ICARDA Soil Plant Analysis Manual 3rd Edition PDF
Document Details
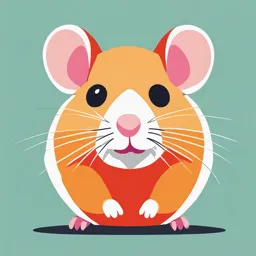
Uploaded by HarmoniousDatePalm
2013
George Estefan, Rolf Sommer, and John Ryan
Tags
Summary
This manual, the third edition of ICARDA Soil Plant Analysis Manual, details methods for soil, plant, and water analysis. Specifically designed for the West Asia and North Africa region, it covers laboratory procedures including soil sampling, processing, and physical analysis. Feedback on the publication is welcome.
Full Transcript
Methods of Soil, Plant, and Water Analysis: A manual for the West Asia and North Africa region -Third Edition- George Estefan, Rolf Sommer, and John Ryan Acknowledgments The authors would like to thank all past and present ICARDA staff associated with the Soi...
Methods of Soil, Plant, and Water Analysis: A manual for the West Asia and North Africa region -Third Edition- George Estefan, Rolf Sommer, and John Ryan Acknowledgments The authors would like to thank all past and present ICARDA staff associated with the Soil and Plant Analysis Laboratory who has contributed to its operation and the development of various procedures. Appreciation is extended to Dr. Abdallah Matar, who put the idea of analysis as a basis for crop production in the region through the medium of the region’s Soil Test Calibration Network. The contributions of Dr. Karl Harmsen, the late Dr. Sonia Garabet (formerly of the Soil Analysis Laboratory), and the late Mr. Samir Masri (formerly of the Soil Preparation Laboratory), to the authorship of the First Edition of this manual is recognized. The contribution of Dr. Abdul Rashid (Pakistan) is much appreciated, particularly for his valuable input regarding to the two First Editions of this manual. Appreciation is extended to Dr. Theib Oweis, Director of the Integrated Water and Land Management Program (IWLMP), for his encouragement and support. Dr. John Ryan has been involved in the whole endeavor of soil and plant analysis, initially in Morocco as a member of the Soil Test Calibration network and later as Soil Fertility Specialist. As much, he was led author of the first two editions of this manual, and co-author of the Third Edition. The Soil-Plant Analysis Laboratory has, since ICARDA’s inception, played a vital role in the research activities of the Natural Resource Management Program (NRMP) and indeed throughout the Center. The ICARDA management has always supported the Soil-Plant Laboratory; without this support, this manual of adapted soil and plant analysis procedures would not have been published. Our thanks go to readers who have noted errors in the first two editions of this manual and those who made suggestions for improvement. Key words:; Feedback ICARDA welcomes comment and feedback on this publication, please respond to [email protected] International Center for Agricultural Research in the Dry Areas (ICARDA) [email protected] www.icarda.org The views expressed are those of the authors, and not necessarily those of ICARDA. Where trade names are used, it does not imply endorsement of, or discrimination against, any product by the Center. Maps have been used to support research data, and are not intended to show political boundaries. Copyright © 2013 ICARDA (International Center for Agricultural Research in the Dry Areas) Box 114/5055, Beirut, Lebanon. All rights reserved. ICARDA encourages fair use, sharing and distribution of this information for non-commercial purposes, with proper attribution and citation. -1- Foreword Soil is a non-renewable resource upon which mankind depends for survival. Historically, the rise of great civilizations has been linked to the quality of soil and the availability of water. Equally, the demise of such civilizations is often attributed to mismanagement of soil and land in its broadest sense. Crop productivity and soil fertility are thus synonymous. In today’s overcrowded world, the challenge to feed and clothe the burgeoning populations of developing countries is a daunting task. Yields have to be increased from existing land areas; adding fertility to the soil to satisfy the demands of higher-yielding crops is essential. Soils vary greatly in their capacity to grow crops without fertilizer; even the richest soils experience declining yields without man’s intervention. In essence, soil is not always a perfect medium for growing plants; it is, however, the only one that is available. Soils vary greatly throughout the world; they have inherent weakness, primarily deficiencies in nutrients that are essential to growing crops. Even when adequately supplied in the early stages of land cultivation, the nutrient-supplying capacity invariably diminishes with time. Most soils are deficient in nitrogen (N); it is transient in nature, and plants need a lot of it. In many cases, phosphorus (P) is just as critical; soil chemical reactions reduce the effectiveness of P fertilizers. The soils of the West Asia and North Africa (WANA) region are generally well supplied with potassium (K), and usually don’t need fertilization, especially for low-yielding rainfed crops. In recent years, there is a growing realization has grown that other elements, e.g., micronutrients, are deficient in some areas of the region. As no essential element can substitute for another, it is critically important to identify where and when such deficiencies occur. That’s where the role of soil and plant analysis comes in. Techniques have been developed to evaluate soil fertility constraints based on soil chemical extraction and analysis of the plants that grow on such soils. Both are complementary and, when calibrated with field crop responses to fertilizer, provide a rational basis to identify what elements are missing, and how much fertilizer, whether organic or inorganic, to apply. Therefore, soil and plant analysis laboratories have a vital role in agricultural development of the WANA region. However, the process does not end there. To be meaningful and valid, tests have to be appropriate for the purpose intended and reliable and repeatable. The idea for this soil, plant and water analysis manual grew out of the Soil Test Calibration Program within the region’s national agricultural programs that laid the basis for sound fertilizer recommendations. Thus, it was appropriate that ICARDA should address this fundamental issue. If soil and plant tests are not reliable, the process of sampling and analysis is meaningless and undermines the validity of any agronomic trials. This manual is a cornerstone in ICARDA’s soil and water-related research program as well as its training program and is a vital link with agricultural scientists of the ICARDA’s mandate region. Dr. Mahmoud Solh Director General of ICARDA -2- Preface This laboratory manual of analytical methods has been compiled to be used primarily by research assistants, technicians and student trainees working in the laboratory. The methods have been selected from different sources and adapted for routine analyses in the WANA region. A detailed description of the methods can be found in the original publications listed in the references. As analytical techniques are developed and improving, this manual will be subject to continuous revision. Any helpful suggestion and feedback from users is most appreciated. The idea of having a common laboratory manual for the WANA region was based on the fact that the soils in this arid to semi-arid area have a common suite of properties, leading to similar nutritional problems in crops. Collaborative research in the Soil Test Calibration Network in the Mediterranean zone had also revealed that a number of soil tests for assessing nutrient availability have regional applicability (Ryan and Matar, 1990, 1992). In addition, there was hardly any laboratory manual on plant analysis relevant to the crop nutritional problems of the region. A common soil, plant, and water analysis manual is also fundamental for success of the WANA soil fertility network. To fill the gap that existed, a comprehensive manual on all essential soil, plant, and water analyses was developed. This manual was designed intentionally in a “cookbook” format, for the ease of laboratory technicians. On completion, the manual draft was reviewed and endorsed at the Soil Fertility Meeting in at Tel Hadya in 1995 (Ryan, 1997). Subsequently, the First Edition of the manual (Ryan. J, S. Garabet, K. Harmsen, and A. Rashid, “A soil and plant analysis manual adapted for the WANA region”) was published in 1996 by ICARDA, and distributed widely among soil and plant analysis laboratories throughout the region. The response was very encouraging. Most laboratory managers appreciated the initiative, particularly for compiling of all the needed methodologies in a single volume in an easy-to-use format for laboratory technicians. The Second Edition of the manual (Ryan. J, G. Estefan, and A. Rashid, 2001, “Soil and plant analysis laboratory manual”) had incorporated all the feedback from laboratory managers of the WANA region, making the manual more comprehensive, up-to-date, and with minimum technical or typographical errors. We appreciate the constructive criticism and suggestions advanced by the fellow soil scientists for improvement of the manual. The Second Edition was translated and published in Arabic, Russian, and Farsi. The Third Edition of the manual reflects the changes that have occurred within the past few years in the region, particularly will respect to water and irrigation. While it is still focused on production agriculture, it reflects an increasing concern about environmental pollution, mainly from nitrates, heavy metals, and toxic organic compounds. With greater sophistication in methodologies and equipment, most soil laboratories can now deal with both agricultural and environmental concerns. In addition, improvements have occurred in electronic handling of data and establishing databases. As with the Second Edition, we anticipate translating the Third Edition into Arabic for the WANA region in general as well as into French, particularly for North Africa. We again encourage all the recipients to continue to provide feedback regarding its utility for their particular situations, and indicate errors, if any. George Estefan, Rolf Sommer, and John Ryan -3- Contents 1. Introduction........................................................................................................................................ 7 2. Laboratory Facilities, Quality Control and Data Handling................................................................ 10 2.1. Laboratory Organization....................................................................................................... 10 2.2. Laboratory Safety................................................................................................................... 12 2.3. Quality Control and Standardization Procedures.................................................................. 16 2.4. Data Processing...................................................................................................................... 19 3. Soil Sampling and Processing........................................................................................................... 20 3.1. Soil Sampling.......................................................................................................................... 20 3.2. Preparation Laboratory Processing....................................................................................... 24 4. Soil Physical Analysis........................................................................................................................ 26 4.1. Soil Moisture Content............................................................................................................ 26 4.2. Water Holding Capacity......................................................................................................... 28 4.3. Particle Size Distribution........................................................................................................ 30 4.4. Hydrometer Method.............................................................................................................. 30 4.5. Soil Structure......................................................................................................................... 40 4.6. Soil Bulk Density..................................................................................................................... 45 4.7. Particle Density...................................................................................................................... 50 4.8. Total Pore Space and Porosity............................................................................................... 53 4.9. Soil Water Retention Curve (pF-curve).................................................................................. 54 5. Soil Chemical Analysis....................................................................................................................... 61 5.1. Saturated Paste...................................................................................................................... 62 5.2. pH........................................................................................................................................... 65 5.3. Electrical Conductivity........................................................................................................... 67 5.4. Calcium Carbonate................................................................................................................. 68 5.5. Organic Matter...................................................................................................................... 74 5.6. Particulates Organic Matter................................................................................................... 77 5.7. Cation Exchange Capacity...................................................................................................... 78 5.8. Gypsum.................................................................................................................................. 80 5.9. Nitrogen................................................................................................................................. 83 5.10. Phosphorus........................................................................................................................ 100 5.11. Potassium........................................................................................................................... 108 5.12. Sodium............................................................................................................................... 111 5.13. Calcium and Magnesium.................................................................................................... 113 5.14. Carbonate and Bicarbonate............................................................................................... 116 5.15. Chloride.............................................................................................................................. 118 5.16. Sulfate................................................................................................................................ 120 5.17. Boron.................................................................................................................................. 124 5.18. Micronutrient Cations........................................................................................................ 127 5.19. Heavy Metals...................................................................................................................... 133 -4- 5.20. Soluble Silicon.................................................................................................................... 135 6. Plant Sampling and Processing....................................................................................................... 137 6.1. Field Processing................................................................................................................... 137 6.2. Laboratory Processing......................................................................................................... 139 7. Plant Analysis.................................................................................................................................. 142 7.1. Moisture Factor................................................................................................................... 143 7.2. Nitrogen............................................................................................................................... 143 7.3. Phosphorus.......................................................................................................................... 150 7.4. Macronutrients and Micronutrients.................................................................................... 152 7.5. Heavy Metals....................................................................................................................... 157 7.6. Silicon................................................................................................................................... 160 8. Water Sampling and Processing..................................................................................................... 164 8.1. Preparation for Sampling..................................................................................................... 164 8.2. Water Sampling Processing................................................................................................. 166 9. Water Analysis................................................................................................................................ 168 9.1. pH......................................................................................................................................... 168 9.2. Electrical Conductivity......................................................................................................... 170 9.3. Total Dissolved Solids.......................................................................................................... 172 9.4. Total Suspended Solids........................................................................................................ 174 9.5. Nitrogen............................................................................................................................... 176 9.6. Phosphorus.......................................................................................................................... 182 9.7. Potassium............................................................................................................................. 187 9.8. Sodium................................................................................................................................. 189 9.9. Calcium and Magnesium...................................................................................................... 191 9.10.Carbonate and Bicarbonate................................................................................................ 194 9.11.Chloride............................................................................................................................... 196 9.12.Sulfate................................................................................................................................. 198 9.13.Boron................................................................................................................................... 199 Box 1. Laboratory Equipments.................................................................................................... 201 Box 2. Influence of Soil pH on Plant Nutrient Availability........................................................... 202 Box 3. Influence of pH on Soil and Common Acid and Alkalis.................................................... 203 Box 4. pH Meter Calibration........................................................................................................ 204 Box 5. Electrical Conductivity Meter Calibration........................................................................ 205 Box 6. Conductivity Reading and Soluble Salts........................................................................... 206 Box 7. Kjeldahl Method for Determining Nitrogen..................................................................... 207 Box 8. Irrigation Water Quality................................................................................................... 208 Box 9. The “Feel” Method for Determining Soil Texture Class................................................... 209 Box 10. Salt and Sodium Hazard................................................................................................. 210 Box 11. Bouyoucos Hydrometer Method................................................................................... 211 10. References and Supplementary Reading..................................................................................... 212 Appendix 1. Abbreviations.......................................................................................................... 218 -5- Appendix 2. Conversion Factors for SI and non-SI Units............................................................. 220 Appendix 3. Symbols, Atomic Number, and Atomic Weight of Elements.................................. 223 Appendix 4. Solution Concentrations......................................................................................... 225 Appendix 5. Some Useful Relationships...................................................................................... 226 Appendix 6. Concentration Normality, Amount of Concentrated Acids, and Bases to Make of 1 N Solution (1-L)........................................................................................................................ 227 Appendix 7. Soil pH Levels and Associated Conditions............................................................... 228 Appendix 8. Summarized Soil Test Methods for Fertility Evaluation.......................................... 229 Appendix 9. Generalized Guidelines for Interpretation of Soil Analysis Data............................ 230 Appendix 10.Suggested Plant Tissue Sampling Procedures for Selected Dryland Crops1.......................................................................................................................................................... 231 Appendix 11. Generalized Interpretation of Nutrient Concentrations in Cereal Plant Tissues Sampled at Boot Stage (Feekes Stage 10.1)........................................................................ 232 Appendix 12. Classification Criteria for Salt-Affected Soils......................................................... 233 Appendix 13. Soil Salinity Classification...................................................................................... 234 Appendix 14. Relative Salt-Tolerance Limits of Crops................................................................ 235 Appendix 15. Relative Tolerance of Plant Species to Boron Toxicity.......................................... 237 Appendix 16. Mesh Sizes of Standard Wire Sieves..................................................................... 239 Appendix 17. Equivalent Weights............................................................................................... 240 Appendix 18. Preservation Methods and Holding Times for Water Samples............................. 241 Appendix 19. Relationships between EC (saturation extract basis), and leaching fraction under conventional irrigation management.................................................................................. 242 About the authors............................................................................................................................... 243 -6- 1. Introduction The idea that one could test or analyze a soil and obtain some information about properties especially its acidity or alkalinity and its nutrient status is long established, and can be traced back to the beginning of scientific inquiry about the nature of soil. Analyses of plants to reflect the fertility status of the soil in which they grew is more recent, although visual crop observations are as old as the ancient Greeks, if not older. In the last few decades, spurred on by commercialization of agriculture and the demands for increased output from limited and even diminishing land resources, both soil and plant analysis procedures have been developed, and are still evolving. With the advent of chemical fertilizers, the need to know nutrient status of a soil in order to use such expensive and limited inputs more effectively became all the more crucial. However, if soil testing is to be an effective means of evaluating fertility status of soils, correct methodology is absolutely essential. A soil or a field may be assessed for its capability of providing a crop with essential nutrients in several ways: 1. Field plot fertilizer trials , 2. Greenhouse pot experiments 3. Crop deficiency symptoms 4. Plant analysis 5. Rapid tissue or sap analysis 6. Biological tests 7. Soil testing prior to cropping While all these approaches can be used in research, the latter one is most amenable, and one upon which recommendations for farmers can be based. On the other hand, plant analysis is a post-mortem approach and one that should be interpreted in the light of soil test results. Soil testing is now an intrinsic part of modern farming in the West, as well as in many developing countries. Tests primarily focus on the elements in most demand by crops which are supplied by fertilizers: nitrogen (N), phosphorus (P), and potassium (K). Depending upon the soil types, in some regions tests are also conducted for secondary nutrients: calcium (Ca), magnesium (Mg), and sulfur (S). In drier areas, micronutrients such as iron (Fe), zinc (Zn), manganese (Mn), copper (Cu), and boron (B) are often measured, since deficiencies of these elements are more frequently associated with calcareous soils. Indeed such areas may also have excessive or toxic levels of some elements, such as B, and high levels of elements such as Na and Mg, which can adversely affect soil physical properties. As nutrient behavior in soils is governed by soil properties and environmental conditions, measurement of such properties is often required. These include pH, salinity, organic matter (OM), calcium carbonate (CaCO3), and texture and aggregate stability. In drier areas, the presence of gypsum (CaSO4.2H2O) is also of concern. Soil testing involves four distinct phases: 1. Sample Collection: This should be such that it reliably reflects the average status of a field for the parameter considered. 2. Extraction or Digestion and Nutrient Determination: The reagents used and the procedures adopted should extract and reflect all or a portion of the element in the soil which is related to the availability to the plant, i.e., it should be correlated with plant growth. 3. Interpreting the Analytical Results: The units of measurement should reliably indicate if a nutrient is deficient, adequate, or in excess (in some cases toxic to plants). 4. Fertilizer Recommendation: This is based upon the soil test calibrated for field conditions, and considers other factors such as yield target, crop nutrient requirement, management of the crop, soil type, and method of fertilizer application, etc. -7- It should be emphasized, however, that a soil test, even if every reliable or accurate, is only one factor in making decisions about the need for fertilization. There are many other factors affecting crop growth and yield, such as soil type and environmental conditions, i.e., moisture and temperature. Because of varying and different forms of nutrients in soils, e.g., calcareous vs. acid soils, soil tests are equally varied, particularly for available P and micronutrients, and to a lesser extent for N (Walsh and Beaton, 1973; Page et al., 1982; Sparks et al., 1966). Being mobile in soils and subject to mineralization-immobilization, N poses particular problems to establish a reliable test. Tests for K, pH, OM, and CaCO3 are more straightforward. Since the development of the DTPA test of Lindsay and Norvell (1978) and adoption of Azomethine-H as a color developing reagent for B (Gaines and Mitchell, 1979), micronutrient tests for alkaline soils (e.g., Fe, Mn, Zn, Cu, and B) have become more valid and therefore more common. Though tests for gypsum are developed (Richards 1954; FAO, 1990), there are unique problems for cation exchange capacity (CEC) measurement in such soils (Rhoades and Polemio, 1977). Special mention has to be made of the original USDA Handbook 60, jointly authored by the Staff of the Salinity Laboratory in Riverside, California, with Richards (1954) as lead author. Despite its age, this iconic manual is the basis for most analyses of salinity parameters in relation to soils, water and plants, and the ensuing interpretative criteria. Though many methods have been modified, the influence of this manual (“Handbook 60” as it is called) still persists. The literature on soil testing is rich and varied. Some salient examples include: Monographs from the American Society of Agronomy for Physical (Klute, 1986) and Chemical Analysis (Page, 1982) which give detailed descriptions of all available soil tests and their modifications. Soil Science Society of America publications (Walsh and Beaton, 1973; Page et al., 1982; Westerman, 1990; Sparks et al., 1996) take a broader look at the philosophy, procedures, and laboratory operations for soil and plant analysis, with interpretation criteria for specific crops. These monographs are regularly updated. The ranges of soil and plant analysis publications include: 1. Soil Testing with a textbook format (Hesse, 1971). 2. University publications that range from those that deal with all soil, water and plant tests (Chapman and Pratt, 1961) to more narrowly based ones (Reisenaur, 1983). 3. Publications that deal with theoretical considerations involved with sampling, correlation, calibration, and interpretation (Brown, 1987). 4. Those that are commercially oriented and reflect “state of the art” instrumentation and computer-assisted data analysis and handling (Jones, 1991; Jones et al., 1991). 5. Finally, publications that are written in “recipe/cook-book” style with little or no discussion; only listed are the equipment and chemicals used and the general steps involved in the procedure (Quick, 1984). While most soil testing sources emanate from the West, publications such as those of the Food and Agriculture Organization of the United Nations (FAO, 1970; 1980) are more international in scope and assume a developing-country perspective. In such countries, soil testing is often less developed and, in some cases, does not exist. Similarly, research pertaining to soil testing and plant analysis is often fragmentary and of questionable relevance. This leads to a consideration of the West Asia – North Africa (WANA) region, which is served by the International Center for Agricultural Research in the Dry Areas (ICARDA). In this region, the development of the Soil Test Calibration Network at ICARDA served as a catalyst to promote soil testing and thus eventually lead to more efficient use of soil and fertilizer resources in the region. Its evolution and potential impact can be seen from scrutiny of the papers presented at the various workshops, e.g., Aleppo, 1986 (Soltanpour, 1987), Ankara, 1987 (Matar et al., 1988), Amman, 1988 (Ryan and Matar, 1990), and in Agadir, 1991 (Ryan and Matar, 1992), and finally in Aleppo, 1995 (Ryan, 1997). -8- Central to the Network, and indeed ICARDA’s operations, has been its Soil, Plant and Water Analysis Laboratory. Though its facilities have been designed and developed without some of the constraints experienced by other governmental and educational laboratories in the region, most of the procedures adapted by the laboratory are based on validated regional research. A key element in any worthwhile laboratory is a list of appropriate tests presented in such a manner that it can be readily followed routinely by those who actually do soil testing and plant analysis, i.e., the laboratory technicians. Therefore, the target audience for this manual is the cadre of technical staff throughout the region. A brief introduction to each test is given so that the technician should have an elementary understanding of the importance of the work he/she is doing. He/she should also know the range of values to be expected for soils and plants in the region, and therefore more readily identify gross errors. We have attempted to select the most appropriate methods for each test and present it in a clear, stepwise manner. While the manual primarily deals with soil testing, a number of important plant tests are presented, since they may complement the soil tests and are frequently needed for soil fertility and plant nutrition studies. Similarly, due emphasis has been given to physical properties, describing the tests routinely done along with chemical analysis. The importance of proper soil, plant and water sampling has been highlighted, and guidelines of sample collection, processing, and storage have been provided. We have also presented material on laboratory organization and safety aspects, which are often overlooked by technical staff but which impinge greatly on their work output and its reliability. Additionally, the Appendices contain useful information on related practiced aspects such as abbreviations, conversion factors, atomic weights, solution concentrations, pH effect on soil conditions, summarized soil test methodologies, plant sampling guidelines, criteria for interpreting soil and plant analysis data, soil salinity, and B toxicity interpretations. -9- 2. Laboratory Facilities, Quality Control and Data Handling 2.1. Laboratory Organization Soil, plant, and water analyses are carried out by various institutions in the public or government sector, as well as in the private domain. Laboratories are operated by several entities, including Ministries of Agriculture, National Research and Teaching Institutes, International Organizations, and Commercial Companies. To be much effective, analytical services should be closely linked to the extension/advisory services and should maintain a functional relationship with the universities and research stations. The kind of facility for such analyses depends on the type of institution it serves, the nature of the clientele, and the volume of samples to be analyzed. Nevertheless, all laboratories, regardless of the size, should be designed in a manner to facilitate operational efficiency, minimizes contamination, and produces reliable and repeatable results. Various publications deal with management considerations in the design and operation of soil testing (e.g., Walsh and Beaton, 1973). While the advantages of standardized laboratory designs are self-evident, many laboratories in the WANA region have apparent deficiencies in this respect (Ryan, 2000; Ryan et al., 1999). All too often one sees soil samples stored or, worse still, ground in wet chemistry laboratories. Similarly, many laboratories are set up in a manner that inadvertently hinders efficient use of staff resources. Soil, plant and water analysis facilities should be located in the same building and be under one unified administration. The Soil, Plant, and Water Analysis Laboratory of ICARDA was designed in the 1980 with these considerations in mind. The various components of the laboratory reflect a logical activity framework. While no two laboratories are ever the same or have the same complement of equipment, the details presented for ICARDA’s laboratory will, hopefully, serve as a general guideline for laboratory arrangement and the type of equipment needed for routine service-oriented operations. The Soil, Plant and Water Analysis Laboratory of ICARDA is represented in the following parts: 1. Soil Preparation Room Where large bulk samples, transported by truck, are received, dried and sieved. This facility is equipped with a large-capacity oven, freezers, soil grinder, containers and trays, stainless steel soil sieve sets, vacuum pumps, sample dividers, different types of soil sampling (augers, spade, and metal rings), exhaust hood, and a compressed-air machine. Soil samples (~0.5 kg) are dried and placed in clean containers and then transferee to the soil testing laboratory for requested analysis. 2. Soil Store Room Where all samples are retained for at least 2 years after analysis; bulk samples of special soil types are kept indefinitely. An inventory or catalogue of all soil samples is maintained. 3. Freezer Room In some cases it is necessary to temporarily store large numbers of samples in cold conditions, such as a freezer room, pending analysis; without such conditions, any delay in analysis would result in bacterial changes in soil samples that would invalidate the analytical results. - 10 - 4. Chemical Analysis Room The chemical analysis room is where the sub-sample of dried soil is received in the soil testing laboratory for requested analyses. This facility is normally has various equipment, such as N digestion/distillation, CN-elemental analyzer, flame photometer, spectrophotometer, pH meter, and conductivity meter. The more traditional analytical processes of weighing, stirring, shaking, filtering under suction, heating, drying, incubating and centrifuging are done almost exclusively with the aid of electrical machines and devices. In addition, for the digestion and preparation of reagents, chemicals carry out in the Fume Hood. 5. Instrument Room This is where soil extraction, where necessary, is carried out for analysis. This facility is normally equipped with various equipment, e.g., atomic absorption spectrophotometer, computer, refrigerator, etc. 6. Physical Analysis Room This is where the dried soil sub-samples are received in the soil testing laboratory for requested analyses. This facility is equipped with various equipment, such as pF instrument for field capacity and permanent wilting point, soil dispersing stirrer (a high-speed electric stirrer with a cup receptacle) for particle size distribution, aggregate stability (for wet and dry methods) instruments, balances, permeability apparatus, and water bath. 7. Water Analysis Room In this room the more traditional analytical processes are carried out, e.g., pH, electrical conductivity, and anions and cations. General Equipment Various items of equipment and associated furnishings are generally found in soil, plant and water analysis laboratories, as indicated as follows: Laboratory working tables Appropriate racks Weighing benches Cupboards Laboratory desks and chairs Fixed suction unit Fume hood or exhaust systems mounted above the flame photometer, atomic absorption spectrometer, muffle furnace, and Kjeldahl digestion Washing-sinks for cleaning glassware Drawing desk facilities for storing standard forms and documentation - 11 - 2.2. Laboratory Safety As with any place of work, safety is an important consideration in soil, plant and water analysis laboratories, and one that is frequently overlooked. A safe working in a chemical laboratory needs special care, both in terms of design and construction of the laboratory building, and handling and use of chemicals. For chemical operations, the release of gases and fumes in some specific analytical operation are controlled through a fume hood or trapped in acidic/alkaline solutions and washed through flowing water. Also, some chemical reactions during the process of analysis, if not handled well, may cause an explosion. Analytical processes normally carried out at room temperature can be affected by differences in temperature so that an analysis performed in a “cold” room can give a different result to one performed in a “hot” room. Many chemicals are affected by the temperature and humidity conditions under which they are stored, particularly if these conditions fluctuate. The air temperature of the laboratory and working rooms should ideally be maintained at a constant level (usually between 20 and 25 °C). Humidity should be kept at about 50 %. All staff, irrespective of grade, technical skill or employment status, should be briefed on all aspects of safety upon commencement of work. Periodic reminders of such regulations should be given to encourage familiarity with respect to regulations. Ideally, posters relatively to laboratory safely should be prominently displayed in the laboratory. While rules pertaining to safety can be extensive, we have endeavored to concisely list the more important ones within different categories of concerns. These have been adapted from laboratory safety guides developed by Kalra and Maynard (1991) and Okalebo et al. (1993). General Attitude 1. Develop a positive attitude towards laboratory safety 2. Observe normal laboratory safety practices 3. Maintain a safe and clean work environment 4. Avoid working alone Instrument Operation 1. Follow the safety precautions provided by the manufacturer when operating instruments. 2. Monitor instruments while they are operating. 3. Atomic Absorption Spectrophotometer must be vented to the atmosphere. Ensure that the drain trap is filled with water prior to igniting the burner. 4. Never open a centrifuge cover until machine has completely stopped. 5. Use of balances: The warming-up time of the balances is 30 minutes Spilled chemicals should be removed immediately Never blow away the spilled product Brushes are supplied with the balances - 12 - Accidents 1. Learn what to do in case of emergencies (e.g., fire, chemical spill, etc.). Fire-fighting equipment must be readily accessible in the event of fire. Periodic maintenance inspections must be conducted. 2. Learn emergency First Aid, such supplies are a necessity and laboratory staff should be well trained in their use. Replacement of expended supplies must take place in a timely fashion. 3. Seek medical attention immediately if affected by chemicals, and use First Aid until medical aid is available. 4. Access to eye-wash fountains and safety showers must not be locked. Fountains and showers should be checked periodically for proper operation. Chemicals 1. Use fume hoods when handling concentrated acids, bases or other hazardous chemicals. 2. Do not pipette by mouth; always use a suction bulb. 3. When diluting, always add acid to water, not water to acid. 4. Some metal salts are extremely toxic and may be fatal if swallowed. Wash hands thoroughly after handling such salts or indeed any chemical regardless of toxicity. Chemical spills should be cleaned promptly and all waste bins emptied regularly. 5. All reagent bottles should be clearly labeled and must include information on any particular hazard. This applies particularly to poisonous, corrosive, and inflammable substances. 6. For the preparation of reagents, only distilled water (DI) is used. Note that volatile acids, ammonia, nitrite, chlorine and carbon dioxide have to be removed by means of a column containing resin (deionizer) which will exchange the charged ions, is needed. Furnaces, Ovens, Hot Plates Use forceps, tongs, or heat-resistant gloves to remove containers from hot plates, ovens or muffle furnaces. Handling Gas Cylinders of compressed gases should be secured at all times. A central gas facility is preferred. Maintenance 1. All electrical, plumbing, and instrument maintenance work should be done by qualified personnel. 2. Fume hoods should be checked routinely. 3. As most equipment operates on low wattage, an Un-interruptible Power Supply (UPS) provides stable power and allows the completion of any batch measurement in the event of power outage. - 13 - Maintenance of Pipettes 1. At the end of the working day, wash the pipette with tap water and then several times with distilled water. 2. Dry the pipette in an oven. 3. Keep the pipette upside down in a special clamp. Eating and Drinking 1. Do not eat or drink in the laboratory. This is essential both for reasons of health, to eliminate any possibly of poisoning, and to reduce contamination. Specific areas should be designated for staff breaks. 2. Do not use laboratory glassware for eating or drinking. 3. Do not store food in the laboratory. Protective Equipment Body Protection Use laboratory coat and chemical-resistant apron. Hand Protection Use gloves, particularly when handling concentrated acids, bases, and other hazardous chemicals. Dust Mask A mask is needed when grinding soil, plant samples, etc. Eye Protection Use safety glasses with side shields. Contact lenses should never be worn around corrosives. Make sure that your colleagues know if you wear contact lenses. Full Face Shield Wear face shields over safety glasses in experiments involving corrosive chemicals. Foot Protection Proper footwear should be used; sandals should not be worn in the laboratory. Waste Disposal 1. Liquid wastes should be poured carefully down a sink with sufficient water to dilute and flush it away. Keep in mind that local ordinances often prohibit the disposal of specific substances through the public sewerage system. 2. Dispose-off chipped or broken glassware in specially marked containers. Continuing Education 1. Display in a prominent place posters on “Laboratory Safety” which pictorially describe various phases of laboratory activities. 2. Similarly, posters depicting First Aid after laboratories accidents should be prominently displayed. Such posters are not for ornamentation; they are for the protection of laboratory personnel, who should be thoroughly conversant with all procedures and eventualities. 3. If the laboratory is a part of a large institution, the laboratory staff should know the Safety Officer or person responsible for safety. If it is a small operation, one laboratory staff member should be responsible for safety. - 14 - Contamination Contamination is a most serious problem in any laboratory; therefore, its sources must be identified and eliminated. Some common sources of contamination are: - External dusts blown from the surrounding environment - Internal dust resulting from cleaning operations - Cross-contamination derived from while handling many samples at the same time (e.g., handling plant and soil samples together) - Failure to store volatile reagents well away from the samples - Washing materials, particularly soap powder - Smoking in the laboratory Technical Remarks 1. The air-dry moisture in a soil sample taken straight from a hot and humid storeroom (or a very cold one) may be different from that in a similar sample kept in an air-conditioned laboratory; both may be weighed for analysis at the same time as “air-dry’ samples. 2. The tap water supplied to a laboratory should be entirely free of pollution, as free as possible from insoluble matter, and under good and steady hydrostatic pressure. It may be necessary to filter the supply to certain pieces of equipment. 3. Drainage should be to a main drain if possible or to good-sized “soak-away”. Effluents from soil laboratories contain considerable quantities of waste soil in addition to acid and alkaline liquids. The facilities should be provided in the design of the drainage system for periodic cleaning and removal of solid matter. - 15 - 2.3. Quality Control and Standardization Procedures What follows in this section is a synthesis from the manual of Okalebo et al. (1993). Quality control is an essential part of good laboratory practice. During routine analyses, errors may gradually appear due to contamination, changes in reagent quality, environmental differences, operator error, and instrument calibration or failure. Maximum reproducibility and adequate accuracy of results are the important objectives. Repeated measurement of an air-dried soil sample should provide consistent results when analyzed over time for most routine chemical procedures. The deviation of an observed value from its absolute “true” value results from either systematic or random errors. Once identified, systematic errors are more easily corrected than those which occur at random. Three precautions are essential for laboratory quality control and should be routinely included among the test samples. These precautions involve the use of blanks, repeats, and internal references, as elaborated below. Whenever a new procedure is introduced to the laboratory, its accuracy should be evaluated and compared to the test already in use. Both methods should be compared for a homogeneous test sample using ten-fold replicates, with the standard deviation calculated for each set. This provides a measure of precision. Known amounts of reagent should be added to the homogeneous test sample, the procedures repeated, and the mean and standard then deviation calculated. The agreement between the increases in the values obtained to the known increase in test sample concentration provides a test of accuracy. For procedures in which the test material is known to interact with the added reagent, as with phosphorus-sorptive soils, this test can be conducted by reagent solutions. Blanks Blanks are reaction vessels that are subjected to identical procedures as the sample in a given batch which has no added test material. Blanks allow correction for any background contamination introduced from reagents, filter papers or other systemic sources of error. Provided the blank values are consistent, the mean value can be subtracted from the sample value. When blanks yield large values, this suggests excess extraneous contamination; in such cases, the entire batch analysis be repeated. Repeats At least 1 in 10 samples selected from the test materials and placed at random within the batch should be analyzed in duplicate. The choice of 1 in 10 is a suggested compromise between the ideal of analyzing all samples in duplicate, considering the time, effort and expense of doing so. Obviously, the analytical results for given pairs of duplicate repeats should closely resemble one another, in general, repeat values should fall within ±2.5 – 5.0 % of their mean, depending on the analysis in question; any greater discrepancy must be investigated. If repeat values are not consistent, the entire batch should be re-analyzed. - 16 - Internal References Internal reference samples are necessary for each type of test material and analysis practiced within the laboratory. The internal sample should not be the same as the homogeneous material routinely used in the testing new methods and analytical technique. A sample obtained from a large, well- mixed and homogeneous composite bulk sample should be included in each batch analyzed. Variation from the mean as calculated over previous batches may be indicated as an error. Analytical results for the internal reference may be plotted on a quality control chart to monitor the performance of the analyses over time. Corrective action could be taken if a single value exceeds the ± 3 standard deviation limits or if two successive values exceed the ± 2 standard deviations. Periodically, the critical limits could be re-assessed by re-calculation of the overall standard deviation of the internal reference sample as more data are accumulated. Standardization of Methods Results can only be validly compared to one another when they have been obtained using standardized methods. Collaboration between laboratories can be improved by exchanging reference materials and then comparing their results (Ryan and Garabet, 1994). Such materials are referred as “External References”. An example of such standardization is the exchange network of ISRIC (International Soil Reference and Information Center) in Wageningen, The Netherlands, and operating international soil and plant analytical exchange programs. Most external reference samples are costly, and their frequent use increases operating costs of the laboratory. Internal reference samples are usually much less expensive. Thus, if a relationship between external and internal reference samples can be firmly established, frequent use of internal reference sample, with occasional use of the external reference sample, can reduce costs, while still providing acceptable quality assurance. - 17 - Errors in Quantitative Analysis In dealing with analysis, the concepts of accuracy and precision (See Figure.1) are important. Accuracy: A measure of systematic error or the degree of agreement of an experimental value with the true or expected value of the quantity of concern. Accuracy of the value is important, but it is important to know when to use a given analytical method and to know its limitations. Precision: A measure of reproducibility affected by random error. It is usually described by the standard deviation, standard error, or confidence interval. Figure. 1 Illustration of Accuracy and Precision - 18 - 2.4. Data Processing A considerable amount of information is generated in any soil, plant, and water analysis laboratory. In order to economically justify the existence of a laboratory, it is necessary to have a record of the number of samples analyzed and the types of analyses performed. With the advent of the computer, such storage is easy and retrieval is greatly facilitated. Computer processing offers the advantage of: - Easier manipulation of large data sets, - Reduced errors in calculation of recommendations, - Preparation of reports, - Automated invoicing and addressing, and - Ready access to historical data for preparation of soil test summaries. The degree to which laboratories should be computerized depends on sample volume, location and user services offered. In general, laboratories with a large volume of samples, and which offer a range of analyses, have more need for computer sophistication and automation than laboratories with a small sample turn-over. In order to facilitate data processing, standard information sheets are required. These vary from laboratory to laboratory, but usually include details of analyses required for the sample and information on the crop to be grown, the soil type, and previous cropping history, particularly with respect to fertilization. Such information enables one to answer questions on the extent of nutrient deficiency in any area from which the samples were obtained, and how fertility levels change over the years. Computer programs are increasingly used to interpret soil test data and making fertilizer recommendations. Several such programs do exist. Standardized report forms for making fertilizer recommendations combine inputs of soil test data together with other soil and crop information. In order to do this, the tests used (soil NO3-N, available P, etc.) must be calibrated with field crop response. With a relatively large output of analyses associated with a large number of on-station and on- farm research trials, analytical results from ICARDA’s laboratory are stored in a computer program to generate fertilizer recommendations based on soil test values. Where soil maps and rainfall data are available, the accumulated soil test values of known locations can help establish relationships with soil type, region, and climatic zone. - 19 - 3. Soil Sampling and Processing While the main focus of this manual is to present an easy-to-use methodology for soil testing and, to a lesser extent, for plant analysis, their related aspects are worthy of due emphasis. Therefore, a brief description of such aspects follows. While much attention is given to laboratory procedures, the process of obtaining soil for analysis, i.e., soil sampling, is often ignored or poorly considered. A good sampling plan should provide a measure of the average fertility level of a field and a measure of how variable it is. If a sample is not representative of the field or is incorrectly taken, the resulting analytical data are meaningless, or at best, difficult to interpret. The error in field sampling is generally much greater than that due to chemical analysis. Therefore, obtaining a representative soil sample from a field is the most important step for making a meaningful soil analysis. 3.1. Soil Sampling A soil sample should be composed of several sub-samples representing a seemingly uniform area or field with similar cropping and management history. There is no universally accepted numbers of sub-samples for different field situations. However, the following points can serve as guidelines: Composite Sampling At ICARDA, eight sub-samples are taken per hectare (ha) in a diagonal pattern for obtaining one composite sample. Other plans range from 5 to 25 borings or sub-samples per composite sample, with sample units varying from 2 to 8 ha. Fewer sub-samples are needed where little or no fertilizer has been used. Sampling areas are often traversed in a zigzag pattern to provide a uniform distribution of sampling sites. Some of these methods are represented in Figure 2 and 3. Correspondingly, more sub-samples are needed where fertility is variable due to hand broadcasting of fertilizers and/or with cropping-livestock systems. Indeed, banding of fertilizer poses serious problems for reliable sampling. Thus, the number of sub-samples taken by farmers should be realistic, considering the particular field situation. - 20 - Figure 2. Some suggested methods for soil sampling; each dot represents a sample point, with formation of a sample pattern within the fielded Figure 3. Sampling pattern for fertility test in a non-uniform land (sample numbers refer to composite sample; Tarzi, 1984) - 21 - Sampling Time Soil samples can be taken any time that soil conditions permit, but sampling directly after fertilization or amendment application should be avoided. Samples taken during the crop growth period will help in knowing the nutrient status of the soil in which plants are actively taking up nutrients. In the WANA region, it is recommended that sampling be carried out in autumn (before planting) if fertilization is intended at planting. It is important to sample at similar times year after year for comparing analysis at regular time intervals. Sampling Depth For most purposes, soil sampling is done to a depth of about 20-cm. Available P, NO3-N, and micronutrients in such samples are related to crop growth, and nutrient uptake. In some cases, especially in irrigated areas, sampling to a depth of 60-100 cm is desirable, especially for monitoring nitrate (NO3-N) leaching. Depth-wise soil samples should also be taken where there is a concern about B toxicity. Soil profile Sampling Tools A uniform slice should be taken from the surface to the depth of insertion of the tool; the same volume of soil should be obtained in each sub-sample. Augers generally meet these requirements. In areas where the topsoil is dry, e.g., during summer, topsoil sampling can be done by a metal ring, by digging out the soil inside the ring, because it is almost impossible to sample dry topsoil with an auger. Soil samples for micronutrient analysis should be taken using a stainless steel auger, or at least ungalvanized auger (because galvanized coating is zinc oxide). Soil sampling tool: Auger Researchers generally use augers for field sampling. Farmers or Extension Agents can use shovels or trowels, with almost the same result. If you do not have sampling tools, use a spade as follows: - Dig a V-shaped hole 15 to 20 cm deep. Then take a fine thick slice from the smooth side (see Figure 4). - Trim the sides leaving a fine strip then dump this strip into a clean bucket. Break the clods, and mix thoroughly. Remove large rocks, pieces of sod, earthworms, etc. Put the soil into the sample container and label the box clearly. For a moist soil, the tube auger or spade is considered satisfactory. For harder soil, a screw auger may be more convenient. - 22 - Figure 4. Soil sampling tool: Spade Instructions for Field Processing Disturbed soil samples should be put in plastic bags (tags and markers are required), or aluminum or stainless steel boxes Depending on the subsequent analysis samples may be kept cool until laboratory analysis. Bags should be examined for cleanliness as well as for strength. Soil samples can be transported to the laboratory in cardboard boxes or sacks. All information about samples is recorded, and each sample is given a laboratory number. Sketch your field. Diagram it the way you sampled it. Be sure sampled areas are labeled the same as sample containers. (This is so you have a record of which recommendations apply to which areas – do not rely on your memory). Information sheet should be clearly written with copying pencil. Fill out the information sheets. The more information you can provide with each sample, the better your recommendation will be. Do not sample unusual area, like unevenly fertilized, old channel, old bunds, area near the tree, and site of previous compost piles and other unrepresentative sites. Avoid any type of contamination at all stages. Soil samples should never be kept in the store along with fertilizer materials and detergents. Contamination is likely when the soil samples are spread out to dry in the vicinity of stored fertilizers or on floor where fertilizers were stored previously. Collect samples from the middle of the rows, when crops have been planted in rows, so as to avoid the area where fertilizer has been band-placed. - 23 - 3.2. Preparation Laboratory Processing Handling in the laboratory As soon as the samples are received at the soil preparation facility, they should be checked with the accompanying information list (including sample number, depth, and date of sampling should be written on the bag from outside, and on a sample card placed inside the bag). Information regarding samples should be entered in a register and each sample be given a laboratory number. The soil-fresh sample received in the laboratory should be analyzed directly after sampling for determination of nitrate, nitrite and ammonium. These samples should not be dried, and the results are expressed on oven-dry basis by separately estimating moisture content in the samples. If short-term storage is unavoidable, this must be done in a fridge at temperature close to 0 °C (but not below zero!). Lag time between field sampling and analysis must be minimized. Otherwise, storage time will inevitably introduce an additional factor influencing analysis results. Drying of the soil samples The soil-fresh samples received in the laboratory should be dried in wooden or enameled trays. The trays can be numbered or a plastic tag could be attached, and care should be taken to maintain the identity of each sample at all stages of preparation. During drying, the soils are allowed to dry in the air. Alternatively, the trays may be placed in racks in a hot air cabinet (the temperature should not exceed 35 °C and humidity should be between 30 and 60 %). In general, excessive oven-drying of the soil affects the availability of most of the Ovens nutrients present in the sample, and should be avoided. Only air-drying is recommended for some of the nutrients present in the sample. Such as, drying has negligible effect on total N content but the NH4 and NO3 content in the soil changes with time and temperature. The microbial biomass is also significantly affected by drying at high temperature. Preparation of soil samples After drying, the samples are ground with a wooden pestle and mortar in preparation room (which is separate from the main laboratory), and clods and large aggregates are crushed and mixed. Remember that: Pebbles, concretions, and stones should not be broken during grinding. Care should be taken not to break the individual soil particles during the grinding process. The entire sample should be passed through the sieve, except for concretions and pebbles of more than 2-mm. The purpose of grinding is to reduce heterogeneity and to provide maximum surface area for physical and chemical reactions. Various devices are used for crushing and grinding soils. However, choice of equipment depends on: - Amount of sample to be crushed or ground - Contamination that can be tolerated - Degree of fineness to be attained - The analysis in question - 24 - After grinding, the soil is screened through a 2-mm sieve. The coarse portion on the sieve should be returned to the mortar for further grinding (except for concretions, pebbles, and organic residues). Repeat sieving and grinding till all aggregate particles are fine enough to pass the 2-mm sieve. It is necessary to reduce the size of the large sample for ease of storage and handling. To achieve this goal, a random method of sub-sampling is essential. Sample splitting can be performed with a mechanical sample splitter, such as a Riffle-type Sample Splitter, by which the sample is divided in half by a series of chutes. This process can be repeated as many times as necessary. Another way for reduction of sample size is by quartering. The sample is spread uniformly over a plastic or paper sheet and divided into four equal portions. For example, portions 2 and 3 are collected and thoroughly mixed, whereas the remainder is discarded. Following the drying and preparing processes, half of the amounts of the dried soil sub- sample are placed in a clean container and then transferee into the soil testing laboratory for the requested analysis, the rest should be stored in cardboard boxes in a store room. Remember, if the soil is to be analyzed for trace elements, containers made of copper (Cu), zinc (Zn) and brass must be avoided during grinding and handling. Sieves of different sizes can be obtained in stainless steel. Aluminum or plastic sieves are useful alternative for general purposes. Soil grinder Riffle-type soil samples splitter (sample dividers) Reduction of soil sample size - 25 - 4. Soil Physical Analysis Soil physical measurements are numerous, depending on the objective of the study for agricultural purposes. These measurements generally includes soil water purpose on the content, infiltration and hydraulic conductivity, evapotranspiration, heat, temperature, reflectivity, porosity, particle size, bulk density, aggregate stability, and particle size distribution. Soil moisture is routinely measured on field-moist samples, since all physical analyses are expressed on oven-dry basis (16-18 hours drying at 105 oC). As texture (e.g., whether sandy or clay) is quite important in relation to nutrient behavior, particle size distribution is often carried out, especially if more precision is needed than provided by the qualitative physical “feel” approach for determining texture. 4.1. Soil Moisture Content As water is the most limiting factor in the arid to semi-arid areas, soil moisture determination is of major significance. Soil moisture influences crop growth not only by affecting nutrient availability, but also nutrient transformations and soil biological behavior. Therefore, at ICARDA soil moisture is routinely measured in most field trials. While it can be assessed in the field by the neutron probe, the gravimetric approach is more flexible, as samples can be readily taken from any soil situation. All analyses in the laboratory are related to an air- or oven-dry basis, and therefore must consider the actual soil moisture content (Sparks et al., 1966). Apparatus Electric oven with thermostat Desiccator Procedure 1. Weigh 10 g air-dry soil (< 2-mm) into a previously dried (105 °C) and weighed metal can with lid. 2. Dry in an oven, with the lid unfitted, at 105 °C overnight (normally for 24 hours). 3. Next day, when the soil has dried, remove the container from the oven, using tongs; fit the lid, cool in a desiccator for at least 30 minutes and re-weigh. Calculation - 26 - Technical Remarks 1. The wet soil sample should be kept loosely in the container. 2. Care should be taken to avoid over-heating of the soil sample by maintaining the oven temperature at 105-110 °C. 3. Dry soil sample should not be left uncovered before weighing. 4. To determine the moisture content of litter and humus samples, dry samples at 70 °C for 48 h. 5. Moisture content in air-dry is called hygroscopic moisture. It varies from less than 0.2% for sand to more than 8% for similar with leaf litter/OM, depending upon the relative humidity in the storage area, and fineness of soil particles. Samples should be air-dried prior to moisture content determination. 6. Moisture content values reproducible to within ± 0.5 % can be achieved. 7. The oven is monitored periodically to ensure that temperature fluctuation does not exceed 5 oC. 8. The water content at field capacity, wilting point, and the hygroscopic coefficient are all based on the oven-dry reference mass. The percentage of water held under each of these conditions can therefore be used to define the following and other forms of soil water. Each of these forms of water can be calculated from the appropriate soil mass. Capillary water (%) = Field capacity - Hygroscopic coefficient Available water (%) = Field capacity – Wilting point Unavailable water (%) = Wilting point Gravitational water (%) = Water content – Field capacity - 27 - 4.2. Water Holding Capacity The water-holding capacity (WHC) is defined as the amount of water held in the soil after the excess gravitational water has drained away and after the rate of downward movement of water has materially ceased. Stage of field capacity is attained in the field after 24 to 72 hours of saturation; this is the upper limit of plant-available soil moisture. We must distinguish between soil water content, (the percent water on an oven-dry weight basis), and the soil water potential (the energy status of water in the soil), which is usually expressed in pressure units (Pascal or bar). However, as indeed we are dealing with a tension – a negative pressure - units are usually considered to be negative. Apparatus Polythene sheets Funnel (glass or plastic) Spade Tubing (to attach to bottom of funnel) Soil auger Clamp (to secure tubing) Moisture boxes/cans Filter paper (to line funnel) Balance Beakers (250-mL) Oven Graduated cylinder Ring stand Stirring rod (long) Procedure A. Field Processing 1. Select a uniform plot measuring (5 m x 5 m) and make a flat and horizontal area. 2. Remove any loose material from the surface (weeds, pebbles, etc.). 3. Make bunds around the plot. 4. Fill sufficient water in the plot to completely saturate the soil. 5. Cover the plot area with a polythene sheet to check evaporation. 6. Take soil sample from the centre of the plot from the desired layer, starting after 24 h of saturation and determine moisture content daily till the values of successive days are nearly equal. 7. Record the weight of the oven-dry soil. 8. Repeat above on next day and so on till a constant oven-dry soil value is reached. - 28 - B. Laboratory Processing 1. Thoroughly air-dry compost and soil samples. 2. Attach and clamp tubing to bottom of funnel and attach funnel to ring stand. 3. Place filter paper in funnel. 4. Fill funnel with the 100 mL sample – do not compact. 5. Measure out 100 mL of water using the graduated cylinder. 6. Gradually add water to the sample until covered. Record the amount of water added. 7. Stir gently and let sit until sample is fully saturated. 8. Release the clamp and collect excess water in the graduated cylinder (water drained, mL). 9. Record the amount of water in the cylinder. 10. Calculate how much water was retained in the 100-mL sample of compost, soil or compost/ soil mixture and then calculate the water-holding capacity. Calculations – Note Water-holding capacity is expressed as the amount of water retained per liter of soil, so the next step is to multiply by 10 to convert from the 100 mL sample to the full liter. Technical Remarks 1. Estimates of soil WHC, wilting point and texture can be made from the saturated moisture content. The method is generally reproducible within ± 12%, dependent on the soil textural class. 2. Plot the daily readings on a graph paper. The lowest reading is taken as the value of field capacity of the soil. - 29 - 4.3. Particle Size Distribution Individual soil particles vary widely in any soil type. Similarly, as these particles are cemented together, a variety of aggregate shapes and sizes occur. For standard particle size measurement, the soil fraction that passes a 2-mm sieve is considered. Laboratory procedures normally estimate percentage of sand (0.05 – 2.0 mm), silt (0.002 – 0.05 mm), and clay (10 mm), coarse (5-10 mm); medium (2-5 mm); fine (1-2 mm); and very fine (0.5 g. However, if the sample aliquot to be assayed is less than 0.5 g, a 40-mesh screen should be utilized. After grinding, the sample should be thoroughly mixed and a 5 to 8 g aliquot withdrawn for analyses and storage. Notes Using a brush or vacuum system, clean the grinding apparatus after each sample. Uniform grinding and mixing are critical in obtaining accurate analytical results. - 140 - Exercise care when grinding very small samples or plant material that is pubescent, deliquescent, or that has a fibrous texture. These samples are difficult to grind in Wiley mills and the operator should allow sufficient time for the sample to pass through the screen to ensure homogeneity. In these instances, Cyclotec or equivalent high-speed grinders are preferable. Most mechanical mills contribute some contamination of the sample with one or more elements. The extent of contamination depends on condition of the mill and exposure time Use stainless steel for cutting and sieving surfaces to minimize contamination. Routine maintenance should be made on mills to ensure optimum operating conditions. Cutting knives or blades should be maintained in sharp condition and in adjustment. Avoid cross-contamination from one sample to the next by cleaning the sample mill thoroughly with a dry brush or by using dry air under pressure. If the plant sample is big enough, the mill can be rinsed with the material to be grinded. When sampling mixed stands particularly forages and pastures, separate plant species. Similarly, the sample should be of only leaves or petioles or whole tops and not mixtures. Storage After grinding and mixing (homogenization), samples should be stored in conditions that minimize deterioration and maintain sample integrity for weighing and follow-up analytical work. Apparatus Airtight plastic storage containers Storage cabinet located in cool, dark, and moisture-free environment Refrigerator Procedure After grinding and homogenization, a representative sub-sample is taken from the ground plant material for analyses and storage, which should be placed in a container that can be securely sealed. Containers should then be placed in a cool, dry place for storage. For long-term storage, ground samples should be thoroughly dried, sealed, and placed under refrigerated conditions (4 oC) until the required analysis can be completed. The dried and milled samples should be stored in a cool and dry place in flasks with tight stoppers or in sealed polyethylene bags, protected against direct sunlight. During storage, the plant material may attract moisture so that the drying procedure must be repeated just before weighing out a sample for analysis. Dry the sample 1 hour on 70 oC before analysis. Technical Remarks 1. If samples are placed in a cool (4 oC), dark, dry environment, storage life is indefinite. 2. Small manila envelopes can also be used for sample storage, but care must be taken to prevent absorption of moisture. Collect the ground sample in the envelope and immediately place in a desiccator cabinet or desiccator to minimize moisture absorption. - 141 - 7. Plant Analysis After soil testing, plant analysis is critical to improving crop nutrition and yield. From the nutritional standpoint, plant analysis is based on the principle that the concentration of a nutrient within the plant is an integral value of all the factors that have interacted to affect it. The principles and procedures used for plant analyses have evolved over many years and changed as knowledge increased about each element that is essential for a plant to complete its life cycle. As such, the use of plant analyses has become an integral part of most agronomic research and a tool for crop consultants and fertilizer dealers to monitor production fields. The concentration of nutrients in plant tissues can be measured in a plant extract obtained from fresh plant material, (i.e., tissue analysis), as well as in whole dried plant material. The former test is qualitative and is appropriate only for quick measurements on a growing crop. Total plant analysis is quantitative in nature and is more reliable and useful. Generalized ranges of deficiency, adequacy, and excess of nutrient- concentrations in cereal crops are given in the Appendix 11. Of prime concern are forms of N, as well as P, B, and micronutrient cations. More detailed interpretative guidelines for plant analysis data are available in Reuter and Robinson (1986, 1997), Munson and Nelson (1990) and Jones et al. (1991). Effective Uses for Plant Analyses Plant analysis is an effective management strategy for a sustainable soil fertility program because it provides a direct measure of nutrient concentrations and balance within the plant. Therefore, the effective uses for plant analysis are as follow: Confirm a diagnosis made from visible symptoms Identify “hidden hunger” where no symptoms are apparent Locate soil areas where deficiencies of one or more nutrients occur Determine whether applied nutrients have entered the plant Indicate among various nutrients Study the internal functioning of nutrients in plants Suggest additional tests or studies in identifying a crop production problem. - 142 - 7.1. Moisture Factor Weighing of oven-dried samples is, however, cumbersome (involves continuous oven-drying and use of desiccators, and is still prone to error), as plant material may absorb moisture during the weighing process, particularly if the relative humidity is high in the laboratory. To get around this difficulty, use moisture factor instead. The moisture factor for each batch of samples can be calculated as follows: by oven-drying only a few sub-samples from the lot (say about 5 from a batch of 100 – 200 samples). Thereafter, air-dry samples are weighed, considering the moisture factor. 7.2. Nitrogen Plants need a wide range of proteins to grow, develop and mature. The main body of protein is amino acids and nitrogen (N) is the major component of amino acids. Nitrogen is also present in chlorophyll. Soil micro-organisms feed on soil N during breakdown of organic materials. Nitrogen improves quality of leafy vegetables. It promotes rapid growth and if the supply is out of balance with other nutrients flowering and fruiting may be delayed. The N content of plant dry matter generally ranges from 1 to 5 %. However occasionally it may be either lower or higher than this range. The common plant analysis is that of N by Kjeldahl method. However, wet ashing with H2SO4 and H2O2 is also used for eliminating the standard use of selenium (Se) in the former method. 7.2.1. Kjeldahl Nitrogen (with Catalyst) Apparatus Block-digester Automatic titrator connected to a pH- meter Distillation unit Vortex tube stirrer Reagents A. Catalyst Mixture (K2SO4-Se), 100:1 w/ w ratio Grind reagent-grade chemicals separately and mix. If caked, grind the mixture in a porcelain pestle and mortar to pass a 60-mesh screen (0.250 mm), taking care not to breathe Se dust or allow Se to come in contact with the skin. B. Sulfuric Acid (H2SO4), concentrated (98 %, sp. gr. 1.84) C. Sodium Hydroxide Solution (NaOH), 10 N Dissolve 400 g NaOH in DI water, transfer to a 1-L heavy-walled Pyrex flask, let it cool, and bring to volume with DI water. D. Boric Acid Solution (H3BO3), saturated Add 500 g H3BO3 to a 5-L flask. Add 3 L DI water, and swirl vigorously. Leave overnight. - 143 - Note There should always be solid H3BO3 on the bottom of the flask. E. Tris Solution [hydroxymethyl aminomethane] (C4H11NO3), 0.01 N Dry reagent-grade Tris in an oven at 80 °C for 3 hours, cool in a desiccator, and store in a tightly stoppered bottle. Dissolve 1.2114 g Tris in DI water, transfer the solution to a 1-L flask, and bring to volume with DI water. F. Sulfuric Acid Solution (H2SO4), 0.01 N Add 28 mL concentrated H2SO4 to about 600 – 800 mL DI water in a 1-L flask, mix well, let it cool, and bring to volume. This solution contains 1 N H2SO4 solution (Stock Solution). Pipette 10 mL Stock Solution to 1-L flask, and bring to volume with DI water. This solution contains 0.01 N H2SO4. G. Standard Stock Solution Dry reagent-grade ammonium sulfate (NH4)2SO4 in an oven at 100 °C for 2 hours, cool in a desiccator, and store in a tightly stoppered bottle. Dissolve 5.6605 g dried (NH4)2SO4 in DI water, and bring to 1-L volume with DI water. This solution contains 1.2 g NH4-N/L (Stock Solution). Procedure A. Pre-treatment 1. Mix and spread finely ground (Cyclone mill) plant sample in a thin layer on a sheet of paper until it looks uniform. 2. Select representative sub-samples of about 1 g by taking at least 10 small portions from all parts of the sample with a spatula, and put them into a plastic vial. 3. Dry the sub-sample at 60 °C in an oven (overnight), and then cool in a desiccator. B. Digestion 1. Weigh 0.25 g (grain) or 0.50 g (straw) of dry plant material, and transfer quantitatively into a 100-mL digestion tube. 2. Add a few pumice boiling granules, and add about 3 g catalyst mixtures using a calibrated spoon. 3. Add 10 mL concentrated H2SO4 using a dispenser, and stir with Vortex tube stirrer until mixed well. 4. Place tubes in a block-digester set at 100 °C for 20 minutes, and remove the tubes to wash down any material adhering to the neck of the tube with the same concentrated H2SO4. 5. Thoroughly agitate the tube contents, and then place the tubes back on the block-digester set at 380 °C for 2 hours after clearing. 6. After digestion is complete, remove tubes, cool, and bring to 100-mL volume with DI water. 7. Each batch of samples for digestion should contain at least one reagent blank (no plant), and one chemical standard (weigh 0.1 g EDTA standard digest), and one standard plant sample (internal reference). - 144 - C. Distillation 1. Before starting a batch for distillation, the distillation unit should be steamed out for at least 10 minutes. Adjust steam rate to 7 – 8 mL distillate per minute. Water should flow through the condenser jacket at a rate sufficient to keep distillate temperature below 22 °C. 2. Calibrate pH meter with buffer solutions of pH 7.0 (buffer), and 4.0 (sensitivity), after setting for temperature. Then standardize the 0.01 N H2SO4 in the Auto-Titrator by titrating three separate 10-mL aliquots of the primary standard, 0.01 N Tris solution, to pH 5.0. The titrations should agree within 0.03 mL; if not; titrate further aliquots until agreement is found. The H2SO4 normality is: 3 Carry out distillations as follows: Dispense 1 mL saturated H3BO3 solution and 1 mL DI water into a 100-mL Pyrex evaporating dish, placed underneath the condenser tip, with the tip touching the solution surface. Pipette 10-mL aliquot into a 100-mL distillation flask. Carefully add 10 mL 10 N NaOH solution, and immediately connect the flask to the distillation unit and begin distillation. Collect about 35 mL distillate in the collecting dish. Remove distillation flask and connect an empty 100-mL distillation flask to the distillation unit. Drain water from the condenser jacket and steam out apparatus for 90 seconds before connecting the next sample. Titrate the distillate to pH 5.0 with standardized 0.01 N H2SO4 using an Auto-Titrator; record titration volume of acid. Each distillation should contain at least two standards (pipette 10 mL digested solution), two blanks (pipette 10 mL digested solution). Recovery of N should be at least 96 %. Notes: After finishing titration, wash the Teflon-coated magnetic stirring bar, the burette tip, and the combined electrode into the dish. Between different samples, steam out the distillations. Disconnect distillation flasks containing the digest sample and NaOH, and attach a 100-mL empty distillation flask to distillation unit. Place a 100-mL empty beaker underneath the condenser tip, turn off cooling water supply (drain the water from the condenser jacket), and steam out for 90 seconds. Each distillation should contain at least two standards and two blanks (reagent blanks). - 145 - Calculations – – – Where: V = Volume of 0.01 N H2SO4 titrated for the V1 = Volume of NH4-N standard solution sample (mL) (mL) V2 = Tolat volume of the plant digest (mL) Wt2 = Weight of dry plant (g) V3 = Volume of plant digest used for N = Normality of H2SO4 solution distillation (mL) C = Concentration of NH4-N standard B = Distillate blank titration volume (mL) solution (μg/mL) B1 = Digested blank titration volume (mL) 14.01 = Atomic weight of N Wt1 = Weight of EDTA (g) 186.1 = Atomic weight of EDTA Technical Remarks 1. Samples should contain about 1 mg of N (but no more than 5 mg). The sensitivity of the procedure depends upon a number of factors, including sample weight, strength of the acid, and accuracy of titration. 2. If the ratio of acid to salt is low at the end of the digestion step, a significant amount of NH 3 may be volatilized during the digestion process. Other situations which may cause N loss during digestion and should be avoided are: localized heating in the digestion flask (temperatures above 410°C), and the use of 30% hydrogen peroxide (H2O2) as an oxidant. 3. The time required for digestion is affected by the catalyst, temperature, and type of plant tissue. It is important to allow an equal length of additional time after the sample clears. As much as 10% of the organic N may not yet be converted to NH4 at clearing. 4. Homogeneity of the sample is important for precision. For best results, dried tissue should pass a 40-mesh sieve. In cases where sample size is less than 0.25 g, special care should be taken to insure sample homogeneity. 5. Digested samples may be stored for several days, provided samples are covered and placed in a cool area. 6. Samples distilled into the H3BO3 solution should be titrated within a short time to avoid absorption of atmospheric CO2. - 146 - 7.2.2. Kjeldahl Nitrogen (Without Catalyst) The routine use of heavy metals as catalysts can be harmful environmentally. Therefore, treatment of plant material with a mixture of H2O2-H2SO4 in the absence of metal catalysts is proposed as an alternative digestion procedure for replacing the standard Kjeldahl-N determination in soils and plants. Reagents Sulfuric Acid (H2SO4), concentrated Hydrogen Peroxide (H2O2), 30% Procedure 1. Weigh 0.5 g dry-plant material into a 100-mL digestion tube. 2. Add 3-4 pumice boiling granules, and then add 5 mL concentrated H2SO4, mix well. 3. Keep overnight. 4. Heat on a block-digester at a moderate temperature 100 – 150 °C. 5. Swirl to restrict foaming. If foaming enters the neck of the digestion tube, add 2 mL 30 % H2O2. 6. Heat the tubes for 30 – 60 minutes on the block-digester. 7. Cool the tubes, and then add 2 mL 30 % H2O2. 8. Raise the temperature of the block-digester to 280 °C. 9. Heat the tubes for 10 minutes at 280 °C. 10. Cool, then add 2 mL 30 % H2O2, and heat for 10 minutes. 11. Repeat Steps 9 and 10 until solution remains clear after 10 minutes of heating. 12. Cool, and make up to 100-mL volume with DI water. Measurement 1. Nitrogen content can be measured in this digest by the Distillation Method (Kjeldahl unit). 2. Phosphorus can be done colorimetrically as be used for P measurement in plants, after filtering the digest through Whatman No. 1 or No. 5 filter paper. Note The results for both N and P are highly correlated with the standard Kjeldahl digestion method. - 147 - 7.2.3. Total Nitrogen (Sulfuric-Salicylic Acid Mixture) This method is based on digestion of plant material in a sulfuric-salicylic acid mixture (Buresh et al., 1982). Reagents A. Sulfuric-Salicylic Acid Mixture (concentrated H2SO4 containing 2.5 % w/v salicylic acid) Dissolve 62.5g reagent-grade salicylic acid (C7H6O3) in 2.5 L concentrated H2SO4. B. Catalyst Mixture (K2SO4 - Se), 100:1 w/w ratio Grind reagent-grade chemicals separately and mix. If caked, grind the mixture in a porcelain pestle and mortar to pass a 60-mesh screen (0.250 mm), taking care not to breath Se dust or allow Se to come in contact with skin. C. Sodium Thiosulfate (Na2S2O3.5H2O), crystal D. Ethylene Diaminetetraacetic Acid Disodium Salt (EDTA), M.W. = 372.2 Procedure A. Pre-treatment 1. Mix and spread finely ground plant sample in a thin layer, on a sheet of paper or plastic until the sample looks uniform. 2. Take a representative sub-sample of about 1 g by systematically withdrawing at least 10 small portions from all parts of the sample with a spatula, and put them into a plastic vial. 3. Dry the sub-sample at 60 °C in an oven (overnight), and then cool in a desiccator. B. Digestion 4. Weigh 0.25 g (grain) or 0.50 g (straw) dry-plant material, and then transfer quantitatively into a dry 250-mL digestion tube. 5. Add 20 mL sulfuric-salicylic acid mixture while rotating the tube to wash down any sample adhering to the neck of the tube, and allow to stand 2 hours or longer with occasional swirling. 6. Add 2.5 g sodium thiosulfate through a long-stemmed funnel to the contents of the tube and swirl gently a few times, and allow to stand overnight. 7. Add 4 g catalyst mixture, and 3 – 4 pumice boiling granules, and place tubes on the block- digester pre-heated to 400 °C. 8. Place a small glass funnel in the mouth of the tubes to ensure efficient refluxing of the digestion mixture and prevent loss of H2SO4, and proceed with the digestion until the mixture clears. 9. Remove the tubes from the block-digester and allow them to cool for about 20 minutes. Then wash down any material adhering to the neck of the tube with a minimum quantity of DI water. 10. Thoroughly agitate the tube contents, place tubes back on the block-digester, and digest for 2 hours after clearing. No particulate material should remain in the tube after digestion. 11. After the digestion is finished, allow the digest to cool, and add water slowly shaking until the liquid level is about 2-cm below the graduation mark. 12. Allow tube to cool to room temperature, and add DI water to bring the volume to the 250- mL mark. 13. Each batch of samples for digestion should contain at least one reagent blank (no plant), and one chemical standard (weigh 0.1 g EDTA standard digest), and one standard plant sample (internal reference). - 148 - C. Distillation 1. Before starting a batch for distillation, the distillation unit should be steamed out for at least 10 minutes. Adjust steam rate to 7 – 8 mL distillate per minute. Water should flow through the condenser jacket at a rate sufficient to keep distillate temperature below 22 °C. 2. Calibrate pH meter with buffer solutions of pH 7.0 (buffer), and 4.0 (sensitivity), after setting for temperature. Then standardize the 0.01 N H2SO4 in the Auto-Titrator by titrating three separate 10-mL aliquots of the primary standard, 0.01 N Tris solution, to pH 5.0. The titrations should agree within 0.03 mL; if not, titrate further aliquots until agreement is found. The H2SO4 normality is: 3. Carry out distillations as follows: Dispense 1 mL saturated H3BO3 solution and 1 mL DI water into a 100-mL Pyrex evaporating dish, placed underneath the condenser tip, with the tip touching the solution surface. Prior to distillation, shake the digestion tube to thoroughly mix its contents, and pipette an aliquot in a 300-mL distillation flask. Carefully add 7 mL 10 N NaOH solution for 25-mL aliquot (or 15 mL 10 N NaOH solution for 50-mL aliquot), and immediately connect flask to distillation unit and begin distillation. Collect about 35-mL distillate in the collecting dish. Remove distillation flask and connect an empty 100-mL distillation flask to the distillation unit. Drain water from the condenser jacket and steam out apparatus for 90 seconds before connecting the next sample. The distillate is then titrated to pH 5.0 with standardized 0.01 N H2SO4 using an Auto- Titrator; record titration volume of acid. Each distillation should contain at least two standards and two blanks (reagent blanks). Recovery of EDTA, corrected for reagent blank, should be at least 97%. Notes: After finishing titration, wash the Teflon-coated magnetic stirring bar, the burette tip, and the combined electrode into the dish. Between