Hema-Midterm PDF
Document Details
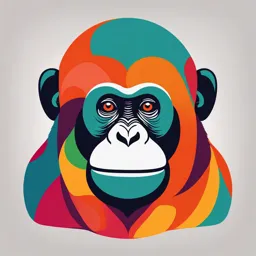
Uploaded by PrincipledMoldavite9056
MCU
Tags
Summary
This document provides an overview of red blood cell (RBC) variations and functions, including size, shape, hemoglobin content, number, and inclusions. It also describes erythrocyte sedimentation rate (ESR) methods and the clinical significance of these variations.
Full Transcript
RBCs 1. Variations in Size (Anisocytosis) Anisocytosis refers to the variation in RBC size. The two key terms associated with RBC size are: Microcytosis: RBCs are smaller than normal. Seen in iron deficiency anemia, thalassemia, or chronic disease. Macrocytosis: RBCs are larg...
RBCs 1. Variations in Size (Anisocytosis) Anisocytosis refers to the variation in RBC size. The two key terms associated with RBC size are: Microcytosis: RBCs are smaller than normal. Seen in iron deficiency anemia, thalassemia, or chronic disease. Macrocytosis: RBCs are larger than normal. Seen in vitamin B12 or folate deficiency, liver disease, and alcohol abuse. 2. Variations in Shape (Poikilocytosis) Poikilocytosis refers to abnormal shapes of RBCs, which occur in various diseases: Spherocytes: Spherical-shaped RBCs with no central pallor. Seen in hereditary spherocytosis and autoimmune hemolytic anemia. Elliptocytes (Ovalocytes): Oval or elongated RBCs. Seen in hereditary elliptocytosis or some forms of anemia. Target Cells (Codocytes): RBCs with a bull's-eye appearance. Seen in liver disease, thalassemia, and post-splenectomy. Sickle Cells: Crescent-shaped RBCs. Seen in sickle cell disease. Schistocytes: Fragmented RBCs. Seen in microangiopathic hemolytic anemia, disseminated intravascular coagulation (DIC), and mechanical heart valves. Teardrop Cells (Dacrocytes): Teardrop-shaped RBCs. Seen in myelofibrosis or severe anemia. Burr Cells (Echinocytes): RBCs with evenly spaced, small projections. Seen in uremia and liver disease. Acanthocytes: RBCs with irregular spiky projections. Seen in liver disease, neuroacanthocytosis, and abetalipoproteinemia. 3. Variations in Hemoglobin Content (Color) Variations in color, or anisochromia, refer to differences in the amount of hemoglobin inside the RBCs: Hypochromia: RBCs have less hemoglobin, making them pale. Seen in iron deficiency anemia and thalassemia. Hyperchromia: RBCs are abnormally dark due to increased hemoglobin content. Rare but may be seen in hereditary spherocytosis. Polychromasia: RBCs appear blue or grayish. Seen in reticulocytosis, a sign of the body producing immature RBCs, usually in response to bleeding or hemolysis. 4. Variations in Number Polycythemia: Increased number of RBCs. Seen in conditions like polycythemia vera or secondary to chronic hypoxia (e.g., lung disease, high altitude). Anemia: Decreased number of RBCs. Seen in various conditions such as iron deficiency, chronic disease, bone marrow disorders, etc. 5. Inclusions Inside RBCs Sometimes, abnormal structures are seen inside RBCs, indicating a pathological process: Howell-Jolly Bodies: DNA remnants inside RBCs. Seen after splenectomy or in certain anemias. Heinz Bodies: Denatured hemoglobin seen in G6PD deficiency and oxidative stress. Basophilic Stippling: Aggregates of ribosomal RNA. Seen in lead poisoning and thalassemia. Pappenheimer Bodies: Iron granules seen in sideroblastic anemia. 6. Rouleaux Formation RBCs form stacks like coins. This is seen in conditions with increased plasma proteins, like multiple myeloma or inflammatory states. 7. Autoagglutination RBCs clump together, typically seen in autoimmune hemolytic anemia. Clinical Importance These variations provide vital clues to underlying diseases. For example: Microcytic hypochromic anemia (small, pale RBCs) points to iron deficiency or thalassemia. Macrocytic anemia (large RBCs) can be a sign of vitamin B12 or folate deficiency. Poikilocytosis (abnormal shapes) can indicate various inherited disorders or marrow stress. Laboratory tests like the mean corpuscular volume (MCV) and a peripheral blood smear help detect and analyze these RBC variations for diagnosis. Erythrocyte Sedimentation Rate (ESR) is a test that measures the rate at which red blood cells (RBCs) settle at the bottom of a test tube over a specified period, typically one hour. ESR is used as a nonspecific marker of inflammation. Various methods are available to measure ESR, with the two most commonly used being the Westergren method and the Wintrobe method. Here are the details of the different ESR methods: 1. Westergren Method This is the most widely used and standardized method for measuring ESR, recommended by the International Council for Standardization in Haematology (ICSH). Procedure: o Blood is drawn into a tube containing an anticoagulant, typically sodium citrate. o The blood-anticoagulant mixture is placed in a vertical Westergren tube (200 mm long and 2.55 mm in diameter). o After 1 hour, the distance that RBCs have settled from the top of the blood column is measured in millimeters. Normal ESR Values (Westergren method): o Men: 0-15 mm/hour o Women: 0-20 mm/hour Advantages: High sensitivity to inflammatory changes. Disadvantages: Larger amount of blood is needed compared to other methods. 2. Wintrobe Method The Wintrobe method is less commonly used but still recognized in certain settings. It uses a shorter and narrower tube compared to the Westergren method. Procedure: o Blood is drawn into a tube containing an anticoagulant (EDTA). o The blood is placed in a Wintrobe tube (100 mm long). o After 1 hour, the distance the RBCs have settled is measured. Normal ESR Values (Wintrobe method): o Men: 0-9 mm/hour o Women: 0-20 mm/hour Advantages: Uses a smaller blood sample, and the same sample can be used for other hematological tests like hematocrit. Disadvantages: Less sensitive to elevated ESR than the Westergren method. 3. Modified Westergren Method This is a slight modification of the Westergren method, used for faster processing. Procedure: o Blood is collected in a tube containing EDTA (ethylenediaminetetraacetic acid) as the anticoagulant. o The sample is diluted with saline or sodium citrate, and the measurement is performed similarly to the standard Westergren method. Advantages: It is faster and more practical for automated systems. 4. Automated ESR Methods In modern clinical laboratories, automated analyzers are increasingly used to measure ESR. These devices are based on the principle of the Westergren method but reduce the time and human error involved. Types of automated methods: o Capillary methods: Use small capillary tubes instead of large Westergren tubes. o Optical methods: These machines use optical sensors to detect the RBC sedimentation rate within a few minutes. Advantages: Faster, standardized, and less prone to human error. Disadvantages: More expensive due to the need for specialized equipment. 5. Zeta Sedimentation Ratio (ZSR) This is an alternative method used in some cases to measure ESR, which is not affected by anemia. Procedure: o Blood is drawn and placed in a capillary tube. o A correction factor is applied to account for hematocrit levels, thus eliminating the impact of anemia on the ESR value. Advantages: More accurate in patients with abnormal hematocrit (e.g., in severe anemia or polycythemia). 6. Micro ESR Method Used primarily in pediatric and neonatal patients because of the smaller blood sample volume required. Procedure: o Blood is collected into a narrow capillary tube (similar to a microhematocrit tube). o The tube is held vertically, and the rate at which RBCs settle is observed over time. Advantages: Requires a very small sample size, making it ideal for newborns and infants. Comparison of ESR Methods Method Tube Size Time Sample Size Sensitivity Westergren Method 200 mm 1 hour Larger sample High sensitivity Wintrobe Method 100 mm 1 hour Smaller sample Lower sensitivity Modified Westergren 200 mm Faster than 1 hour Larger sample High sensitivity Automated ESR Variable Few minutes Depends on machine High sensitivity ZSR Capillary tube Variable Small sample Corrects for hematocrit Micro ESR Capillary tube Few minutes Very small sample Moderate sensitivity Clinical Significance of ESR ESR is not disease-specific but can indicate the presence of inflammation, infection, or malignancies. Common conditions associated with an elevated ESR include: Infections Autoimmune diseases (e.g., rheumatoid arthritis, lupus) Inflammatory conditions (e.g., vasculitis, temporal arteritis) Malignancies (e.g., multiple myeloma, lymphoma) However, it is important to note that ESR can be influenced by factors such as age, gender, and pregnancy. Therefore, ESR is often used in conjunction with other diagnostic tests. I. Introduction to Erythropoiesis and Erythrocyte Lifecycle 1. Definition of Erythropoiesis 1. The process of producing red blood cells (RBCs) or erythrocytes. 2. Overview of Erythrocyte Function 1. Transport of oxygen (O₂) from the lungs to tissues. 2. Transport of carbon dioxide (CO₂) from tissues to the lungs. 3. Erythrocyte Lifecycle 1. Lifespan of approximately 120 days. 2. Production in the bone marrow and destruction in the spleen, liver, and bone marrow. II. Erythropoiesis: The Production of Red Blood Cells 1. Sites of Erythropoiesis 1. Fetal life: Liver, spleen, and later bone marrow. 2. Postnatal life: Exclusively in the bone marrow. 2. Hematopoietic Stem Cells (HSCs) 1. Role of pluripotent HSCs in the differentiation to erythroid progenitors. 3. Stages of Erythroid Maturation 1. Proerythroblast 1. Large nucleus, basophilic cytoplasm. 2. Basophilic Erythroblast 2. More basophilic cytoplasm, less condensed chromatin. 3. Polychromatophilic Erythroblast 3. Hemoglobin synthesis begins, mixed cytoplasmic staining. 4. Orthochromic Erythroblast (Normoblast) 4. Eosinophilic cytoplasm, pyknotic nucleus. 5. Reticulocyte 5. Immature erythrocyte, no nucleus, some residual RNA. 6. Mature Erythrocyte 1. Fully hemoglobinized, biconcave disc shape, no organelles. 4. Regulation of Erythropoiesis 1. Erythropoietin (EPO): Hormonal regulation by the kidneys. 2. Hypoxia as a Stimulus: Low oxygen levels trigger increased EPO production. 3. Role of growth factors (GM-CSF, IL-3, SCF). III. Destruction of Erythrocytes: Erythrophagocytosis 1. Senescence of Red Blood Cells 1. Aging RBCs undergo membrane and enzymatic changes. 2. Loss of deformability and exposure of phosphatidylserine (PS). 2. Role of the Spleen in Erythrocyte Destruction 1. Red Pulp: Filters out senescent and damaged RBCs. 2. Macrophages phagocytose defective erythrocytes. 3. Extravascular Hemolysis 1. Occurs mainly in the spleen and liver. 2. Hemoglobin is broken down into heme and globin. 3. Iron is recycled, and bilirubin is produced from heme breakdown. 4. Intravascular Hemolysis 1. Occurs within the blood vessels. 2. Free hemoglobin binds to haptoglobin and is cleared by the liver. 5. Iron Recycling and Storage 1. Ferritin and hemosiderin: Storage forms of iron. 2. Iron from degraded erythrocytes is reused for new RBC synthesis. IV. Disorders Related to Erythropoiesis and Destruction 1. Ineffective Erythropoiesis 1. Anemias resulting from defects in RBC production. 2. Examples: Sideroblastic anemia, megaloblastic anemia. 2. Excessive Erythrocyte Destruction 1. Hemolytic Anemias: Premature RBC destruction. 2. Inherited causes: Sickle cell anemia, hereditary spherocytosis. 3. Acquired causes: Autoimmune hemolytic anemia, microangiopathic hemolytic anemia. 3. Polycythemia 1. Overproduction of erythrocytes. 2. Primary polycythemia (Polycythemia vera) vs. secondary causes (hypoxia, tumors). 4. Aplastic Anemia 1. Failure of the bone marrow to produce adequate RBCs. V. Laboratory Evaluation of Erythropoiesis and Destruction 1. Complete Blood Count (CBC) and Reticulocyte Count 1. Hemoglobin (Hb), Hematocrit (Hct), RBC indices (MCV, MCH, MCHC). 2. Reticulocyte count to assess bone marrow activity. 2. Bone Marrow Examination 1. Evaluation of erythroid hyperplasia or hypoplasia. 3. Erythrocyte Morphology 1. Blood smears: Anisocytosis, poikilocytosis, polychromasia. 2. RBC inclusions: Howell-Jolly bodies, basophilic stippling. 4. Iron Studies 1. Serum iron, ferritin, transferrin saturation, and total iron-binding capacity (TIBC). 5. Hemolysis Markers 1. Elevated bilirubin, decreased haptoglobin, elevated lactate dehydrogenase (LDH). 2. Urine tests for hemoglobinuria and hemosiderinuria. VI. Clinical Significance 1. Impact of Abnormal Erythropoiesis 1. Clinical presentation and complications of anemia. 2. Monitoring Erythropoiesis in Disease 1. Assessment of therapeutic response (e.g., in treatment of anemia of chronic disease). 3. Erythropoiesis-Stimulating Agents (ESAs) 1. Use of synthetic EPO in clinical management (renal failure, chemotherapy-induced anemia). I. Introduction to Erythrocyte Metabolism and Membrane Function 1. Overview of Erythrocyte Metabolism 1. Lack of mitochondria: RBCs rely on anaerobic glycolysis for energy. 2. Role in maintaining cell integrity and function, despite a simple structure. 2. Importance of Membrane Structure 1. Maintaining erythrocyte shape (biconcave disc) for optimal gas exchange. 2. Flexibility and deformability for passage through capillaries. II. Erythrocyte Metabolism 1. Glycolytic Pathway (Embden-Meyerhof Pathway) 1. Primary source of ATP production in RBCs (anaerobic metabolism). 2. Glucose as the primary fuel. 3. ATP generated through substrate-level phosphorylation. 2. Hexose Monophosphate Shunt (HMP Shunt or Pentose Phosphate Pathway) 1. Produces NADPH, critical for maintaining glutathione in its reduced form. 2. Protects RBCs from oxidative stress by neutralizing reactive oxygen species (ROS). 3. Deficiency in G6PD (Glucose-6-Phosphate Dehydrogenase) leads to hemolytic anemia due to oxidative damage. 3. Methemoglobin Reductase Pathway 1. Maintains hemoglobin in its reduced (ferrous, Fe²⁺) state. 2. Prevents accumulation of methemoglobin (oxidized hemoglobin that cannot carry oxygen). 3. Deficiency in methemoglobin reductase can lead to methemoglobinemia. 4. Rapoport-Luebering Shunt 1. Controls the levels of 2,3-Bisphosphoglycerate (2,3-BPG), which regulates oxygen binding and release. 2. Increased 2,3-BPG promotes oxygen release to tissues by reducing hemoglobin’s affinity for oxygen. III. Erythrocyte Membrane Structure 1. Lipid Bilayer 1. Composed of phospholipids, cholesterol, and glycolipids. 2. Phospholipid distribution: Asymmetry is critical for membrane stability (e.g., phosphatidylserine on the inner leaflet). 3. Role of cholesterol in membrane fluidity and stability. 2. Membrane Proteins 1. Integral Proteins 1. Spanning the lipid bilayer, involved in ion transport and maintaining RBC shape. 2. Examples: Band 3 protein (anion exchanger), glycophorin (gives negative charge to RBC surface). 2. Peripheral Proteins 3. Attached to the inner surface of the membrane and involved in cytoskeletal structure. 4. Examples: Spectrin, ankyrin, protein 4.1, protein 4.2. 3. Cytoskeleton 1. Provides structural integrity and flexibility to the RBC membrane. 2. Spectrin-Actin Network: Anchors to membrane proteins through ankyrin and protein 4.1. 3. Abnormalities in cytoskeletal proteins (e.g., spectrin deficiency) lead to membrane fragility and conditions like hereditary spherocytosis. IV. Function of the Erythrocyte Membrane 1. Maintaining RBC Shape 1. Biconcave shape provides optimal surface area for gas exchange and allows flexibility. 2. Deformability and Stability 1. Critical for navigating narrow capillaries and splenic sinusoids. 2. Membrane proteins and cytoskeletal integrity ensure elasticity. 3. Altered deformability leads to hemolysis and sequestration in the spleen (e.g., in hereditary elliptocytosis). 3. Permeability Regulation 1. Control of ion and water balance. 2. Key ion transporters: Sodium-potassium (Na⁺/K⁺) ATPase, calcium ATPase, and Band 3 (anion exchange for chloride and bicarbonate). 3. Abnormal ion transport can lead to osmotic fragility and cell lysis. 4. Membrane Repair Mechanisms 1. RBCs are constantly subjected to mechanical stress. 2. Membrane repair and turnover of phospholipids to prevent premature hemolysis. V. Membrane Abnormalities and Associated Diseases 1. Hereditary Spherocytosis 1. Defects in ankyrin, spectrin, or other membrane-cytoskeleton interactions. 2. Results in spherical, less deformable RBCs that are prone to destruction in the spleen. 2. Hereditary Elliptocytosis 1. Mutations in spectrin or protein 4.1 lead to elliptical RBCs with reduced deformability. 3. Hereditary Stomatocytosis 1. Increased permeability to sodium and potassium. 2. Characteristic cup-shaped (stomatocytic) RBCs. 4. Paroxysmal Nocturnal Hemoglobinuria (PNH) 1. Deficiency of GPI-anchored proteins due to mutations in the PIGA gene. 2. Results in increased sensitivity to complement-mediated lysis, leading to intravascular hemolysis. 5. G6PD Deficiency 1. Impaired ability to detoxify oxidative stress. 2. Hemolysis triggered by oxidative agents (e.g., certain drugs, infections). VI. Laboratory Evaluation of Erythrocyte Metabolism and Membrane Function 1. Osmotic Fragility Test 1. Measures RBC resistance to hemolysis in hypotonic solutions. 2. Increased fragility in hereditary spherocytosis. 2. Erythrocyte Enzyme Assays 1. Evaluation of G6PD activity and other metabolic enzymes. 2. Detects enzyme deficiencies that predispose RBCs to oxidative damage. 3. Ektacytometry 1. Measures RBC deformability and membrane elasticity. 2. Used in the diagnosis of hereditary elliptocytosis and spherocytosis. 4. Flow Cytometry for PNH 1. Detects absence of GPI-anchored proteins (e.g., CD55, CD59) on RBCs in PNH. VII. Clinical Significance 1. Impact of Metabolic Disorders 1. Clinical presentations of RBC enzyme deficiencies (e.g., G6PD deficiency hemolysis). 2. Membrane Disorders and Hemolytic Anemias 1. Understanding the role of membrane defects in hereditary anemias. 3. Therapeutic Approaches 1. Treatment options for metabolic and membrane abnormalities (e.g., splenectomy in hereditary spherocytosis, supportive care in G6PD deficiency). I. Introduction to Hemoglobin Metabolism 1. Definition of Hemoglobin (Hb) 1. Hemoglobin is the iron-containing oxygen transport protein in erythrocytes. 2. Structure of Hemoglobin 1. Composed of four polypeptide globin chains (tetramer) and four heme groups. 2. Globin chains: Two alpha (α) and two beta (β) in adult hemoglobin (HbA). 3. Heme group: Contains ferrous iron (Fe²⁺) that binds oxygen (O₂). II. Synthesis of Hemoglobin 1. Globin Synthesis 1. Occurs in the ribosomes within erythroblasts. 2. Controlled by genes on chromosomes 11 (beta-like chains) and 16 (alpha-like chains). 2. Heme Synthesis 1. Occurs partly in the mitochondria and partly in the cytoplasm of erythroblasts. 2. Steps in Heme synthesis: 1. Delta-aminolevulinic acid (ALA) formation. 2. Formation of porphobilinogen. 3. Production of uroporphyrinogen. 4. Conversion to coproporphyrinogen. 5. Synthesis of protoporphyrin IX. 6. Incorporation of ferrous iron (Fe²⁺) into protoporphyrin IX to form heme. 3. Enzymes involved: ALA synthase, ferrochelatase, etc. 4. Rate-limiting step: ALA synthase. 3. Hemoglobin Assembly 1. Combination of two alpha and two beta chains with four heme molecules. 2. Other forms of hemoglobin: Fetal hemoglobin (HbF: α₂γ₂), Hemoglobin A2 (HbA2: α₂δ₂). III. Functions of Hemoglobin 1. Oxygen Transport 1. Hemoglobin binds O₂ in the lungs and releases it in tissues. 2. Carbon Dioxide Transport 1. Carries CO₂ as carbaminohemoglobin or converted into bicarbonate (HCO₃⁻) in RBCs. 3. Buffering Role 1. Hemoglobin acts as a buffer, maintaining pH balance by binding or releasing protons (H⁺). 4. Oxygen Affinity and Regulation 1. Oxygen affinity is regulated by: 1. 2,3-Bisphosphoglycerate (2,3-BPG): Decreases oxygen affinity, promoting O₂ release. 2. Bohr Effect: Decreased pH reduces O₂ affinity, facilitating O₂ delivery in acidic environments. 3. Haldane Effect: Binding of O₂ reduces hemoglobin's affinity for CO₂ and H⁺. IV. Hemoglobin Breakdown (Degradation) 1. Senescence of Erythrocytes 1. Average RBC lifespan is 120 days. 2. Old and damaged erythrocytes are removed primarily by macrophages in the spleen (extravascular hemolysis) or within blood vessels (intravascular hemolysis). 2. Heme Breakdown 1. Heme is broken down into: 1. Biliverdin (green pigment) by the enzyme heme oxygenase. 2. Bilirubin (yellow pigment) by the enzyme biliverdin reductase. 3. Excretion of Bilirubin 1. Bilirubin is transported in the blood bound to albumin (unconjugated bilirubin). 2. In the liver, bilirubin is conjugated with glucuronic acid to form conjugated bilirubin (water-soluble). 3. Conjugated bilirubin is excreted into bile and ultimately into the intestine. 4. In the intestine, bilirubin is converted to urobilinogen and stercobilin, responsible for the color of urine and feces, respectively. 4. Iron Recycling 1. Iron from degraded hemoglobin is recycled by macrophages. 2. Stored as ferritin or hemosiderin in tissues or transported by transferrin for reuse in erythropoiesis. V. Hemoglobin Variants 1. Normal Hemoglobin Variants 1. HbA (Adult Hemoglobin): α₂β₂, predominant in adults. 2. HbA2: α₂δ₂, minor component in adults. 3. HbF (Fetal Hemoglobin): α₂γ₂, predominant in fetuses and newborns. 2. Abnormal Hemoglobin Variants 1. Hemoglobinopathies: Genetic mutations in globin genes. 1. Sickle Cell Hemoglobin (HbS): A point mutation in the beta-globin gene. 2. Hemoglobin C (HbC): Mutation causing decreased solubility. 3. Hemoglobin E (HbE): Common in Southeast Asia, mild anemia. 2. Thalassemias: Deficient production of alpha or beta globin chains. 1. Alpha Thalassemia: Reduced alpha-globin synthesis, causing excess beta chains. 2. Beta Thalassemia: Reduced beta-globin synthesis, causing excess alpha chains. VI. Disorders of Hemoglobin Metabolism 1. Hemoglobinopathies 1. Sickle Cell Disease: HbS polymerizes under low oxygen tension, causing RBC sickling. 2. Hemoglobin C Disease: Causes mild hemolytic anemia and splenomegaly. 2. Thalassemias 1. Alpha and beta thalassemias lead to ineffective erythropoiesis, microcytic anemia, and hemolysis. 3. Methemoglobinemia 1. Increased levels of methemoglobin (oxidized hemoglobin, Fe³⁺), which cannot bind oxygen. 2. Causes cyanosis and tissue hypoxia. 4. Porphyrias 1. Defects in heme biosynthesis pathway. 2. Accumulation of porphyrin precursors leads to photosensitivity, abdominal pain, and neuropsychiatric symptoms. 5. Iron Metabolism Disorders 1. Iron Deficiency Anemia: Lack of iron leads to impaired hemoglobin synthesis. 2. Hereditary Hemochromatosis: Excessive iron absorption and deposition in tissues. 6. Anemias of Chronic Disease 1. Impaired iron utilization in chronic inflammatory states, leading to decreased hemoglobin production. VII. Laboratory Evaluation of Hemoglobin Metabolism 1. Hemoglobin Concentration (Hb) 1. Part of the complete blood count (CBC). 2. Low hemoglobin levels indicate anemia. 2. Hemoglobin Electrophoresis 1. Used to identify different hemoglobin variants. 2. Differentiates between HbA, HbF, HbS, HbC, HbE, etc. 3. Oxygen Dissociation Curve 1. Assesses hemoglobin’s oxygen affinity. 2. Shifts in the curve indicate changes in oxygen delivery capacity (e.g., 2,3-BPG effects). 4. Iron Studies 1. Measures serum iron, ferritin, total iron-binding capacity (TIBC), and transferrin saturation. 2. Helps assess iron availability and utilization in hemoglobin synthesis. 5. Bilirubin Measurement 1. Unconjugated and conjugated bilirubin levels assess the breakdown of hemoglobin. 2. Elevated bilirubin indicates increased hemolysis or impaired liver function. VIII. Clinical Significance 1. Impact of Abnormal Hemoglobin Metabolism 1. Anemia, jaundice, and hypoxia resulting from hemoglobin disorders. 2. Therapeutic Approaches 1. Treatment of hemoglobinopathies (e.g., hydroxyurea for sickle cell disease). 2. Iron supplementation or chelation therapy for iron metabolism disorders. Detailed methods may be found in the lab manual.