endergonic processes in cellular synthesis require energy Energy comes from ATP Catabolic and anabolic reactions are fundamentally linked 8 Reduction-oxidation (redox) reactions Transfer of electrons is required for both catabolic and anabolic reactions Electron transfer reactions are called redox reactions as it comprises two half reactions Electrons are transferred from an electron donor in one half reaction to an electron acceptor in the other half reaction The loss of electrons is called oxidation, hence the electron donor (commonly called energy source) is oxidized in the redox reaction The gain of electron is called reduction, hence the electron acceptor is reduced In this example of redox reaction, the two half reactions are: Oxidation of glucose (electron donor) to CO2 Reduction of O2 (electron acceptor) to H2O 9 Reduction potential Catabolism depends on the directed flow of electron, from electron donor to electron acceptor Reduction potential (E0’): affinity of substance for electrons Redox Reactions and Reduction Potentials Electrons cannot exist in solution Must be transferred form one atom (or molecule) to another directly in redox reactions Redox reactions occur in pairs, therefore are called half reactions or redox couple By convention, in expressing the redox reaction, the reactant that is oxidized is written on the left, and the reactant that is reduced is written on the right with a forward slash in between (e.g., CO2/glucose and ½ O2/H2O) 10 The redox tower – examples of redox couples in nature In this redox tower, redox couples are arranged from the strongest electron donors at the top to the strongest electron acceptors at the bottom. Reduction potential (E0’): tendency to donate or accept electrons Expressed in volts (V) compared with reference (typically H2 Electrons are negatively charged, so the reduced substance of a redox couple has a tendency to donate when the redox couple has a negative reduction potential; e.g., glucose in CO2/glucose, E0’ = –0.43 V Oxidized substance will accept electrons when the redox couple has positive reduction potential; e.g., O2 in ½ O2/H2O The greater the difference in reduction potentials of 11 the two half reactions, the more energy is released Electron carriers; e.g., NAD+/NADH In cells, the transfer of electrons from one substance to another rarely takes place in a single step Movement in electrons proceeds through consecutive reactions at different locations in the cell Soluble electron carriers such as nicotinamide adenine dinucleotide (NAD+/NADH) needed to carry electrons NAD+/NADH redox couple = –0.32 V Reduction requires 2 e– and 1 H+ Reduction of NAD+ results in NADH + H+ 12 NAD+/NADH cycling NAD+/NADH are coenzymes They are diffusible and mediate many different reactions They and many other molecules are electron shuttles Typically involved in catabolic reactions NADP+/NADPH is another electron shuttle typically involved in anabolic reactions 13 Calculating changes in free energy Calculating free energy based on the redox tower ΔE0’ is proportional to ΔG0’ ΔG0’= nFΔE0’ where n is the number of electrons transferred and F is Faraday constant (96.5 kJ/V) ΔG0’ can also be determined if the free energy of formation (Gf0) is known See table 3.3 for free energy of formation of select compounds ΔG0’ tells whether the reaction is exergonic (–ΔG0’) or endergonic (+ΔG0’) 14 Catalysis Free-energy calculations only reveal energy change during a reaction, nothing about the rate Consider the formation of water from hydrogen and oxygen Favorable reaction with ΔG0’ of –237kJ Reaction will not occur spontaneously Breaking of the bonds of the two reactants requires energy Energy of activation – minimum energy required to initiate a chemical reaction Catalyst is required to overcome the energy of activation Can increase the reaction rate by 108-1020 times the spontaneous rate 15 Catalysis and enzymes Enzymes are biocatalysts Typically proteins, some RNAs Lowers activation energy, increases enzyme rate In reaction, enzyme binds substrate, forming enzyme-substrate complex, then releases product and enzyme 16 2. Catabolism: chemoorganotrophs 1. 2. 3. 4. 5. Energy-rich compounds Glycolysis, the Citric Acid Cycle, and the Glyoxylate Cycle Principles of Fermentation Principles of Respiration: Electron Carriers Principles of Respiration: Generating a Proton Motive Force 17 Energy-rich compounds of cells Energy is released from redox reactions, but how is energy conserved to do work Adenosine triphosphate (ATP) the most important energy-rich phosphorylated compound Several others have energy-rich phosphate or sulfur (coenzyme A) bonds Cells typically use compounds with free energy of hydrolysis (∆G0’) <-30 kJ/mol as energy “currency” 18 Glycolysis pathway Nearly universal pathway for glucose catabolism Glucose oxidized to pyruvate: 1) preparative stage, 2) oxidative stage, 3) reductive stage Can participate in multiple forms of catabolism (fermentation, aerobic respiration, anaerobic respiration) 19 Citric acid cycle Pathway by which pyruvate is oxidized to CO2; for every pyruvate that enters the cycle the following are produced 1 ATP 3 CO2 4 NADH 1 FADH2 20 Other catabolic pathways of chemoorganotrophs Glycolysis and citric acid cycle can oxidize several C4-C6 compounds; e.g., glucose, citrate, malate, fumarate, succinate Some C2 (e.g., acetate) compounds are catabolized through glyoxylate cycle C3 compounds are carboxylated by pyruvate carboxylase or phosphoenolpyruvate carboxylase 21 Essentials of fermentation Fermentation of glucose involves substrate-level phosphorylation and redox balance via pyruvate reduction + excretion as waste Fermentation is a form of anaerobic catabolism Many fermentation products (waste) are useful for humans (e.g., beer and wine, yogurt, cheese, effect of microbiome on health) 22 Maintaining redox balance NAD+ Balanced Redox NADH NADH Intermediate Substrate ADP ATP NAD+ Fermentation product Cell growth All fermentations must do two things: Conserve energy – produce energy-rich compounds (e.g., ATP) Redox balance – donate electrons back to an electron acceptor derived from original organic donor Oxidize NADH back to NAD+ Maintain balance by dumping electrons onto some intermediates (e.g., pyruvate), generating fermentation end products (e.g., ethanol, lactic acid) 23 Respiration Respiration: electrons transferred from reduced electron donors (e.g., glucose) to external electron acceptors (e.g., O2) Reduced electron shuttles produced by glycolysis and citric acid cycle must be reoxidized for redox balance Reoxidation in respiration is achieved by: Electron transport using electron carriers Electron transport occurs in cytoplasmic membrane (mitochondrial membrane in eukaryotes) Forms electrochemical gradient (usually protons) that conserves energy through ATP synthesis 24 Respiration and the proton motive force: overview The coenzyme NADH (generated from the citric acid cycle, glycolysis etc) possesses electrons with high reduction potential Oxidation of NADH would release a large amount of energy Electrons from NADH are transferred through a series of redox reactions localized at the cytoplasmic membrane. As electrons flow from a more negative to a more positive reduction potential, protons (H+) are transported from the inside to the outside of the cellular membrane Oxidative phosphorylation: synthesis of ATP at the expense of proton motive force 25 Electron carriers in electron transport Electron carriers are arranged in membrane with increasingly more positive reduction potential NADH dehydrogenases – active site binds NADH Two e– and one H+ from NADH plus on H+ from cytoplasm are transferred to a flavoprotein The regenerated NAD+ returns to participate in glycolysis Flavoproteins contain flavin (Flavin mononucleotide (FMN) or Flavin adenine dinucleotide (FAD)) as a prosthetic group Accepts 2 e– and 2 H+ from NADH dehydrogenase and donate one e– with the proton released into the cytoplasm 26 Cytochromes and other electron carriers Cytochromes contain heme prosthetic groups Many types depending on the type of heme group Nonheme iron-sulfur proteins Quinones – small hydrophobic redox molecules that lack a protein component Nonheme iron-sulfur protein Cytochrome Ubiquinone 27 Generation of proton motive force during aerobic respiration in Paracoccus denitrificans Electrons are flowing from a more negative to a more positive reduction potential Note: this is one wellstudied example, there are many electron transport schemes 28 Complex I: NADH dehydrogenase Primary electron donor can enter the chain at either Complex I or Complex II Complex I contains 14 different proteins functioning as a unit Also called NADH dehydrogenase or NADH:quinone oxidoreductase NADH oxidized to NAD+, ubiquinone (Q) reduced to ubiquinol (QH2) and diffuses to Complex III Four H+ released outside – contribute to proton motive force 29 Complex II: succinate dehydrogenase Complex II – also called succinate dehydrogenase complex Alternate entry point for primary electron donor 2 e– from FADH2 and 2 H+ from cytoplasm transferred to ubiquinone (Q) to ubiquinol (QH2) Less energy conserved due to lack of H+ translocation 30 Complexes III and IV: cytochromes Complex III: cytochrome bc1 complex Transfer e– from QH2 to cytochrome c Pumps 2 H+ from QH2 to outside cytoplasmic membrane Cytochrome c shuttles to Complex IV Complex IV: cytochrome a and a3 Terminal oxidase, reduces O2 to H2O Needs 4 e– and 4 H+ from cytoplasm Pumps 1 H+ per electron to outside 31 Electron transport and proton release For every 2 e– from NADH to O2, 10 H+ are transferred outside cytoplasmic membrane 4 at Complex I 4 at Complex III 2 at Complex IV 2 consumed in cytoplasm (H2O) For every 2 e– from FADH2 to O2, 6 H+ are transferred outside membrane 4 at Complex III 2 at Complex IV 2 consumed in cytoplasm (H2O) 32 Generation of ATP from proton motive force ATP synthase (ATPase) Uses energy from proton motive force to form ATP Comprises two components F1: multiprotein complex extending to the cytoplasm that catalyzes ATP synthesis F0: membrane-integrated proton-translocating multiprotein complex H+ passing through F0 is coupled to the rotation of F1 The free energy captured in the rotation is coupled to ATP synthesis ~3.3 H+ required to produce one ATP F1/F0 complex catalyzes the reversible reaction between ATP and ADP 33 Energetics of fermentation and aerobic respiration Respiration conserves more energy than fermentation due to oxidative phosphorylation Lactic acid buildup in muscle cells after hard workout is the result of anaerobic catabolism Lactic acid fermentation in muscle cells ET: electron transport SLP: substrate-level phosphorylation Ox. Phos.: oxidative phosphorylation 34 Metabolic diversity and its relationship to oxygen Fermentation uses internal electron acceptor Respiration require external electron acceptor and generate ATP by oxidative phosphorylation Aerobic respiration uses oxygen as electron acceptor Anaerobic respiration uses electron acceptors other than oxygen; e.g., nitrate Chemolithotrophs use inorganic compounds as electron donors 35