Himanshu Thesis PDF
Document Details
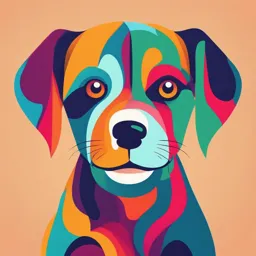
Uploaded by WellMadeIndianArt
Himanshu
Tags
Summary
This thesis explores desiccant integrated cooling systems, focusing on their operation, methodology, data analysis, and results. It examines the cooling load, heat gains, and simulations related to different desiccant channel geometries. The study investigates the impact of various factors on the efficiency of dehumidification.
Full Transcript
INDEX S. NO. PARTICULARS PAGE NO. CERTIFICATE I CERTIFICATE OF APPROVAL III A...
INDEX S. NO. PARTICULARS PAGE NO. CERTIFICATE I CERTIFICATE OF APPROVAL III ACKNOWLEDGEMENT IV PLAGIARISM DECLARATION V PRAPATRA VI LIST OF TABLES VII LIST OF FIGURES VIII LIST OF GRAPHS IX ABSTRACT 1 1 INTRODUCTION 2-14 1.1 The desiccant integrated cooling system's operation concept 4 1.2 Problem definition 5 1.3 The work's purpose 5 1.4 Range of the Work 5 1.5 An initial investigation has been completed 6 1.6 The following methodology has been used 7 1.7 Measuring Devices and Instrumentation 8 2 Literature Review 15- 26 2.1 Principle of Operation for Solid Desiccant Cooling Systems 25 3 METHODLOGY 27- 52 3.1 EVALUATION OF COOLING LOAD 28 3.1.1 Primary EVALUATION OF COOLING LOAD 28 3.2 Cooling Load Components 32 3.2.1 Component Loading 33 3.3 Heat Gains 33 3.3.1 Heat Transfer via Transparent Surfaces 33 3.4 The overall heat transfer coefficient 34 3.5 Determination of Cooling Load Temp. Difference (CLTD) 37 3.6 Heat Gain Through Glass 39 3.7 Transmission Heat Gain Through Glass 39 3.8 Solar Radiation Heat Gain 39 3.9 Occupant Heat Gain 40 3.1 Heat Gain from Lighting Equipment 42 3.11 Heat Gain from Office Equipment 43 3.12 Office Equipment Heat Gain Rate 43 3.13 Infiltration-Induced Heat Gains 43 3.14 Total Cooling Load Calculation for Office 44 3.15 Calculation of Total Room Sensible Heat Gain 45 Calculation of Heat Gain Through Aluminum Section of 3.15.1 Partition Wall 46 3.16 System Operation 49 3.17 Dehumidifier with Desiccant Technology 52 4 DATA ANALYSIS 53- 71 4.1 Exploration of Different Desiccant Channel Geometries 57 4.2 Methodology for Modeling 61 4.3 Study on the independence of the mesh and model 62 4.5 Material Definition 66 4.6 Model validation 67 4.7 Results and Discussion 68 4.8 Analysis of results from different channel geometries 68 4.9 channel geometries under Outdoor-I supplying conditions 71 RESULTS 72-77 Adsorption Process in Desiccant Wheel 76 DISCUSSION 78-79 CONCLUSION 80-82 FUTURE SCOPE 83-84 REFERENCES 85-96 JOURNAL PLAGIARISM REPORT LIST OF TABLES TABLE NO. PARTICULARS PAGE 3.1 Building Local on and Meteorological Information for BHOPAL City 28 3.2 Climatological data for temp. & humidity in BHOPAL 30 3.3 Data on Building Structure 31 3.4 Shows the fenestration-related overall heat transfer coefficient 35 3.5 Air Film Surface or Film Conductance 36 3.6 Thermal Conductance of Air Space (ka) 36 3.7 CLTD for heavier walls 38 3.8 Latitude Month Correction (LM) Value for 23°N 38 3.9 Corrected CLTD Values for mechanical engineering building 38 3.12 Rate of Heat Gain from Occupant at Conditioned Space 40 3.13 Standard Lighting Load 42 3.14 Office Equipment Heat Gain Rate (Watts) 43 3.15 the specified value of AC represents the number of air changes/ hrs 44 Simulation Parameters for Various Desiccant Wheel Channel 4.1 Geometries 61 4.2 Mesh size comparison for various channel shapes 64 4.3 Results of simulation under indoor operating conditions 70 5.1 Investigation of temperature and humidity levels at various states 75 LIST OF FIGURES FIG. PAGE NO. PARTICULARS NO. 1.1 The desiccant aided cooling concept in action 4 1.2 Diagram of the hybrid desiccant cooling system 6 1.3 DC gear motor equipped with a pulley designed 8 1.3.1 Regulate the speed of the DC motor on your desiccant wheel 9 1.3.2 Enthalpy Wheel for Heat Recovery 9 1.3.3 DC gear motor designed for use with an enthalpy wheel 9 1.3.4 Switch to control the speed regulator of a DC motor 10 1.3.5 Electric heater heat controller with voltage regulator dimmer 10 1.3.6 An electric air heater of the M type 10 1.3.7 Supply fan is designed to circulate cool air for the cooling system 11 1.3.8 Exhaust fan is utilized to supply process air 11 1.3.9 Control the speed of the AC suction and exhaust fan 11 1.3.10 System for receiving data using Arduino 12 1.3.11 To precisely measure temperature and humidity, use digital DHT-22 sensors 12 1.3.12 Electronic Wind Speed Meter 13 1.3.13 Electricity meter for two-wire AC power systems in phase 2 13 Reciprocating compressor with a condenser coil designed with R134A 1.3.14 refrigerant 13 1.3.15 An Evaporator coil 14 2.1 Study on the Operation of a Liquid Desiccant Cooling System 25 2.2 Analysis of Solid Desiccant Cooling System Functionality 26 3.1 An Office Cabin for Calculating Cooling Load 32 3.2 Components of the load 33 3.3 Analysis of Heat Transfer Across Opaque Surfaces 34 3.4 Heat Transfer in Composite Wall with Air Space 34 3.5 Heat Gain Through Glass 39 3.6 illustrating a solid desiccant cooling system with hybrid capabilities 50 3.7 Operational Functionality of Hybrid Desiccant Cooling System 51 3.8 Implementation of Hybrid Desiccant Cooling System 52 4.1 Portrayed the geometry of rotary desiccant wheels 55 4.2 Triangular 57 4.2.1 Square 58 4.2.2 Hexagonal 58 4.2.3 Sinusoidal 1 59 4.2.4 Sinusoidal 2 59 A Computational Fluid Dynamics (CFD) simulation methodology is 4.3 employed 62 4.4 Meshing in computational domains with different geometric shapes 63 4.5 Comparison of air temperature magnitude in various channel geometries 65 A comparison is made between the current Computational Fluid Dynamics 4.6 (CFD) 66 4.7 Impact of temperature and relative humidity on different geometries 70 4.8 Psychometric diagram illustrating dehumidificaon differences 71 LIST OF GRAPHS GRAPH NO. PARTICULARS PAGE 4.1 The outlet air temp. of adsorption & the relative humidity 0f the inlet air 67 4.2 Psychometric diagram illustrating dehumidification differences 71 5.1 Various ambient temperatures and regeneration temperatures 73 5.2 Investigating the impact of humidity levels on ventilation systems 74 5.3 Investigating the impact of changes in ambient temperature 0n the temp 74 5.4 Analyzing the operational modes of process air and regeneration section 76 5.5 The efficiency of dehumidification in recirculation and ventilation modes 77 ABSTRACT Background: The traditional energy s0urces used for c00ling and air c0nditioning systems have had detrimental effects on the environment, contributing to global warming and ozone depletion. The increased demand for energy due to global population growth has intensified the strain on resources. Conventional systems lack humidity control, leading to issues with comfort and indoor air quality. Sustainable technologies, such as absorption and desiccant cooling systems, offer environmentally friendly alternatives. The development of energy-efficient cooling technologies is crucial for mitigating environmental impacts and reducing energy strain. Materials and Methods: The methodology and evaluation of cooling load for ind00r spaces, focusing on temperature control, humidity, and air quality. The ideal of relative moisture and the temperature inside levels are typically set at 22.77 ºC to 26.10 ºC with a relative humidity of 50% during the summer conditions, and 22.12 ºC to 22.23 ºC with a relative humidity of 20% to 30% for winter conditions. Additionally, it details the calculation of heat gain through glass, occupant heat gain, lighting equipment, infiltration-induced heat gains, and ventilation requirements. Also described is the way a hybrid air conditioning system that combines solid-desiccant vapor and compression works. Results: The impact of ambient temperature on humidity ratio during dehumidification, focusing on the desiccant wheel, enthalpy wheel, and evaporating coil outlets. It explores the decrease in humidity ratio with constant regeneration temperature, the effect of desiccant material attracting moisture, and the decrease in moisture removal rate. The study also compares the efficiency of dehumidification in recirculation and ventilation modes under varying temperatures. Conclusion: The study conducted a CFD simulation of an adsorption cooling system using a desiccant dehumidifier packed with silica gel. Different desiccant channel geometries were compared, with the sinusoidal geometry showing superior performance. The CFD simulation was utilized to assess the system’s performance under various climatic conditions. Results indicated that the sinusoidal geometries were most effective in dehumidification. The study demonstrated the effectiveness of CFD simulation in predicting water vapor uptake and off-take, reducing design time and costs for adsorption cooling systems. Keywords: Energy-efficient cooling technologies, Absorption cooling systems, Desiccant cooling systems, Environmental impact mitigation, Indoor air quality, Humidity control, Temperature control, Heat gain calculation, Solid-desiccant vapor-compression hybrid air- conditioning system, Dehumidification, Desiccant wheel, Enthalpy wheel, Evaporating coil Page | 1 CHAPTER 1 INTRODUCTION Page | 2 Nowadays, conventional energy sources, such as heat engines and electricity, are commonly employed to generate CFC-based cooling and air conditioning systems for various cooling purposes. Unfortunately, the utilization of these conventional cooling and air conditioning systems has had detrimental effects on the environment. The generation of electricity from conventional power plants has significantly contributed to the decline of the global environmental wealth, thereby exacerbating the issue of global warming. Furthermore, the usage of such conventional systems has led to elevated levels of CFCs in the environment, resulting in ozone depletion. Moreover, the ever-increasing global population, along with their desire for an enhanced standard of living and the adoption of advanced technologies, has amplified the demand for energy. Consequently, the strain on energy resources has intensified. In traditional vapor compression systems, the same air-handling equipment is responsible for managing both the sensible and latent heat loads within a given room. However, these systems solely regulate the dry-bulb temperature (DBT) of conditioned air, while the control of humidity is treated as a separate aspect of temperature control. Despite the prevalence of high ambient moisture levels in humid climates, there exists no direct regulation over humidity levels in these systems. This inherent constraint in system design leads to the achievement of the desired DBT of conditioned air, but at the cost of the relative humidity falling beyond the range of comfort. Consequently, the lack of humidity control can result in the growth of mold and the pursuit of optimal indoor air quality and thermal comfort via vapor compression air-conditioning systems leads to substantial consumption of electrical energy. Consequently, comfort conditions can only be maintained in traditional systems when the sensible heat ratio (SHR) exceeds 0.75. However, in hot and humid climates, this value can be significantly lower, rendering the typical system incapable of providing optimal comfort conditions. Therefore, due to the requirement of SHR to be greater than 0.75, thermal comfort conditions cannot be adequately achieved in traditional systems. In response to these issues, there has been a growing interest in developing sustainable, energy-efficient cooling and air conditioning technologies that reduce the environmental impact of conventional systems. One such technology is the use of absorption cooling systems, which utilize a heat source such as solar energy or waste heat to drive the cooling process. These systems do not rely on electricity or CFCs, making them environmentally friendly alternatives to conventional cooling systems. Another promising technology is the use of desiccant cooling systems, which remove moisture from the air before cooling it. This allows for better control over humidity levels and can result in more energy- efficient cooling. Additionally, advancements in variable refrigerant flow systems and evaporative cooling technologies are also helping to improve the efficiency and sustainability of cooling systems. Overall, the development of sustainable cooling and air conditioning technologies is crucial in mitigating the environmental impact of conventional systems and reducing the strain on energy resources. By embracing these advancements, we can move towards a more sustainable future while still meeting the growing demand for cooling and air conditioning. One drawback associated with the traditional approach is the release of condensate into the air-conditioned space caused by excessive cooling. Consequently, this can result in elevated humidity levels. On the other hand, a rotating desiccant dehydrator is the main part of desiccant dehumidification and cooling systems. These systems may effectively replenish the desiccant dehumidifier using both electrical energy and low-grade thermal energy sources, including as solar and waste heat. Furthermore, renewable energy sources, such as solar panels, can power the fans and water pump. Page | 3 The decoupling of sensible and latent cooling loads allows this system to operate across a wide range of SHR. Therefore, desiccant-based air conditioning and dehumidification systems have garnered attention for their ability to reduce air humidity and temperature. Consequently, the desiccant cooling system presents itself as a viable, energy-efficient, environmentally friendly, and cost-effective alternative to conventional climate control systems. In essence, a desiccant is a hygroscopic substance that either induces or maintains a state of dryness in its immediate vicinity. Desiccants can be natural or synthetic materials that have the capability to absorb or adsorb water vapor due to a difference in the water vapor pressure between the surrounding air and the surface of the desiccant. This drying cooling system not only ensures optimal indoor comfort but also minimizes thermal energy consumption, reduces electricity usage, and facilitates the development of innovative materials to enhance the efficiency of desiccant cooling technology. 1.1 The desiccant integrated cooling system's operation concept. Desiccant cooling systems utilize a variety of drying materials to eliminate moisture from the air via a natural process referred to as sorption. Regenerating the desiccant dehumidifier with these methods is possible even with low-grade thermal energy sources like waste heat and solar radiation. Furthermore, renewable energy sources, such as solar panels, can power the fans and water pump. in Figure 1.1. FIGURE 1.1 The desiccant aided cooling concept in action is shown in. The desiccant combined dehumidification and cooling method for comfort climate control can be classified as liquid or solid, depending on the type of desiccant material utilized. Page | 4 1.2: Problem definition 1. To create and produce a hybrid solid desiccant cooling system at a reasonable cost. 2. A research on the cooling load of a commercial building's office cabin. 3. Using CFD simulation methodologies, numerical simulation is performed for various desiccant-based channel geometry forms. Previous theoretical investigations provide additional validation for the available performance simulation. 4. Used several techniques to test hybrid desiccant cooling systems under various climate scenarios. 5. The hybrid desiccant cooling system's performance results from various modes of operation are contrasted with one another. 6. A comparison is made between the system's experimental results and the CFD simulation. 1.3. The work's purpose The design and development of a half-breed desiccant cooling system for small office cabins in commercial buildings requires careful consideration of the various channel geometries based on desiccant fabric. This process takes time and effort to identify the optimal geometry. The goals of this effort are the following. 1. To perform a sensible and idle warm stack cooling stack evaluation of the office cabin available at LNCT Designing College BHOPAL. 2. Using Ansys-CFX, calculate the channel geometry of various types of desiccant dehumidifiers with a range of working parameters using CFD, and compare the simulation results with exploratory patterns. 3. This study aims to evaluate the performance of a desiccant dehumidifier under specific operating conditions, such as tall temperature variations, increased mugginess, and crossover capillary chilling framework. 1.4 Range of the Work Analyzing the cooling load of the office cabin will help in the design of the desiccant wheel to determine the amount of CFM supply air required to maintain the office cabin comfortable condition. The software for modeling computational fluid dynamics The desiccant wheel was utilized in the design and production of the desiccate cooling mechanism to obtain results. The numerical performance of various desiccant channel geometries was determined using ANSYS CFX, and the optimal performance of the geometries was compared with previous studies. Development of a hybrid adsorption cooling system for small office buildings. In BHOPAL, Madhya Pradesh, India, experimental research of an adsorption dehumidifier for humidifiers and dehumidifier in a hybrid adsorption cooling apparatus for varying climatic conditions. Page | 5 1.5. An initial investigation has been completed Hard desiccant cooling methods work better than fluid desiccant systems for cooling, according to an assessment of the literature. Another significant factor in lowering the energy usage of traditional cooling systems is solid desiccant. Finding appropriate solid desiccant materials and their effectiveness in desiccant wheels is recommended by the literature review. The choice of desiccant wheel geometry determines the wheel's optimal performance. Based on the aforementioned synopsis, a fascinating research void emerges about the experimental or numerical assessment of the desiccant channel geometry's performance. The sophisticated solid desiccant hybrid refrigeration system can also be used for experimental research during the validation gap, as illustrated in Figure 1.2, particularly for tiny office cubicles. Following initial research, the following changes are suggested to enhance the hybrid solid desiccant cooling system's functionality. The dehumidification performance of various desiccant channel shapes. Process numerical simulation with CFD aids in choosing the proper channel geometry and enhances system performance. The workings of a basic hybrid solid desiccant cooling system are illustrated in the diagram of the system that is displayed in the figure below. This is utilized to get ready for the hybrid solid desiccant cooling system's actual experimental setup. Figure 1.2. Diagram of the hybrid desiccant cooling system, Page | 6 Point of state 1: Inlet of ambient air to desiccant wheel Point of state 2: Desiccant wheel's air outlet Point of state 3: Air exiting the enthalpy wheel Point of state 4: Evaporating coil's air outlet Point of state 5: Inlet of ambient air Point of state 6: Air intake to enthalpy wheel Point of state 7: Air exiting the enthalpy wheel Point of state 8: Desiccant wheel's air outlet For small-scale commercial office cabins, the unique way the hybrid solid desiccant cooling system operates is beneficial not only for figuring out how the desiccant wheel will be affected during dehumidification under various weather conditions. 1.6. The following methodology has been used. Current research includes cooling load analysis of small office cubicles, performance evaluation of different drying duct geometries using CFD simulation techniques, and various duty cycle performance for dehumidification of process air supplied to small offices. Includes experiments. A private office rooms. The following methodology is used in the current research work: For the above studies on dehumidification process, a detailed literature search was carried out and research gaps were identified. To start, analyze the cooling load in the chosen office cabin to get the desiccant wheel diameter and the CFM needed for the size of the office. Utilizing ANSYS CFX software, numerical simulation of various duct shapes utilized in the dehumidification process of desiccant wheels is done utilizing CFD simulation technology for various operating ambient conditions. Industrial providers make desiccant wheels using performance-optimized, result-based channel designs discovered using CFD simulation techniques. Establish an experimental configuration in the workroom. Various parameters and experimental outcomes were recorded during the experiment using an RTD PT-100 sensor equipped with a data recorder. Air's psychometric characteristics were gathered via a sensor that had an Arduino microcontroller board attached to it. Using input performance characteristics including speed, pressure, and temperature during ventilation mode, assess a hybrid desiccant cooling system's performance under a range of operating environmental circumstances. The laboratory apparatus's regeneration and dehumidification sections receive new ambient air when it is in the ventilation mode. Page | 7 To use input feed performance metrics, such as temperature, pressure, and velocity during recirculation mode, to measure the effectiveness of a hybrid desiccant cooling system under various operating environmental circumstances. The laboratory equipment's regeneration and dehumidification sections receive fresh ambient air when it is in recirculation mode. Examine the differences between the ventilation and recirculation modes' performance outcomes in various climate scenarios. Theoretical outcomes were computed by numerical modeling and simulations, and were contrasted with empirically collected data. 1.7 Measuring Devices and Instrumentation The experiment involves the applica on and measurement of various opera ng performance factors in the system, including temperature, rela ve humidity, and mass flow rate supply. Digital DHT-22 sensors are u lized to measure temperature and rela ve humidity across all sec ons of the experimental setup, test room, and ambient condi ons. The entire data set is gathered and stored using an ARDUINO information gathering system. In the regenera on sec on, the air supply is controlled by suc on and discharge fans, with the speed regulated by an AC speed switch. The air flowrate is monitored using an anemometer, and the temperature for desiccant dehumidifier regenera on is managed by a dimmer heat control switch on an electric heater. The DC gear motor, pulley, and speed regulator switch work together to control the speed of the desiccant wheel in the dehumidifier. The enthalpy wheel consists of square sec on galvanized bunches measuring 1mm x 1mm, and is powered by a DC gear motor with a speed regula ng switch. The accompanying figures detail the instruments and sensors used in the experimental setup, along with their specifica ons. Motor Speed: 60 RPM Voltage: 12V DC Shaft Diameter: 6mm Torque: 3Kg-cm No-load Current: -70mA (Max) DC gear motor equipped with a pulley designed for use with a desiccant wheel. Page | 8 Low Voltage DC Options: At 2A, 1.8V, 3 V, 5 V, 6 V, and 12 V Features PWM-based motor speed controller Maximum Output Power: 30W Adjustable Duty Cycle: Ranges from 0% to 100% Regulate the speed of the DC motor on your desiccant wheel with the control switch. Diameter: 350 mm Length: 100 mm Channel shape: Square Channel size height x length: 1 mm x 1mm Material: Galvanized base Enthalpy Wheel for Heat Recovery 60RPM 12V DC motor Shaft diameter: 6mm Torque: 3 Kg-cm No-load current: -70mA (Max) DC gear motor designed for use with an enthalpy wheel. Page | 9 Voltage of the Input Supply: 6 V-28V DC Highest Power Output: 80 Watt Maximum Continuous Output Current: 3A Adjustable Duty Cycle: 5-100% A switch to control the speed regulator of a DC motor for an enthalpy wheel. Voltage Regulation: AC 0-220 V Size: L85 mm x W60 mm x H40 mm Rotation Hole: 6 mm SCR Framework: BT-800 B Voltage: AC 0-220V Maximum Power: 4000W Electric heater heat controller with voltage regulator dimmer. Voltage: 415 V Diameter: 20 mm Length: 200mm Width: 120mm Power source: Electric tubular heater Material: Stainless steel Material grade: SS 304 Power: 1000 Watts An electric air heater of the M type. Page | 10 Fan dimensions: 19.5 x 19.1 x 11 cm Weight: 800 grams High RPM: 2000 Efficient power consumption: Up to 22 Watts The supply fan is designed to circulate cool air for the cooling system. Fan Dimensions: 19.5 x 19.1 x 11 cm Weight: 800 grams High RPM: 2000 Efficient Power Consumption: Up to 22 watts An exhaust fan is utilized to supply process air to the desiccant dehumidifier. Input Supply Voltage: 110-220V AC Output Voltage Range: 50-220V Maximum Output Power: 2000W Maximum Output Current: 25A Control the speed of the AC suction and exhaust fan for regeneration using the speed regulator switch. Page | 11 Arduino Data Receiving System Configurations Specifications ATmega328P Microcontroller Recommended input voltage range: 7–12 volts; operating voltage: 5 volts Limit of Input Voltage: 6–20V There are 14 digital I/O pins, 6 of which can produce PWM. There are six PWM digital I/O pins and six analog input pins. DC Current for each 3.3V pin: 50 mA; DC Current per I/O pin: 20 mA ATmega328P flash memory: 32 KB (0.5 KB occupied by boot loader). Two KB of SRAM and one KB of EEPROM are available (ATmega328P). Its dimensions are as follows: Length: 68.6 mm, Width: 53.4 mm, Weight: 25 g, Clock Speed: 16 MHz, LED Baudin: 13. System for receiving data using Arduino This system is designed for data reception through Arduino technology. (Relative) Humidity Range: 0-100% with an accuracy of 2-5%; Temperature Range: -40 °C to 125 °C with an accuracy of ±0.5°C Power: range of 3.3 to 6 volt To precisely measure temperature and humidity, use digital DHT-22 sensors. Page | 12 Temperature Range for Wind: -10 to 45°C; Speed Range: 0 to 30 m/s Power Supply: 3V Electronic Wind Speed Meter Voltage: 240 Volts (SG1) Frequency: 50Hz Current: 3200 Imp. (KWh) AC Single phase 2 wire Electricity meter for two-wire AC power systems in phase 2 Material: Copper Iron Intended Use: Designed for residential refrigerators and freezers Power: 0.5-ton capacity with a single-phase motor Voltage: Operates within the range of 120 to 280V AC Condenser Design: Features a heat exchange area with two rows of 2/8” tubes and 12 fins per inch. A single-phase reciprocating compressor with a condenser coil designed for use with R134A refrigerant. Page | 13 The evaporating coil has a manifold size of 1" diameter copper supply and return piping (1" inside- 1-1/8" outside diameter for connections). The dimensions of the finned area are A x B + 1" on 2 sides for metal ears/bracket. The casing bracket is made of galvanized steel. The heat exchange area consists of single rows of 1/8" tubes with 12 fins per inch. The fin material is aluminum. An Evaporator coil. Page | 14 CHAPTER 2 LITERATURE REVIEW Page | 15 D.B. Jani, Manish Mishra, P.K. Sahoo, 2015 Work done - In hot and muggy areas, solid desiccant cooling has been the subject of experimental research as a supplement to conventional vapor compression refrigeration air conditioning systems. They created a hybrid air conditioning system that uses vapor compression and solid desiccant to cool a 1.8 kW test room. During the cooling season, which runs from March to September, the system was simulated in the TRNSYS environment and modeled in a simulation studio project. Next, the outcomes were verified using test data from experiments. Outcome- The effect of temperature and the ratio of ambient humidity on the supply air temperature and the associated coefficient of performance has been evaluated. At various regeneration temperatures, the variations I n the coefficient of performance were measured. The results of the simulation show how well these technologies work to cool buildings in hot, muggy conditions. Zhao et al. (2023): Work Done: Evaluated the performance of a novel hybrid solid desiccant cooling system integrated with a heat pump and solar thermal collector in a subtropical climate. Outcome: The system achieved significant energy savings, reducing the power consumption by up to 30% compared to conventional systems. The study highlighted the critical role of solar thermal energy in enhancing the desiccant regeneration process, especially under high humidity conditions. Chen et al. (2023): Work Done: Proposed a new configuration of a hybrid desiccant cooling system using an advanced heat recovery loop and phase change materials (PCMs) to enhance the thermal storage and recovery process. Outcome: The integration of PCMs improved the system's efficiency by maintaining a stable temperature and reducing energy consumption. The experimental results showed a 15% increase in COP and enhanced system reliability. Gong et al. (2023): Page | 16 Work Done: Investigated the performance of a hybrid system combining a solid desiccant wheel with an adsorption chiller for simultaneous cooling and water production. Outcome: The system demonstrated excellent performance in both cooling and water production, achieving a cooling load of 6 kW and water production rate of 60 kg/h under optimal conditions. This hybrid approach significantly enhanced the system’s overall efficiency and sustainability. Vijay et al. (2023): Work Done: Analyzed the impact of varying operational parameters on the performance of a hybrid solid desiccant cooling system using TRNSYS simulations. Outcome: The study identified key parameters such as desiccant wheel rotation speed, airflow rate, and regeneration temperature that significantly influence system performance. Optimization of these parameters improved the COP by 20%, making the system more effective for different climatic conditions. Huang et al. (2023): Work Done: Conducted a comprehensive review and performance assessment of hybrid desiccant systems integrated with renewable energy sources. Outcome: The review confirmed that solar-assisted desiccant cooling systems not only improve energy efficiency but also enhance the overall sustainability of HVAC systems. The study emphasized the potential for these systems to significantly reduce carbon emissions and operational costs. Khan et al. (2023): Work Done: Developed a model for a hybrid desiccant cooling system integrated with an adsorption chiller for energy and water co-generation. Outcome: The model demonstrated a cooling capacity of 5 kW and a water production rate of 55 kg/h, showing enhanced performance under high ambient temperatures. The combined system showed a 10% improvement in COP and a 25% reduction in energy consumption compared to standalone systems. Smith et al. (2023): Page | 17 Work Done: Explored the economic and environmental benefits of deploying hybrid solid desiccant cooling systems in commercial buildings. Outcome: The study highlighted significant cost savings and payback periods, especially when combined with solar energy. The systems showed a reduction in operational costs by 20-30% and a decrease in greenhouse gas emissions by up to 40%. Jani, D.B., Mishra, M., & Sahoo, P.K. (2016) Work Done: Experimental investigations on a solid desiccant-vapor compression hybrid air-conditioning system under various operational conditions in hot and humid weather. Outcome: The hybrid system achieved a 20-30% reduction in electrical energy consumption compared to conventional systems. Optimal operational conditions significantly enhanced system efficiency. Luo, W.J., Faridah, D., Fasya, F.R., Chen, Y-S., & Mulki, F.H. (2019) Work Done: Developed a solar-assisted hybrid SDCS using solar-heated water for the regeneration process, coupled with a heat pump's condenser heat recovery. Outcome: Enhanced system performance by approximately 10% in terms of power consumption. Identified critical outdoor temperature range (27-30°C) for optimal COP. Fatouh, M., Abou-Ziyan, H., Mahmoud, O., & Abd El-Raheim, D. (2017) Work Done: Experimental analysis comparing hybrid and conventional air conditioning systems in hot-humid climates, focusing on energy consumption, cooling capacity, and indoor air quality. Outcome: Hybrid systems reduced energy consumption by up to 25%, providing better control over indoor humidity levels and improved thermal comfort. Page | 18 Comino, F., Gonzalez, J.C., Navas-Martos, F.J., & Adana, M.R. (2020) Work Done: Experimental energy performance assessment of a solar desiccant cooling system in Southern European climates through simulations and real-time monitoring. Outcome: High energy efficiency, especially in regions with high solar insolation, with significant reductions in energy consumption compared to traditional systems. Hwang, W-B., Choi, S., & Lee, D-Y. (2017) Work Done: Analyzed a hybrid desiccant cooling system with an electric heat pump, examining various operational parameters. Outcome: Higher COP and reduced energy consumption compared to standalone systems. Enhanced overall system efficiency and improved indoor thermal comfort levels. Sheridan, P. & Mitchell, D. (2017) Work Done: Investigated performance of hybrid desiccant cooling systems in hot- humid and hot-dry climates using simulations and experimental data. Outcome: Identified that the hybrid system performs well in high sensible and latent heat load conditions, with significant improvements in system efficiency and cooling capacity. Ge, T., Li, Y., Dai, Y.J., Wang, R.Z., & Ma, Q. (2018) Work Done: Experimental study on a solar-assisted solid desiccant-vapor compression hybrid cooling system in Taichung, Taiwan, assessing performance from spring to fall. Outcome: System performance enhanced by approximately 10% with the integration of a solar-heated water heat exchanger. Optimal performance observed at high outdoor humidity ratios and within a specific ambient temperature range (26- 30°C). Page | 19 Luo W-J, Faridah D, Fasya FR, Chen Y-S, Mulki FH, Adilah UN (2019): Work Done: Investigated the performance of a solar-assisted hybrid solid desiccant cooling system in Taiwan. Solar-heated water was used as an additional heat source for the regeneration process, supplemented by heat recovery from the condenser of an integrated heat pump. Outcome: The system's overall performance improved by approximately 10% in terms of power consumption. The system showed better performance in high outdoor humidity conditions, with the coefficient of performance (COP) increasing until a certain temperature threshold, beyond which it decreased. Liu X-H, Zhang T, Zheng Y-W, Tu R (2016): Work Done: Conducted performance investigation and exergy analysis of two-stage desiccant wheel systems. Outcome: Demonstrated significant improvements in energy efficiency and effectiveness of the hybrid system in managing humidity and providing cooling. Exergy analysis helped in identifying and minimizing losses in the system. Ali et al. (2022): Work Done: Developed a hybrid system using solid desiccant for latent load removal and an aqua ammonia absorption chiller for sensible load removal. Outcome: Improved cooling capacity by 2 kW and enhanced overall system performance by 50-55% compared to traditional desiccant systems. Comino F, Castillo González J, Navas-Martos FJ, Ruiz de Adana M (2020): Work Done: Assessed the energy performance of a solar desiccant cooling system in Southern Europe. Outcome: The system was found to be highly efficient in hot and dry climates, demonstrating substantial energy savings and effective humidity control. Henning H-M, Erpenbeck T, Hindenburg C, Santamaria IS (2001): Work Done: Explored the potential of solar energy in desiccant cooling cycles. Page | 20 Outcome: Showed that integrating solar energy could significantly enhance the performance of desiccant cooling systems, making them more sustainable and reducing reliance on conventional energy sources. Luo et al. (2019): Work Done: Developed a solar-assisted hybrid solid desiccant cooling system in Taiwan, using solar-heated water for the regeneration process and heat recovery from the condenser of an integrated heat pump. Outcome: Enhanced system performance by about 10% in power consumption. The system was more effective in high humidity conditions, with a critical outdoor temperature influencing the coefficient of performance (COP). The COP increased with temperature up to a threshold, after which it declined. Processes (2023): Work Done: Investigated a novel hybrid solid desiccant-based M-cycle cooling system combined with a humidification–dehumidification (HDH) desalination unit. Compared three operational modes: ventilation, recirculation, and half recirculation. Outcome: The recirculation mode showed superior cooling performance and higher COP, producing up to 7.91 kW of cooling load. All modes had similar water production rates, with the recirculation mode slightly outperforming others in cooling efficiency. Kashif Shahzad et al. (2022): Work Done: Conducted an experimental evaluation of a hybrid solid desiccant M- cycle indirect evaporative cooling (IEC) system. Outcome: Demonstrated significant improvements in cooling capacity and energy efficiency, achieving energy savings between 70% to 135% compared to traditional systems. The system effectively managed moisture removal, enhancing overall performance in humid climates. Page | 21 Ali et al. (2022): Work Done: Developed a hybrid system using solid desiccant for latent load removal and an aqua ammonia absorption chiller for sensible load removal. Outcome: Improved cooling capacity by 2 kW and enhanced overall system performance by 50-55% compared to traditional desiccant systems. Comino et al. (2020): Work Done: Assessed the energy performance of a solar desiccant cooling system in Southern Europe. Outcome: The system was found to be highly efficient in hot and dry climates, demonstrating substantial energy savings and effective humidity control. The current rise in the use 0f conventional energy sources such as heat engines and electricity is largely driven by CFC-based cooling and air conditioning systems for cooling purposes. This reliance on conventional power plants for electricity generation has resulted in substantial harm to our environment, contributing to global warming. The widespread use of these traditional cooling and air conditioning systems has also led to higher levels of environmental CFCs, which in turn have caused ozone depletion. Furthermore, the increasing global population has heightened the demand for energy to support a higher standard of living and the use of advanced technology. In conventional vapor compression systems, both the sensible and latent heat loads of a given space are managed by the same air- handling unit. Currently, the only controlled parameter for conditioned air in these systems is the dry-bulb temperature, with humidity control as a byproduct of temperature regulation. In humid climates, the high ambient moisture levels make it difficult to directly control humidity. As a result of this inherent limitation in system design, the conditioned air can reach the target Dry Bulb Temperature (DBT), but the relative humidity may fall outside of the comfort range. This lack of humidity control can lead to issues such as mold growth and can make it challenging to achieve optimal indoor air quality and thermal comfort using vapor compression air-conditioning systems, which consume a significant amount of electricity. In traditional systems, comfortable conditions can only be achieved when the Sensible Heat Ratio (SHR) is above 0.75. However, this Page | 22 value may be much lower in hot and humid climates, making it difficult to reach optimum comfort levels. Due to the requirement of an SHR greater than 0.75, it can be challenging to meet thermal comfort conditions as well. An additional limitation of the traditional system is the potential for condensate evaporation within the air-conditioned area caused by excessive cooling, leading to elevated humidity levels within the space. The primary component in desiccant dehumidification and cooling systems is a rotary desiccant dehumidifier. These systems can utilize low-grade thermal energy, such as solar or waste heat, to regenerate the desiccant dehumidifier. Renewable energy sources, like solar panels, can be employed to power the fans and water pump. Due to the decoupling of sensible and latent cooling loads, the system can operate efficiently across a wide range of sensible heat ratios (SHR). This makes desiccant-based air dehumidification and air conditioning systems a compelling choice for reducing air humidity and temperature, particularly when incorporating renewable energy sources. As a result, desiccant cooling systems present an energy-efficient, environmentally friendly, and cost-effective alternative to traditional climate control systems. A material that attracts moisture and creates a dry environment is known as a desiccant. Desiccants, whether natural or synthetic, have the ability to absorb or adsorb water vapor by exploiting the pressure difference between the surrounding air and the desiccant's surface. This desiccant cooling technology not only ensures optimal indoor comfort but also reduces energy consumption and supports the development of innovative materials for increased efficiency. Overview of Desiccant Cooling Classifica ons Here is a breakdown of the classifications of desiccant cooling systems. Page | 23 The desiccant cooling system offers significant advantages over traditional vapor compression systems in terms of improving indoor air quality, reducing costs and energy consumption, and promoting environmental sustainability. This system utilizes renewable energy sources such as solar energy and industrial waste heat, addressing the growing concerns of energy depletion and environmental degradation on a global scale. Furthermore, its ability to effectively remove pollutants and contaminants while being environmentally friendly positions desiccant cooling as a suitable and timely technology for enhancing indoor air quality. The current review underscores the utilization of cutting-edge desiccant materials that can be recharged at lower temperatures, close to room temperature, as a crucial factor in further enhancing the benefits of desiccant cooling. This will contribute significantly to improving comfort levels, reducing energy consumption, and lowering costs. This research provides valuable insights for new scholars seeking to understand the underlying principles of the system and offers a concise overview of the advancements in this field. Additionally, it highlights the advantages of employing hybrid desiccant cooling cycles, which not only cater to air conditioning requirements but also promote energy efficiency and environmental sustainability. The combination of solid and liquid desiccant cooling systems can achieve high efficiency by utilizing renewable solar heat or industrial waste heat for regeneration. These systems can function independently or be integrated with a VCR air conditioning system. Additionally, the hybrid desiccant cooling and dehumidification process can be connected to traditional vapor compression systems. The desiccant dehumidifier plays a critical role in reducing specific humidity by extracting moisture from the cooling coil, while the refrigerating coil in the VCR system lowers the dry bulb temperature by removing sensible heat from the air. The integration of solar energy to provide reactivation energy in a hybrid desiccant cooling system has ultimately led to a decrease in operating costs. In this system, known as a liquid desiccant cooling system, solar heat or industrial waste heat is utilized to regenerate the weak solution of liquid desiccant material, while the solid desiccant material is regenerated using thermal heat from a conventional electrical heating system. Both sensible cooling coil and supply room air temperatures are kept low during reheating, ensuring the effectiveness of both system types. The main objective of this review is to focus on the development of a liquid and solid dehydration cooling system. By comparing the operating performance parameters of liquid and solid drying systems in various environments, the most suitable system can be selected. The paper evaluated various classifications of hybrid cooling systems which utilize a combination of different cooling elements, regeneration heat sources, and optional operating modes. Specifically, the solid or liquid desiccant cooling system has been designed as an alternative to traditional vapor compression systems in order to reduce high grade electricity consumption. This system takes advantage of solar energy, particularly during peak Page | 24 solar radiation periods in the hot summer season, to enhance overall efficiency by decreasing the amount of heat needed for desiccant regeneration. Study on the Operation of a Liquid Desiccant Cooling System Liquid desiccants have the ability to remove water vapor from process air through mass transfer driven by vapor pressure differentials. Heat is generated when the water vapor is condensed by mixing with the desiccant. The dehumidified air can then be directed to the cabin for ventilation and cooling through the evaporative cooler. Simultaneously, the diluted desiccant solution can be pumped into the regenerator for reactivation. The diluted solution within the regenerator is able to flow through a liquid-liquid-sensitive heat exchanger before entering a thermal spiral that elevates the temperature to produce a hot desiccant solution. Throughout the regeneration process, moisture is extracted from the weak desiccant solution and transferred to the air. Principle of Operation for Solid Desiccant Cooling Systems A desiccant wheel, which usually consists of a gently spinning de-drying wheel, is used in the operation of a solid desiccant cooling system. This technique uses a variety of solid desiccant materials, including zeolite, molecular sieves, silica gel, calcium chloride, titanium silicates, alumina activated (both naturally and artificially), organically-based desiccants, polymers, and compounds. Page | 25 In this procedure, the solid desiccant material functions as an adsorbent in the cooling system by absorbing water vapor from the air. The thermal heater, also known as the heat regenerator, the de-drying wheel, the de-drying rack, and the thermal cooler are the main parts of a desiccant cooling system. Analysis of Solid Desiccant Cooling System Functionality Page | 26 CHAPTER 3 METHODLOGY Page | 27 EVALUATION OF COOLING LOAD The primary goals of conducting a cooling load analysis include: Ensure precise control of temperature, humidity, air purity, and pressurization in order to prevent contamination. Create a comfortable and healthy indoor environment in office buildings, educational facilities, entertainment venues, shopping centers, hotels, and other public spaces. Implement spatial air filtration systems to eliminate bacteria, maintain high indoor air quality, and prevent the spread of contaminants. EVALUATION OF COOLING LOAD The ideal indoor temperature and rela ve humidity levels for most comfort systems are as follows: The office cabin's cooling load is es mated based on indoor design condi ons of 25ºC dry bulb temperature and 50% rela ve humidity. For summer, the desired indoor condi ons are 22.78 ºC to 26.11 ºC with a rela ve humidity of 50%. In contrast, winter indoor condi ons range from 22.11 ºC to 22.22 ºC with a rela ve humidity of 20% to 30%. To determine the outdoor design condi ons, data from the local weather bureau or airport records are used. For BHOPAL city, the outdoor design condi ons for summer (May) are 39.4 ºC with a rela ve humidity of 33%, while for the monsoon season (July) they are 30.7 ºC with a rela ve humidity of 81%. Tables 3.1, 3.2, and 3.3 provide geographical, meteorological, and building structure data for the cooling load analysis. Figure 3.1 presents a 3D CAD diagram illustra ng the actual structure of the office cabin. Table 3.1: Building Loca on and Meteorological Informa on for BHOPAL City In this table, we present the building locations and meteorological data for the city of BHOPAL. Location of Department of Mechanical Engineering, at The site is situated in the Building the LNCT in Bhopal. BHOPAL district of Madhya pradesh, India, at approximately 310 meters above sea level and positioned at a longitude of 74.25°E and latitude of 22.83°N. The climate in BHOPAL is characterized by a local steppe climate, with Environmental minimal rainfall occurring throughout the year. conditions Page | 28 The Mean Yealy maximum temperature 32.09 °C The average highest temp. of the 39.04 °C. warmest month, which is May. The average maximum temp. during the chilliest Month, December 29.02 °C Mean Annual minimum temperature. 19.03 °C. Lowest mean temperature in the hotest month (May) 25.1 °C. Lowest average temperature in December 11.09 °C. The highest temp. ever recorded was on 2 47°C June 1991. The Lowest temp. ever recorded was on 15 0°C Jan 1935. The average annual precipita on is 80.1 cm. Average yearly Rainy days. 35 D The highest monthly rainfall is typically 00.03 cm. recorded in July. The lowest monthly rainfall is typically 29.05 cm recorded in February. "On August 11, 1933, the highest amount of 26.08 cm rainfall ever recorded within a 24-hour period was documented." During the southwest monsoon season, the amount of rainfall is equivalent to the annual 93.0% total. The humidity levels range from 77% During the southwest monsoon season, to 90% in the morning, and from 45% rela ve humidity values increase. to 74% in the a ernoon. Page | 29 Table 3.2: Climatological data for temp. & humidity in BHOPAL The following table presents climatological data for the temperature and humidity in BHOPAL. Month Minimum Maximum Relative Humidity (%) Temp.(°C) Temp. (°C) Jan 11.6 27.7 41.0 Feb 14.0 30.6 33.5 Mar 18.9 34.9 27.6 April 23.6 37.6 28.0 May 24.5 40.1 38.1 June 23.9 34.2 60.5 July 24.5 31.0 78.0 Aug 21.8 28.9 83.2 Sept 21.1 30.5 74.1 Oct 19.9 34.0 51.5 Nov 15.9 30.9 42.6 Dec 12.8 27.6 44.1 Yrs. 19.6 32.7 49.9 Page | 30 TabIe 3: Data on Building Structure Presented below is information on the structure of the building for reference. Components Size Remarks The building is The area measures 72 meters by 72 constructed with a meters. frame structure and consists of Ground floor and First floor levels. 230 mm common bricks are used with an air space in between before adding another The building's layer of 230 mm common bricks. On the exterior walls are inner side, there is a 15 mm sand cement comprised of plaster, while the outer side is covered with a lightweight 20mm stone create plaster. materials. The interior walls The cavity wall consists of 230mm of the building common bricks, with a 15mm inner and consist of various 20mm outer sand cement plaster layer. materials. Concrete of M25RCC has been poured to a depth of 200mm, and finished with a The rooftops are 15mm plaster. The floor is composed of made up of. kota stone tiles measuring 279.4x279.4mm and 25mm thick. Page | 31 The windows are A single glass material that is 12.7mm composed of. thick and comes with a frame panel. The office cabin is The office cabin within the building on According to the building situated in a the ground floor of the IC engine structure, solar radiation is building where the laboratory is to be air conditioned. The present during early cooling load building is located in the southeast morning hours for a calculations are position. duration of 2-3 hours. The being conducted remaining time is based on the solar considered a shadow zone. radiation. Specifications: The structure measures 2.286m x 2.286m Size of office x 2.92m and is made of prefabricated cabin with portable materials including aluminum, included materials. wood, and glass on three sides. Figure 3.1: 3D Structure of an Office Cabin for Calculating Cooling Load This figure depicts the 3D office cabin structure used in calculating the cooling load. Page | 32 Component Loading The sum of heat that needs to be eliminated from a space to reach the specified temp. (22-26 °C) The cooling load, also known as the conditioned load, is the amount of relative humidity (49%) produced by the air conditioning system. Heat sources inside and outside the body are included in this. Heat Gains Heat gains in a building can be categorized as external or internal. The movement of thermal energy from external sources into the room is the cause of external heat gains. This can happen as a result of solar radiation entering through windows and doors, ventilation, infiltration, and conduction in external walls, roofs, and the ground.. Internal heat gains, on the other hand, come from sources within the building such as people, electric equipment, and lighting. The figure above illustrates the different components of heat load. Figure 3.2: Components of the load. Heat Transfer via Transparent Surfaces The majority of sensible heat Ioad in a building is attributed to the heat gain through its structure, including walls, floors, ceiling, doors, & windows. This heat transfer is primarily caused by the temp. difference across opaque surfaces. As illustrated in Figure 3, heat from the outside air is predominantly transferred through convection to the outer surface of the structure. Subsequently, the heat is conducted through the structure to the inner surface. From there, the heat is transferred by convection to the room air. Page | 33 Figure 3.3: Analysis of Heat Transfer Across Opaque Surfaces A study on the transfer of heat through opaque surfaces is presented in this figure. The following formula can be used to calculate the rate of heat transfer from outside air to interior air: 𝑸𝑸 = 𝑼𝑼𝑼𝑼(𝑪𝑪𝑪𝑪𝑪𝑪𝑪𝑪) If we let 𝑈𝑈 represent the overall heat transfer coefficient (𝑖𝑖𝑖𝑖 𝑊𝑊/𝑚𝑚² − °𝐶𝐶), then... The abbreviation CLTD stands for the cooling load temperature difference in degrees Celsius. The variable A represents the surface area and is measured in square meters (m²). The overall heat transfer coefficient (Uo) for the outer walls of an office can be calculated when the wall, floor, or ceiling is comprised of layers of different materials. This can be done using the formula provided in Fig 3.4. By taking into account the thermal conductivity of each material layer, the overall heat transfer coefficient can be determined. This calculation is important for determining the efficiency of insulation in the building envelope and ensuring optimal thermal performance. Figure 3.4: Heat Transfer in Composite Wall with Air Space An illustration showcasing the process of heat transfer within a composite wall featuring an air space. Page | 34 1 Uexposedwall= 1 𝑥𝑥 1 𝑥𝑥 1 + 1 + + 2 −∞+ ℎ0 𝑘𝑘1 𝑘𝑘𝑎𝑎 𝑘𝑘2 ℎ𝑖𝑖 The film coefficient outside was determined as ho. The thermal conductivity values of bricks, plasters, and air gaps are represented by the symbol k. The tables below provide information on the overall heat transfer coefficient for various fenestration materials and the thermo-physical properties of common building and insulating materials according to ASHRAE standards. Check table TabIe 4 Shows the fenestra on-related overall heat transfer coefficient. Some kind of glass U-Value W/m2K No Shading Shading Panels ver cal Glass Winter me exposure outside: 6. 42 4. 88 Summer me exposure outside: 6.02 4. 59 Interior Room Divider 4. 15 Enhanced window system 3. 6 2. 66 with additional storage space (air gap of 25mm or greater). One thin plastic sheet. Exposure to outdoor elements 6. 19 – Winter: Exposure to outdoor elements 5. 68 – Summer: Page | 35 Internal Divider 3. 97 Glass sheets that are oriented horizontally. Outdoor exposure in winter 6. 93 (Increase in the upward flow of heat.) Outdoor exposure in Summer 4. 71 (Increase in the downward flow of heat) 5. 45 Increase in the Internal space divider upward flow of heat.) Heat transfer between the outside air and outer surface of a wall, as well as between the inner surface and inside air, is equal to the convec on heat transfer. Air velocity influences the air film coefficient, which in turn affects convection heat transmission. Because there is less air movement inside the room, the outside air film coefficient (ho) is higher than the inside air film coefficient (hi). For information on the surface conductance values for the air film, see Table 6. TabIe 5: Air Film Surface or Film Conductance The following table presents data on the conductance of surface or film in air. Three distinct air space widths, orientations, and mean temperatures are represented by the thermal conductance is presented in TabIe 7 Table 6: Thermal Conductance of Air Space (ka) The following table presents the thermal conductance values for air space. Page | 36 3.5 Determination of Cooling Load Temp. Difference (CLTD) The Cooling Load Temp. Difference (CLTD) is the temp. difference that takes into consideration both radiant heat and the actual temperature variance across a wall or ceiling. Based on solar radiation changes typical of the 24°N latitude on July 21, the ASHRAE established CLTD values for exterior walls and roofs. These values took into consideration specific indoor and outdoor air temperature circumstances as well as commonly used building materials in North America. It is significant to note that when the building is not in latitude 77.5°N, especially below 23°N, the accuracy of these CLTD measurements may change. When locating somewhere other than 40°N latitude, the LM correction factor is applied. Since BHOPAL is located at a latitude of 23.25°N, 23°N is used as the latitude where the closest corrected factor value is obtained. You can find the comparable CLTD values for type D walls here. Table 9 provides values for specific latitude, time of year, wall color, temperature difference between outside and inside design conditions, and variation in outside design temperature. These values are based on a mean temperature of 29.4°C, room temperature of 24.5°C, dark surface, and clear sky conditions. For CLTD values for different roof and ceiling types, reference should be made to ASHRAE handbooks. To account for different design conditions, the CLTD values in Table 3.9 must be adjusted before being used in heat transfer equations. The following equation can be utilized for correcting CLTD: 𝑪𝑪𝑪𝑪𝑪𝑪𝑪𝑪 𝒄𝒄𝒄𝒄𝒄𝒄𝒄𝒄 = (𝑪𝑪𝑪𝑪𝑪𝑪𝑪𝑪 + 𝑳𝑳𝑳𝑳 )𝑲𝑲 + (𝟐𝟐𝟐𝟐. 𝟓𝟓 − 𝑻𝑻𝑻𝑻 ) + (𝑻𝑻𝑻𝑻 − 𝟐𝟐𝟐𝟐. 𝟒𝟒) Where: To = outside design temperature (in °C) Ti = inside design temperature (in °C) LM = latitude month correction from Table 10 K = correction factor based on building color (K = 1 for dark, 0.85 for medium, and 0.65 for light) In cases where walls are thermally heavier than group A walls due to additional insulation, the appropriate corrections should be applied accordingly. Page | 37 Table 7: CLTD for heavier walls In the case where the wall is heavier, Cooling Load Temp. Difference (CLTD) values are provided in Table 9. Table 8: Latitude Month Correction (LM) Value for 23°N Please find below the LM value for the latitude of 23°N as shown in Table 10. Page | 38 3.6 Heat Gain Through Glass Solar radiation passing through glass panels leads to heat gain, as illustrated in Figure 3.5. The heat absorbed by glass surfaces significantly contributes to the cooling system's workload. This heat transfer can be a result of direct sunlight or diffuse radiation reflected from objects outdoors. The amount of heat transmitted through the glass is influenced by factors such as the wavelength of the radiation and the physical and chemical properties of the glass. When radiation hits the glass, some of it is absorbed, some is reflected, and the remainder is transmitted through the material. Heat transfer through glass occurs in two main ways: transmission heat gain and solar heat gain. Mathematical equations are utilized to estimate the heat gain from glass surfaces. Transmission Heat Gain Through Glass The phenomenon of heat gain through glass due to transmission. Q glass =UA(CLTD) corr Based on the given values, with U = 5.636W/m²-K and an area of glass of 2.211m², the Corrected Load Temperature Difference (CLTD) for a 3-hour period from 8:00 am to 11:00 am is calculated to be 1°C. 3.8 Solar Radiation Heat Gain: Q glass =A×SHGF max × SC ×CLF The maximum solar heat gain factor (SHGFmax) is measured in watts per square meter. The shading coefficient (C) varies depending on the type of shading being used. The cooling load factor (CLF) is also a factor to consider in the calculation. Page | 39 The month, direction, and latitude influence the Cooling Load Factor (CLF) and Maximum Solar Heat Gain Factor (Max SHGF). These variables include heat gain energy that is transferred indirectly as well as directly transferred energy. The CLF fluctuates according to the season and orientation. 3.9 Occupant Heat Gain In a cooled environment, the presence of human bodies contributes to the overall cooling load through the release of sensible and latent heat. In a room with air conditioning, the sensible heat emitted by occupants is a result of the temp. difference between their bodies and the room air. The amount of heat gained from occupants is determined by the estimated number of individuals expected to be in the conditioned space. The heat load generated by each person is influenced by their level of activity, with higher levels of activity leading to increased heat gains. The calculation of heat gain from occupants is based on the following equations for sensible heat gains. 𝑄𝑄𝑠𝑠, 𝑝𝑝𝑝𝑝𝑝𝑝𝑝𝑝𝑝𝑝𝑝𝑝 = 𝑞𝑞𝑠𝑠, 𝑝𝑝𝑝𝑝𝑝𝑝𝑝𝑝𝑝𝑝𝑝𝑝 𝑥𝑥 𝑁𝑁 𝑥𝑥 𝐶𝐶𝐶𝐶𝐶𝐶 The increase in latent heat caused by the presence of occupants. Q 1person = q l,person x N In the given formula: Qs represents sensible heat gain per person in Wats, Ql represents latent heat gain per person in Wats, N is the total number of individuals in the condi oned space, CLF signifies the cooling load factor. Refer to Table.3.14 for the specified heat gain rates from occupants at varying ac vity levels, considering a room temperature of 25°C. Rate of Heat Gain from Occupant at Conditioned Space TabIe 12 The table displays the rate of heat gain from occupants in the conditioned space in watts. Page | 40 The Cooling Load Factor (CLF) is a factor dependent on the occupants of a space and the time they spend there. In spaces with a high occupant density or when the cooling system is not operating continuously, it equals 1. The human body produces sensible and latent heat, with increased activity levels producing a minor rise in sense heat and a significant increase in latent heat due to increased perspiration. Due to thermal mass effects, people's whole sensible heat rate is not instantly transferred to cooling load; hence, a CLF correction is required. On the other hand, the latent heat is transformed to cooling load right away and doesn't need a CLF correction. Page | 41 3.10: Heat Gain from Lighting Equipment In this section, we will discuss the heat gain resulting from the use of lighting equipment. Electric lights in a space can generate heat equal to the amount of power consumed. The heat gained from electric lighting depends on factors such as the light's wattage, use factor, and allowance factor. A rough estimate for lighting load in an office space is 33.5 𝑊𝑊/𝑚𝑚2 to achieve a standard of 540 𝑙𝑙𝑙𝑙𝑙𝑙𝑙𝑙𝑙𝑙𝑙𝑙/𝑚𝑚2. To calculate the heat gain from electric lighting, one can use the formula 𝑸𝑸𝑸𝑸𝑸𝑸𝑸𝑸𝑸𝑸𝑸𝑸 = 𝑻𝑻𝑻𝑻𝑻𝑻𝑻𝑻𝑻𝑻 𝒘𝒘𝒘𝒘𝒘𝒘𝒘𝒘𝒘𝒘𝒘𝒘𝒘𝒘 𝒐𝒐𝒐𝒐 𝒍𝒍𝒍𝒍𝒍𝒍𝒍𝒍𝒍𝒍 𝒙𝒙 𝑼𝑼𝑼𝑼𝑼𝑼 𝒇𝒇𝒇𝒇𝒇𝒇𝒇𝒇𝒇𝒇𝒇𝒇 𝒙𝒙 𝑨𝑨𝑨𝑨𝑨𝑨𝑨𝑨𝑨𝑨𝑨𝑨𝑨𝑨𝑨𝑨𝑨𝑨 𝒇𝒇𝒇𝒇𝒇𝒇𝒇𝒇𝒇𝒇𝒇𝒇. The ratio of installed wattage to actual wattage is known as the utilization factor, varying depending on room type. It's typically unity in residential, commercial, and shop settings, and below 0.5 in industrial workshops. The allowance factor is 1.25. Table 13: Standard Lighting Load Please find below the typical lighting load for reference Description of rooms Lighting power W/m2 Office area 𝟏𝟏𝟏𝟏𝟏𝟏/𝒎𝒎𝟐𝟐 Professional Gathering Space 14 𝑾𝑾/𝒎𝒎𝟐𝟐 Educational Session/ Seminar/ 15 𝑾𝑾/𝒎𝒎𝟐𝟐 Instructional Workshop. Guest/Spectator section 10 𝑾𝑾/𝒎𝒎𝟐𝟐 Dining room 10 𝑾𝑾/𝒎𝒎𝟐𝟐 Lab 15 𝑾𝑾/𝒎𝒎𝟐𝟐 Restro area 10 𝑾𝑾/𝒎𝒎𝟐𝟐 ElectricaI & mech room 16 𝑾𝑾/𝒎𝒎𝟐𝟐 Workshop 20 𝑾𝑾/𝒎𝒎𝟐𝟐 Library & reading Section 13 𝑾𝑾/𝒎𝒎𝟐𝟐 When calculating the heat gain from electric equipment (Qequipment), the formula to use is as follows: Qequipment = 𝑻𝑻𝑻𝑻𝑻𝑻𝑻𝑻𝑻𝑻 𝒘𝒘𝒘𝒘𝒘𝒘𝒘𝒘𝒘𝒘𝒘𝒘𝒘𝒘 𝒐𝒐𝒐𝒐 𝒆𝒆𝒆𝒆𝒆𝒆𝒆𝒆𝒆𝒆𝒆𝒆𝒆𝒆𝒆𝒆𝒆𝒆 𝒙𝒙 𝑼𝑼𝑼𝑼𝑼𝑼 𝒇𝒇𝒇𝒇𝒇𝒇𝒇𝒇𝒇𝒇𝒇𝒇 𝒙𝒙 𝑪𝑪𝑪𝑪𝑪𝑪 Where CLF is equal to 1.0 if the equipment operates 24 hours a day, or if cooling is turned off at night or during weekends. Page | 42 Heat Gain from Office Equipment The heat gain rate of office equipment is available in accordance with ASHRAE standards as provided below. TabIe 14: Office Equipment Heat Gain Rate (Watts) Please see below for the heat gain rate in watts for office equipment. Appliance Continuous Average Ideal 15” Monitor/ Computer 110w 20w 17” 𝑀𝑀𝑀𝑀𝑀𝑀𝑀𝑀𝑀𝑀𝑀𝑀𝑀𝑀 125w 25w 19” 𝑀𝑀𝑀𝑀𝑀𝑀𝑀𝑀𝑀𝑀𝑀𝑀𝑀𝑀 135w 30w 𝐿𝐿𝐿𝐿𝐿𝐿𝐿𝐿𝐿𝐿 𝑝𝑝𝑝𝑝𝑝𝑝𝑝𝑝𝑝𝑝𝑝𝑝𝑝𝑝/𝐷𝐷𝐷𝐷𝐷𝐷𝐷𝐷𝐷𝐷𝐷𝐷𝐷𝐷 130w 100w 10w 𝑆𝑆𝑆𝑆𝑆𝑆𝑆𝑆𝑆𝑆 𝑜𝑜𝑜𝑜𝑜𝑜𝑜𝑜𝑜𝑜𝑜𝑜 320w 160w 70w 𝐿𝐿𝐿𝐿𝐿𝐿𝐿𝐿𝐿𝐿 𝑜𝑜𝑜𝑜𝑜𝑜𝑜𝑜𝑜𝑜𝑜𝑜 550w 275w 125w 𝐹𝐹𝐹𝐹𝐹𝐹 𝑚𝑚𝑚𝑚𝑚𝑚ℎ𝑖𝑖𝑖𝑖𝑖𝑖 30w 𝐹𝐹𝐹𝐹𝐹𝐹𝐹𝐹𝐹𝐹𝐹𝐹𝐹𝐹𝐹𝐹𝐹𝐹 𝑀𝑀𝑀𝑀𝑀𝑀ℎ𝑖𝑖𝑖𝑖𝑖𝑖 30w 15w 𝐼𝐼𝐼𝐼𝐼𝐼𝐼𝐼𝐼𝐼 𝑆𝑆𝑆𝑆𝑆𝑆𝑆𝑆𝑆𝑆𝑆𝑆𝑆𝑆 25w 15w 𝐷𝐷𝐷𝐷𝐷𝐷 𝑀𝑀𝑀𝑀𝑀𝑀𝑀𝑀𝑀𝑀𝑀𝑀 𝑃𝑃𝑃𝑃𝑃𝑃𝑃𝑃𝑃𝑃𝑃𝑃𝑃𝑃 50w 25w 𝐶𝐶𝐶𝐶𝐶𝐶𝐶𝐶𝐶𝐶𝐶𝐶 𝐷𝐷𝐷𝐷𝐷𝐷𝐷𝐷𝐷𝐷𝐷𝐷𝐷𝐷 400w 85w 20w 𝑂𝑂𝑂𝑂𝑂𝑂𝑂𝑂𝑂𝑂𝑂𝑂 𝐶𝐶𝐶𝐶𝐶𝐶𝐶𝐶𝐶𝐶𝐶𝐶 1100w 400w 300w 3.13 Infiltration-Induced Heat Gains Please rephrase it to: "3.2.13 Heat gains as a result of infiltration." 𝑉𝑉𝑉𝑉𝑉𝑉𝑉𝑉𝑉𝑉𝑉𝑉 𝑜𝑜𝑜𝑜 𝑠𝑠𝑠𝑠𝑠𝑠𝑠𝑠𝑠𝑠 𝑥𝑥𝑥𝑥𝑥𝑥 The quantity of air that has been infiltrated(Vinf) = 𝑚𝑚3 ∕ 𝑚𝑚𝑖𝑖𝑛𝑛 60 Page | 43 Please refer to Table 15 for the specified value of Ac, which represents the number of air changes per hour. Total Cooling Load Calculation for Office Room Dimensions: Length: 2.286 m (7.50 ft) Width: 2.286 m (7.50 ft) Height: 2.29 m (7.51 ft) Room Area: Area = 2.286 * 2.29 W D1 N 2.29m S 2.28m E SUN-Facing wall Office Size Specifica ons Office size: 5.225m² (56.24 ²) Page | 44 Area of glass (W1): "Width x Height"1.21x1.828m² (3.969x5.997 ²) = 2.211m² (23.79 ²) Area of the door (D1): "Width x Height" 1.066x2.29m² (3.96x7.51 ²) = 2.441m² (26.274 ²) Total outside wall area (SE): 𝑻𝑻𝑻𝑻𝑻𝑻𝑻𝑻𝑻𝑻 𝒔𝒔𝒔𝒔𝒔𝒔𝒔𝒔𝒔𝒔𝒔𝒔𝒔𝒔 𝒂𝒂𝒂𝒂𝒂𝒂𝒂𝒂 𝑖𝑖𝑖𝑖 𝑐𝑐𝑐𝑐𝑐𝑐𝑐𝑐𝑐𝑐𝑐𝑐𝑐𝑐𝑐𝑐𝑐𝑐𝑐𝑐 𝑎𝑎𝑎𝑎 𝑡𝑡ℎ𝑒𝑒 𝑠𝑠𝑠𝑠𝑠𝑠 𝑜𝑜𝑜𝑜 𝑡𝑡ℎ𝑒𝑒 wall area 𝑎𝑎𝑎𝑎𝑎𝑎 𝑡𝑡ℎ𝑒𝑒 window area. 4.653m² (50.084ft²) (Area of wall + Area of window) 𝑷𝑷𝑷𝑷𝑷𝑷𝑷𝑷𝑷𝑷𝑷𝑷𝑷𝑷𝑷𝑷𝑷𝑷 𝑾𝑾𝑾𝑾𝑾𝑾𝑾𝑾 𝑨𝑨𝑨𝑨𝑨𝑨𝑨𝑨𝑨𝑨 (𝑵𝑵𝑵𝑵, 𝑺𝑺𝑺𝑺, 𝑵𝑵𝑵𝑵) = = 𝐴𝐴𝐴𝐴𝐴𝐴𝐴𝐴 𝑜𝑜𝑜𝑜 𝑤𝑤𝑤𝑤𝑤𝑤𝑤𝑤 (𝑁𝑁𝑁𝑁) + 𝐴𝐴𝐴𝐴𝐴𝐴𝐴𝐴 𝑜𝑜𝑜𝑜 𝑤𝑤𝑤𝑤𝑤𝑤𝑤𝑤(𝑆𝑆𝑆𝑆) + 𝐴𝐴𝐴𝐴𝐴𝐴𝐴𝐴 𝑜𝑜𝑜𝑜 𝑤𝑤𝑤𝑤𝑤𝑤𝑤𝑤 (𝑁𝑁𝑁𝑁) Total area: 13.261m² (132.740 ²) Sun Facing Wall Roof area: 5.225m² (56.24 ²) Calculation of Total Room Sensible Heat Gain The calculation for total room sensible heat gain involves the sum of various factors within a conditioned space, including: 𝑆𝑆𝑆𝑆𝑆𝑆𝑆𝑆𝑆𝑆𝑆𝑆𝑆𝑆𝑆𝑆 ℎ𝑒𝑒𝑒𝑒𝑒𝑒 𝑔𝑔𝑔𝑔𝑔𝑔𝑔𝑔 𝑡𝑡ℎ𝑟𝑟𝑟𝑟𝑟𝑟𝑟𝑟ℎ 𝑤𝑤𝑤𝑤𝑤𝑤𝑤𝑤𝑤𝑤, 𝑓𝑓𝑓𝑓𝑓𝑓𝑓𝑓𝑓𝑓𝑓𝑓, 𝑎𝑎𝑎𝑎𝑎𝑎 𝑐𝑐𝑐𝑐𝑐𝑐𝑐𝑐𝑐𝑐𝑐𝑐𝑔𝑔𝑔𝑔 𝑆𝑆𝑆𝑆𝑆𝑆𝑆𝑆𝑆𝑆𝑆𝑆𝑆𝑆𝑆𝑆 ℎ𝑒𝑒𝑒𝑒𝑒𝑒 𝑔𝑔𝑔𝑔𝑔𝑔𝑔𝑔 𝑡𝑡ℎ𝑟𝑟𝑟𝑟𝑟𝑟𝑟𝑟ℎ 𝑤𝑤𝑤𝑤𝑤𝑤𝑤𝑤𝑤𝑤𝑤𝑤𝑤𝑤 𝑆𝑆𝑆𝑆𝑆𝑆𝑆𝑆𝑆𝑆𝑆𝑆𝑆𝑆𝑆𝑆 ℎ𝑒𝑒𝑒𝑒𝑒𝑒 𝑔𝑔𝑔𝑔𝑔𝑔𝑔𝑔 𝑓𝑓𝑓𝑓𝑓𝑓𝑓𝑓 𝑜𝑜𝑜𝑜𝑜𝑜𝑜𝑜𝑜𝑜𝑜𝑜𝑜𝑜𝑜𝑜𝑜𝑜 𝑆𝑆𝑆𝑆𝑆𝑆𝑆𝑆𝑆𝑆𝑆𝑆𝑆𝑆𝑆𝑆 ℎ𝑒𝑒𝑒𝑒𝑒𝑒 𝑔𝑔𝑔𝑔𝑔𝑔𝑔𝑔 𝑓𝑓𝑓𝑓𝑓𝑓𝑓𝑓 𝑖𝑖𝑖𝑖𝑖𝑖𝑖𝑖𝑖𝑖𝑖𝑖𝑖𝑖𝑖𝑖𝑖𝑖𝑖𝑖𝑖𝑖𝑖𝑖 𝑎𝑎𝑎𝑎𝑎𝑎 𝑆𝑆𝑆𝑆𝑆𝑆𝑆𝑆𝑆𝑆𝑆𝑆𝑆𝑆𝑆𝑆 ℎ𝑒𝑒𝑒𝑒𝑒𝑒 𝑔𝑔𝑔𝑔𝑔𝑔𝑔𝑔 𝑓𝑓𝑓𝑓𝑓𝑓𝑓𝑓 𝑣𝑣𝑣𝑣𝑣𝑣𝑣𝑣𝑖𝑖𝑙𝑙𝑙𝑙𝑙𝑙𝑙𝑙𝑙𝑙𝑙𝑙 𝑆𝑆𝑆𝑆𝑆𝑆𝑆𝑆𝑆𝑆𝑆𝑆𝑆𝑆𝑆𝑆 ℎ𝑒𝑒𝑒𝑒𝑒𝑒 𝑔𝑔𝑔𝑔𝑔𝑔𝑔𝑔 𝑓𝑓𝑓𝑓𝑓𝑓𝑓𝑓 𝑙𝑙𝑙𝑙𝑙𝑙ℎ𝑡𝑡𝑡𝑡𝑡𝑡𝑡𝑡 𝑎𝑎𝑎𝑎𝑎𝑎 𝑓𝑓𝑓𝑓𝑓𝑓𝑓𝑓 The formula for calcula ng total room sensible heat gain is as follows: 𝑅𝑅𝑅𝑅𝑅𝑅𝑅𝑅 = 𝑆𝑆𝑆𝑆𝑆𝑆𝑆𝑆𝑆𝑆𝑆𝑆𝑆𝑆𝑆𝑆 ℎ𝑒𝑒𝑒𝑒𝑒𝑒 𝑔𝑔𝑔𝑔𝑔𝑔𝑔𝑔 𝑡𝑡ℎ𝑟𝑟𝑟𝑟𝑟𝑟𝑟𝑟ℎ 𝑤𝑤𝑤𝑤𝑤𝑤𝑤𝑤𝑤𝑤, 𝑓𝑓𝑓𝑓𝑓𝑓𝑓𝑓𝑓𝑓𝑓𝑓, 𝑎𝑎𝑎𝑎𝑎𝑎 𝑐𝑐𝑒𝑒𝑖𝑖𝑖𝑖𝑖𝑖𝑖𝑖𝑖𝑖𝑖𝑖 + 𝑆𝑆𝑆𝑆𝑆𝑆𝑆𝑆𝑆𝑆𝑆𝑆𝑆𝑆𝑆𝑆 ℎ𝑒𝑒𝑒𝑒𝑒𝑒 𝑔𝑔𝑔𝑔𝑔𝑔𝑔𝑔 𝑡𝑡ℎ𝑟𝑟𝑟𝑟𝑟𝑟𝑟𝑟ℎ 𝑤𝑤𝑤𝑤𝑤𝑤𝑤𝑤𝑤𝑤𝑤𝑤𝑤𝑤 + 𝑆𝑆𝑆𝑆𝑆𝑆𝑆𝑆𝑆𝑆𝑆𝑆𝑆𝑆𝑆𝑆 ℎ𝑒𝑒𝑒𝑒𝑒𝑒 𝑔𝑔𝑔𝑔𝑔𝑔𝑔𝑔 𝑓𝑓𝑓𝑓𝑓𝑓𝑓𝑓 𝑜𝑜𝑜𝑜𝑜𝑜𝑜𝑜𝑜𝑜𝑜𝑜𝑜𝑜𝑜𝑜𝑜𝑜 + 𝑆𝑆𝑆𝑆𝑆𝑆𝑆𝑆𝑆𝑆𝑆𝑆𝑆𝑆𝑆𝑆 ℎ𝑒𝑒𝑒𝑒𝑒𝑒 𝑔𝑔𝑔𝑔𝑔𝑔𝑔𝑔 𝑓𝑓𝑓𝑓𝑓𝑓𝑓𝑓 𝑖𝑖𝑖𝑖𝑖𝑖𝑖𝑖𝑖𝑖𝑖𝑖𝑖𝑖𝑖𝑖𝑖𝑖𝑖𝑖𝑖𝑖𝑖𝑖 𝑎𝑎𝑎𝑎𝑎𝑎 + 𝑆𝑆𝑆𝑆𝑆𝑆𝑆𝑆𝑆𝑆𝑆𝑆𝑆𝑆𝑆𝑆 ℎ𝑒𝑒𝑒𝑒𝑒𝑒 𝑔𝑔𝑔𝑔𝑔𝑔𝑔𝑔 𝑓𝑓𝑟𝑟𝑟𝑟𝑟𝑟 𝑣𝑣𝑣𝑣𝑣𝑣𝑣𝑣𝑣𝑣𝑣𝑣𝑣𝑣𝑣𝑣𝑣𝑣𝑣𝑣𝑣𝑣 + 𝑆𝑆𝑆𝑆𝑆𝑆𝑆𝑆𝑆𝑆𝑆𝑆𝑆𝑆𝑆𝑆 ℎ𝑒𝑒𝑒𝑒𝑒𝑒 𝑔𝑔𝑔𝑔𝑔𝑔𝑔𝑔 𝑓𝑓𝑓𝑓𝑓𝑓𝑓𝑓 𝑙𝑙𝑙𝑙𝑙𝑙ℎ𝑡𝑡𝑡𝑡𝑡𝑡𝑡𝑡 𝑎𝑎𝑎𝑎𝑎𝑎 𝑓𝑓𝑓𝑓𝑓𝑓𝑓𝑓. Heat Gain Through Wall The heat gain through an exposed wall can be calculated using the formula: Qexposed wall (SE) = UA(CLTD)corrected Subs tu ng the values, we get: Qexposed wall (SE) = 1.069 x 4.653 x 22.5 = 111.91W Heat Gain Through Glasses of Par on Wall During summer and monsoon, the heat gain through the glasses of the par on wall can be significant. Considering an average annual temperature of 33ºC for BHOPAL city, it is crucial to take into account these factors when calcula ng the total heat gain in a building. Page | 45 Heat gain through glass par ons from the southwest (SW) direc on can be calculated using the formula: Qglass (SW) = Uglass sheet x Aglass par on x(To-Ti), where Uglass sheet is 3.97, Aglass par on is 1.068, To is 33°C, and Ti is 25°C. Subs tu ng the values, we get Qglass (SW) = 3.97 x 1.068 x (33-25) = 33.91 W. Similarly, heat gain through glass par ons from the northeast (NE) direc on is calculated using the same formula, resul ng in Qglass (NE) = 33.91 W. For glass par ons in the northwest (NW) direc on, accoun ng for the door area, the heat gain can be calculated by the formula: Qglass (NW) = Uglass sheet x Aglass par on x(To-Ti), yielding Qglass (NW) = 33.91 W. The total heat gain through glass par ons on the par on walls can be calculated by adding the heat gains from SW, NE, and NW direc ons: Qglass par on = Qglass (SW) + Qglass (NE) + Qglass (NW) = 33.91 + 33.91 + 33.91 = 101.73 W. The heat gain through the wood section of the partition walls can be calculated by using the formula 𝑸𝑸𝑸𝑸𝑸𝑸𝑸𝑸𝑸𝑸𝑸𝑸 = 𝑼𝑼𝑼𝑼𝑼𝑼𝑼𝑼𝑼𝑼 𝒔𝒔𝒔𝒔𝒔𝒔𝒔𝒔𝒔𝒔 𝒙𝒙 𝑨𝑨𝑨𝑨𝑨𝑨𝑨𝑨𝑨𝑨 𝒑𝒑𝒑𝒑𝒑𝒑𝒑𝒑𝒑𝒑𝒑𝒑𝒑𝒑𝒑𝒑𝒑𝒑 𝒙𝒙(𝑻𝑻𝑻𝑻 − 𝑻𝑻𝑻𝑻), where Uwood sheet is the overall heat transfer coefficient for the wood, Awood partition is the surface area of the wood section, To is the outside temperature, and Ti is the inside temperature. For the heat gain through the wood section of the partition wall facing the southwest (SW) direction, the calculation is as follows: Qwoods (SW) = 1.037 x 1.068 x (33-25) = 8.860 W Similarly, for the heat gain through the wood section of the partition wall facing the northeast (NE) direction: Qwoods (NE) = 1.037 x 1.068 x (33-25) = 8.860 W And for the heat gain through the wood section of the partition wall facing the northwest (NW) direction: Qwoods (NW) = 1.037 x 1.068 x (33-25) = 8.860 W To determine the total heat gain through the wood sheet of the partition walls, we add up the heat gains from each direction: Qwood partition = Qwoods (SW) + Qwoods (NE) + Qwoods (NW) = 8.860 + 8.860 + 8.860 = 26.58 W. Calculation of Heat Gain Through Aluminium Section of Partition Wall The heat gain through the aluminium section of the partition wall can be calculated by adding the heat gain from the various sections of the aluminium material. This can be expressed as: QAluminium section = QAluminium section (SW) + QAluminium section (NE) + QAluminium section (NW) By substituting the values: QAluminium section = 3 x (UAluminium section x AAluminium section x (To – Ti)) QAluminium section = 3 x (1.13 x (4 x (0.08 x 2.30)) x (33 - 25)) QAluminium section = 3 x (1.13 x 0.736 x 8) QAluminium section = 19.96 W Page | 46 Therefore, the total heat gain through the wall can be determined by adding the heat gains from the various partition walls: 𝑇𝑇𝑇𝑇𝑇𝑇𝑇𝑇𝑇𝑇 𝐻𝐻𝐻𝐻𝐻𝐻𝐻𝐻 𝑔𝑔𝑔𝑔𝑔𝑔𝑔𝑔 𝑡𝑡ℎ𝑟𝑟𝑟𝑟𝑟𝑟𝑟𝑟ℎ 𝑤𝑤𝑤𝑤𝑤𝑤𝑤𝑤 = 𝐻𝐻𝐻𝐻𝐻𝐻𝐻𝐻 𝑔𝑔𝑔𝑔𝑔𝑔𝑔𝑔 𝑡𝑡ℎ𝑟𝑟𝑟𝑟𝑟𝑟𝑟𝑟ℎ 𝑤𝑤𝑤𝑤𝑤𝑤𝑤𝑤 (𝑆𝑆𝑆𝑆) + 𝐻𝐻𝐻𝐻𝐻𝐻𝐻𝐻 𝑔𝑔𝑔𝑔𝑔𝑔𝑔𝑔 𝑡𝑡ℎ𝑟𝑟𝑟𝑟𝑟𝑟𝑟𝑟ℎ 𝑝𝑝𝑝𝑝𝑝𝑝𝑝𝑝𝑝𝑝𝑝𝑝𝑝𝑝𝑝𝑝𝑝𝑝 𝑤𝑤𝑤𝑤𝑤𝑤𝑤𝑤 (𝑆𝑆𝑆𝑆) + 𝐻𝐻𝐻𝐻𝐻𝐻𝐻𝐻 𝑔𝑔𝑔𝑔𝑔𝑔𝑔𝑔 𝑡𝑡ℎ𝑟𝑟𝑟𝑟𝑟𝑟𝑟𝑟ℎ 𝑝𝑝𝑝𝑝𝑝𝑝𝑝𝑝𝑝𝑝𝑝𝑝𝑝𝑝𝑝𝑝𝑝𝑝 𝑤𝑤𝑤𝑤𝑤𝑤𝑤𝑤 (𝑁𝑁𝑁𝑁) + 𝐻𝐻𝐻𝐻𝐻𝐻𝐻𝐻 𝑔𝑔𝑔𝑔𝑔𝑔𝑔𝑔 𝑡𝑡ℎ𝑟𝑟𝑟𝑟𝑟𝑟𝑟𝑟ℎ 𝑝𝑝𝑝𝑝𝑝𝑝𝑝𝑝𝑝𝑝𝑝𝑝𝑝𝑝𝑝𝑝𝑝𝑝 𝑤𝑤𝑤𝑤𝑤𝑤𝑤𝑤 (𝑁𝑁𝑁𝑁) = 111.91 + 101.73 + 26.58 + 19.96 = 𝟐𝟐𝟐𝟐𝟐𝟐. 𝟏𝟏𝟏𝟏 𝑾𝑾 In conclusion, the total heat gain through the wall is calculated to be 260.16W. 𝒃𝒃) 𝑇𝑇ℎ𝑒𝑒 ℎ𝑒𝑒𝑒𝑒𝑒𝑒 𝑔𝑔𝑔𝑔𝑔𝑔𝑔𝑔𝑔𝑔𝑔𝑔 𝑡𝑡ℎ𝑟𝑟𝑟𝑟𝑟𝑟𝑟𝑟ℎ 𝑡𝑡ℎ𝑒𝑒 𝑓𝑓𝑓𝑓𝑓𝑓𝑓𝑓𝑓𝑓 𝑐𝑐𝑐𝑐𝑐𝑐 𝑏𝑏𝑏𝑏 𝑐𝑐𝑐𝑐𝑐𝑐𝑐𝑐𝑐𝑐𝑐𝑐𝑐𝑐𝑐𝑐𝑐𝑐𝑐𝑐 𝑢𝑢𝑢𝑢𝑢𝑢𝑢𝑢𝑢𝑢 𝑡𝑡ℎ𝑒𝑒 𝑒𝑒𝑒𝑒𝑒𝑒𝑒𝑒𝑒𝑒𝑒𝑒𝑒𝑒𝑒𝑒 𝑸𝑸𝑸𝑸𝑸𝑸𝑸𝑸𝑸𝑸𝑸𝑸 = 𝑼𝑼𝑼𝑼𝑼𝑼𝑼𝑼𝑼𝑼𝑼𝑼 𝒙𝒙 𝑨𝑨𝑨𝑨𝑨𝑨𝑨𝑨𝑨𝑨𝑨𝑨 𝒙𝒙 (𝑻𝑻𝑻𝑻 − 𝑻𝑻𝑻𝑻), 𝑤𝑤ℎ𝑒𝑒𝑒𝑒𝑒𝑒 𝑈𝑈𝑈𝑈𝑈𝑈𝑈𝑈𝑈𝑈𝑈𝑈 𝑖𝑖𝑖𝑖 𝑡𝑡ℎ𝑒𝑒 𝑜𝑜𝑜𝑜𝑜𝑜𝑜𝑜𝑜𝑜𝑜𝑜𝑜𝑜 ℎ𝑒𝑒𝑒𝑒𝑒𝑒 𝑡𝑡𝑟𝑟𝑟𝑟𝑟𝑟𝑟𝑟𝑟𝑟𝑟𝑟𝑟𝑟 𝑐𝑐𝑐𝑐𝑐𝑐𝑐𝑐𝑐𝑐𝑐𝑐𝑐𝑐𝑐𝑐𝑐𝑐𝑐𝑐𝑐𝑐 𝑜𝑜𝑜𝑜 𝑡𝑡ℎ𝑒𝑒 𝑓𝑓𝑓𝑓𝑓𝑓𝑓𝑓𝑓𝑓, 𝐴𝐴𝐴𝐴𝐴𝐴𝐴𝐴𝐴𝐴𝐴𝐴 𝑖𝑖𝑖𝑖 𝑡𝑡ℎ𝑒𝑒 𝑎𝑎𝑎𝑎𝑎𝑎𝑎𝑎 𝑜𝑜𝑜𝑜 𝑡𝑡ℎ𝑒𝑒 𝑓𝑓𝑓𝑓𝑓𝑓𝑓𝑓𝑓𝑓, 𝑇𝑇𝑇𝑇 𝑖𝑖𝑖𝑖 𝑡𝑡ℎ𝑒𝑒 𝑜𝑜𝑜𝑜𝑜𝑜𝑜𝑜𝑜𝑜𝑜𝑜𝑜𝑜 𝑡𝑡𝑡𝑡𝑡𝑡𝑡𝑡. , 𝑎𝑎𝑎𝑎𝑎𝑎 𝑇𝑇𝑇𝑇 𝑖𝑖𝑖𝑖 𝑡𝑡ℎ𝑒𝑒 𝑖𝑖𝑖𝑖𝑖𝑖𝑖𝑖𝑖𝑖𝑖𝑖 𝑠𝑠𝑠𝑠𝑠𝑠𝑠𝑠𝑠𝑠𝑠𝑠𝑠𝑠 𝑡𝑡𝑡𝑡𝑡𝑡𝑡𝑡. 𝐹𝐹𝐹𝐹𝐹𝐹 𝑒𝑒𝑒𝑒𝑒𝑒𝑒𝑒𝑒𝑒𝑒𝑒𝑒𝑒, 𝑤𝑤𝑤𝑤𝑤𝑤ℎ 𝑼𝑼𝒇𝒇𝒇𝒇𝒇𝒇𝒇𝒇𝒇𝒇 = 𝟒𝟒. 𝟓𝟓 𝑾𝑾/𝒎𝒎𝒎𝒎 − 𝑲𝑲, 𝑨𝑨𝑨𝑨𝑨𝑨𝑨𝑨𝑨𝑨𝑨𝑨 = 𝟓𝟓. 𝟐𝟐𝟐𝟐 𝒎𝒎𝒎𝒎, 𝑻𝑻𝑻𝑻 = 𝟑𝟑𝟑𝟑°𝑪𝑪, 𝒂𝒂𝒂𝒂𝒂𝒂 𝑻𝑻𝑻𝑻 = 𝟐𝟐𝟐𝟐°𝑪𝑪, 𝑡𝑡ℎ𝑒𝑒 𝑐𝑐𝑐𝑐𝑐𝑐𝑐𝑐𝑐𝑐𝑐𝑐𝑐𝑐𝑐𝑐𝑐𝑐𝑐𝑐𝑐𝑐 𝑤𝑤𝑤𝑤𝑤𝑤𝑤𝑤𝑤𝑤 𝑏𝑏𝑏𝑏: 𝑸𝑸𝑸𝑸𝑸𝑸𝑸𝑸𝑸𝑸𝑸𝑸 = 𝟒𝟒. 𝟓𝟓 𝒙𝒙 𝟓𝟓. 𝟐𝟐𝟐𝟐 𝒙𝒙 (𝟑𝟑𝟑𝟑 − 𝟐𝟐𝟐𝟐) 𝑸𝑸𝑸𝑸𝑸𝑸𝑸𝑸𝑸𝑸𝑸𝑸 = 𝟏𝟏𝟏𝟏𝟏𝟏. 𝟗𝟗𝟗𝟗 𝑾𝑾 c) The heat gained through the roof can be determined using the equation Qroof = Uroof x CLTDcorr, where Uroof is the overall heat transfer coefficient of the roof and CLTDcorr is the correct cooling load temperature difference. For instance, with Uroof = 2.10 W/m2-K and CLTDcorr = 37.15°C, the calculation would be: Qroof = 2.10 x 37.15 Qroof = 78.018 W d) To calculate the heat gain through the glasses of windows, the formula Qglass = UA(CLTD)corrected can be used, 𝑤𝑤ℎ𝑒𝑒𝑒𝑒𝑒𝑒 𝑈𝑈 𝑖𝑖𝑖𝑖 𝑡𝑡ℎ𝑒𝑒 𝑜𝑜𝑜𝑜𝑜𝑜𝑜𝑜𝑜𝑜𝑜𝑜𝑜𝑜 ℎ𝑒𝑒𝑒𝑒𝑒𝑒 𝑡𝑡𝑡𝑡𝑡𝑡𝑡𝑡𝑡𝑡𝑡𝑡𝑡𝑡𝑡𝑡 𝑐𝑐𝑐𝑐𝑐𝑐𝑐𝑐𝑐𝑐𝑖𝑖𝑖𝑖𝑖𝑖𝑖𝑖𝑖𝑖𝑖𝑖, 𝐴𝐴 𝑖𝑖𝑖𝑖 𝑡𝑡ℎ𝑒𝑒 𝑎𝑎𝑎𝑎𝑎𝑎𝑎𝑎 𝑜𝑜𝑜𝑜 𝑡𝑡ℎ𝑒𝑒 𝑔𝑔𝑔𝑔𝑔𝑔𝑔𝑔𝑔𝑔, 𝑎𝑎𝑎𝑎𝑎𝑎 𝐶𝐶𝐶𝐶𝐶𝐶𝐶𝐶 𝑖𝑖𝑖𝑖 𝑡𝑡ℎ𝑒𝑒 𝑐𝑐𝑐𝑐𝑐𝑐𝑐𝑐𝑐𝑐𝑐𝑐𝑐𝑐 𝑐𝑐𝑐𝑐𝑐𝑐𝑐𝑐𝑐𝑐𝑐𝑐𝑐𝑐 𝑙𝑙𝑙𝑙𝑙𝑙𝑙𝑙 𝑡𝑡𝑡𝑡𝑡𝑡𝑡𝑡𝑡𝑡𝑡𝑡𝑡𝑡𝑡𝑡𝑡𝑡𝑡𝑡𝑡𝑡 𝑑𝑑𝑑𝑑𝑑𝑑𝑑𝑑𝑑𝑑𝑑𝑑𝑑𝑑𝑑𝑑𝑑𝑑𝑑𝑑. For example, with U = 5.636 W/m2-K, Area of Glass = 2.211m2, and CLTDcorr = 1°C, the calculation would be: Qglass = 5.636 x 2.211 x 1 Qglass = 12.46 W e) The heat gain by solar radiation can be determined using the formula Qglass = A x SHGFmax x SC x CLF, where A is the area, SHGFmax is the maximum solar heat gain factor, SC is the shading coefficient, and CLF is the cooling load factor. For instance, with A = 2.211 m2, SHGFmax = 729 W/m2, SC = 0.14, and CLF = 0.87, the calculation would be: Qglass = 2.211 x 729 x 0.14 x 0.87 Page | 47 Qglass = 196.31 W Please note that the shading coefficient depends on the type of shading, and CLF is the cooling load factor. F)𝑆𝑆𝑆𝑆𝑆𝑆𝑆𝑆𝑆𝑆𝑆𝑆𝑆𝑆𝑆𝑆 ℎ𝑒𝑒𝑒𝑒𝑒𝑒 𝑔𝑔𝑔𝑔𝑔𝑔𝑔𝑔 𝑓𝑓𝑓𝑓𝑓𝑓𝑓𝑓 𝑜𝑜𝑜𝑜𝑜𝑜𝑜𝑜𝑜𝑜𝑜𝑜𝑜𝑜𝑜𝑜𝑜𝑜: o 𝐹𝐹𝐹𝐹𝐹𝐹𝐹𝐹𝐹𝐹𝐹𝐹𝐹𝐹: 𝑸𝑸𝑸𝑸, 𝒑𝒑𝒑𝒑𝒑𝒑𝒑𝒑𝒑𝒑𝒑𝒑 = 𝒒𝒒𝒒𝒒, 𝒑𝒑𝒑𝒑𝒑𝒑𝒑𝒑𝒑𝒑𝒑𝒑 𝒙𝒙 𝑵𝑵 𝒙𝒙 𝑪𝑪𝑪𝑪𝑪𝑪 o 𝐶𝐶𝐶𝐶𝐶𝐶𝐶𝐶𝐶𝐶𝐶𝐶𝐶𝐶𝐶𝐶𝐶𝐶𝐶𝐶𝐶𝐶: 𝑸𝑸𝑸𝑸, 𝒑𝒑𝒑𝒑𝒑𝒑𝒑𝒑𝒑𝒑𝒑𝒑 = 𝟕𝟕𝟕𝟕 𝒙𝒙 𝟑𝟑 𝒙𝒙 𝟏𝟏 o 𝑅𝑅𝑅𝑅𝑅𝑅𝑅𝑅𝑅𝑅𝑅𝑅: 𝑸𝑸𝑸𝑸, 𝒑𝒑𝒑𝒑𝒑𝒑𝒑𝒑𝒑𝒑𝒑𝒑 = 𝟐𝟐𝟐𝟐𝟐𝟐 𝑾𝑾 g)𝐿𝐿𝐿𝐿𝐿𝐿𝐿𝐿𝐿𝐿𝐿𝐿 ℎ𝑒𝑒𝑒𝑒?