Exam 2 Review Slides PDF
Document Details
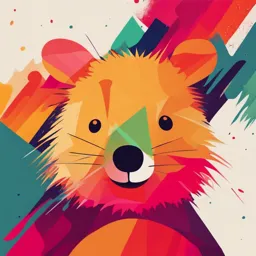
Uploaded by HardWorkingElegy
Tags
Summary
This document appears to be a review of exam 2 material covering exercise physiology. The document includes information about the circulatory and respiratory systems as well as training and acid-base balance in exercise. The review includes figures and diagrams.
Full Transcript
Exam 2 KIN 4312 Exercise Physiology Total 50 questions; Multiple choice questions + true/false questions Total 150 points (3 per one question) Chapter 9 – Circulatory Response to Exercise (14 questions)...
Exam 2 KIN 4312 Exercise Physiology Total 50 questions; Multiple choice questions + true/false questions Total 150 points (3 per one question) Chapter 9 – Circulatory Response to Exercise (14 questions) Chapter 10 – Respiration During Exercise (15 questions) Chapter 13 – Physiology of Training (14 questions) Chapter 11 – Acid-Base Balance (7 questions) 1 2 Function of Circulatory System Works with the pulmonary system Cardiopulmonary or cardiorespiratory system Q1 Q2 Purposes of the cardiorespiratory system Transport O2 and nutrients to tissues } Blood Removal of CO2 and wastes from tissues circulation Regulation of body temperature Two major adjustments of blood flow during exercise Increased cardiac output Redistribution of blood flow from inactive organs to active muscle 3 1 Cardiovascular Anatomy: Heart Q3 - AVs prevent backflow of blood from the ventricles into the atria - Semilunar valves prevent backflow of blood from the arteries into the ventricles 4 Pulmonary and Systemic Circuits Pulmonary circuit Q4 Right side of the heart Pumps deoxygenated blood to the lungs via pulmonary arteries Returns oxygenated blood to the left side of the heart via pulmonary veins - provides oxygen to the blood 5 Cardiac Cycle at Rest and During Exercise CONFIRMING PAGES At rest, diastole longer than systole During exercise, both systole and diastole are shorter Q5 Systole Diastole Rest Each beat Heart rate = 75 beats/min 0.3 second 0.5 second Systole > Diastole Heavy exercise Each beat Heart rate = 180 beats/min 0.2 second 0.13 second Figure 9.5 Illustration of cardiac cycle at rest and during exercise. Notice that increases in heart rate during exercise are achieved primarily through Contraction phasea decrease in the time spent in diastole; however, at high heart rates, the length of time spent in systole also decreases. diastole. A healthy 21-year-old female might have an Time (seconds) average resting heart rate of 75 beats per minute. This 6 0 0.2 0.4 0.6 0.8 120 means that the total cardiac cycle lasts 0.8 second, with 0.5 second spent in diastole and the remaining 100 0.3 second dedicated to systole (17) (see Fig. 9.5). If the heart rate increases from 75 beats per minute to Pressure (mm Hg) 80 180 beats per minute (e.g., heavy exercise), there is a Ventricle reduction in the time spent in both systole and dias- 60 tole (11). This point is illustrated in Fig. 9.5. Note that a rising heart rate results in a greater time reduction 40 in diastole, whereas systole is less affected. 20 Pressure Changes During the Cardiac Cycle Dur- ing the cardiac cycle, the pressure within the heart 0 Systole Diastole chambers rises and falls. When the atria are relaxed, blood flows into them from the venous circulation. As 120 2 Volume (ml) these chambers fill, the pressure inside gradually in- creases. Approximately 70% of the blood entering the 80 atria during diastole flows directly into the ventricles 40 through the atrioventricular valves before the atria Factors that Influence Arterial Blood Pressure Q6 Determinants of mean arterial pressure (MAP) Cardiac output Total vascular resistance MAP = cardiac output (Q) x total vascular resistance Q=HR x SV (Q ) (HR) (SV) Resistance 7 Regulation of blood pressure Q7 Short-term regulation Sympathetic nervous system (SNS) Baroreceptors in aorta and carotid arteries Vascular Increase in BP = decreased SNS activity Q & Resistance Decrease in BP = increased SNS activity Blood Volume Long-term regulation Kidneys Baroreceptors Carotid sinus baroreceptor Via control of blood volume Aortic arch baroreceptor 8 Normal Electrocardiogram CONFIRMING PAGES 0.8 second Q8 P-wave: Atrial depolarization R R +1 QRS: PQ segment ST segment Ventricular depolarization & atrial repolarization Millivolts P wave T wave 0 ST/T-wave: PR Q interval S QT –1 interval QRS interval Ventricular repolarization Atria Ventricles Atria Ventricles contract contract contract contract Figure 9.11 The normal electrocardiogram during rest. CARDIAC OUTPUT controls heart rate, changes in heart rate often involve factors that influence the SA node. The two most prom- · ) is the product of the heart rate inent factors that influence heart rate are the parasym- Cardiac output (Q (HR) and the stroke volume (SV) (amount of blood pathetic and sympathetic nervous systems (25). 9 pumped per heartbeat): The parasympathetic fibers that supply the heart arise from neurons in the cardiovascular control cen- Q? 5 HR 3 SV ter in the medulla oblongata and make up a portion Thus, cardiac output can be increased due to of the vagus nerve (4). Upon reaching the heart, a rise in either heart rate or stroke volume. During these fibers make contact with both the SA node and exercise in the upright position (e.g., running, cy- the AV node (see Fig. 9.14). When stimulated, these cling, etc.), the increase in cardiac output is due to nerve endings release acetylcholine, which causes a an increase in both heart rate and stroke volume. decrease in the activity of both the SA and AV nodes Table 9.2 presents typical values at rest and during due to hyperpolarization (i.e., moving the resting maximal exercise for heart rate, stroke volume, and membrane potential further from threshold). The end cardiac output in both untrained and highly trained result is a reduction of heart rate. Therefore, the para- endurance athletes. The gender differences in stroke sympathetic nervous system acts as a braking system volume and cardiac output are due mainly to differ- to slow down heart rate. ences in body sizes between men and women (2) Even at rest, the vagus nerves carry impulses to (see Table 9.2). the SA and AV nodes (25). This is often referred to as parasympathetic tone. As a consequence, changes in parasympathetic activity can cause heart rate Regulation of Heart Rate to increase or decrease. For instance, a decrease in During exercise, the quantity of blood pumped by the parasympathetic tone to the heart can elevate heart heart must change in accordance with the elevated rate, whereas an increase in parasympathetic activity skeletal muscle oxygen demand. Because the SA node causes a slowing of heart rate. 196 Section One Physiology of Exercise pow23534_ch09_185-214.indd 196 24/07/14 3:03 PM 3 Regulation of Heart Rate Q9 Parasympathetic nervous system Via vagus nerve Slows HR by inhibiting SA and AV node Sympathetic nervous system Via cardiac accelerator nerves Increases HR by stimulating SA and AV node Low resting HR due to parasympathetic tone Increase in HR at onset of exercise Initial increase due to parasympathetic withdrawal Up to ~100 beats/min Later increase due to increased SNS stimulation 10 CONFIRMING PAGES IN SUMMARY Blood pressure 240 Systolic Blood is composed of two principal compo- (mm Hg) 200 nents: plasma and cells. 160 Mean Blood flow through the vascular system is di- 120 rectly proportional to the pressure at the two Diastolic 80 ends of the system and inversely proportional 25 50 75 100 to resistance: D Pressure Blood flow 5 _____________________________ Resistance Stroke volume ˙ 2 max ~40% VO (ml beat−1) 140 The most important factor determining resis- 120 tance to blood flow is the radius of the blood vessel. The relationship between vessel radius, 100 vessel length, blood viscosity, and flow is 80 25 50 75 100 Changes in Cardiac Output During Exercise Length 3 Viscosity Resistance 5 ____________________________________________________ Radius4 The greatest vascular resistance to blood flow is 25 Cardiac output offered in the arterioles. (. min−1) 20 CONFIRMING PAGES 15 Q10 10 Cardiac output increases due to: 5 Q 25 = HR 50 x SV 75 100 Increased HR CHANGES IN OXYGEN IN SUMMARY DELIVERY TO MUSCLE pressure 240 Systolic Blood is composed of two principal compo- Linear increase to max Hg) 200 DURING EXERCISE min−1 ) nents: plasma and cells. 200 rate (beats (mm 160 Mean Blood flow through the vascular system is di- Blood 150 120 Heart rectly During proportional intense exercise, to thethe pressure need metabolic at thefor twooxy- Diastolic 100 80 For adults: Max HR = gen inends 220 of the skeletal – age systemincreases muscle to resistance: and inversely many proportional times over the resting value. To meet this rise in oxygen demand, 50 25 50 75 100 Pressure Dmuscle 25 50 75 100 blood flow to Blood the contracting must increase. flow 5 _____________________________ Resistance For children: Max As HR =skeletal 208 – 0.7 x agevia two mentioned earlier, increased oxygen delivery to Stroke volume ˙ 2 max ~40% VO (ml beat−1) 140 exercising The most musclefactor important is accomplished determining resis- 18 120 Arteriovenous mechanisms: tance to(1) an increased flow is thecardiac output and (2) O2 difference (ml 100ml−1) blood radius of the blood a redistribution vessel. Theof blood flowbetween relationship from inactive vessel organs radius, to 100 Increased SV vessel length, the working skeletalblood viscosity, and flow is muscle. 12 80 Length 3 Viscosity 25 50 75 100 Resistance 5 ____________________________________________________ 6 Increase, then plateau Changesatin40~60% Cardiac Output VO2 max The greatest vascular resistance to blood flow is Radius4 25 50 75 100 During Exercise 25 ˙ 2 max Cardiac output Percent VO No plateau in highly trained subjects offered in the arterioles. (. min−1) 20 Cardiac output increases during exercise in direct pro- Figure 9.22 Changes in blood pressure, stroke volume, 15 portion to the metabolic rate required to perform the cardiac output, heart rate, and the arterial–mixed ve- 10 nous oxygen difference as a function of relative work exercise task. This is shown in Fig. 9.22. Note that the relationship between cardiac output and percent max- rates. See5text for details. 25 50 75 100 CHANGES IN OXYGEN imal oxygen uptake is essentially linear. The increase in cardiac output during exercise in the upright posi- DELIVERY tion is achieved byTO MUSCLE an increase in both stroke volume for a 70-kg, active (but not highly trained) college-age 11 DURING EXERCISE (beats min−1) and heart rate. However, in untrained or moderately male. See 200 Table 9.2 for examples of maximal stroke vol- Heart rate trained subjects, stroke volume does not increase be- ume and150 cardiac output for trained men and women. During yond intense of a workload exercise, 40% to the 60%metabolic of V̇O2 maxneed(Fig.for9.22 oxy- ). Note 100 that although most experts agree that stroke gen in skeletal Therefore, at work muscle rates increases many greater than times 40% overV̇the to 60% O2 volume50 reaches a plateau between 40% to 60% of V̇O2 resting max, the value. rise inTocardiac meet output this riseininthese oxygen demand, individuals max in untrained and moderately trained individuals, 25 50 75 100 is blood flowby achieved to increases the contracting in heartmuscle must The rate alone. increase. ex- evidence exists that stroke volume does not plateau in As mentioned amples presentedearlier, in Fig.increased oxygen delivery 9.22 for maximal heart rate, to highly trained athletes during running exercise. This exercising skeletal muscle is accomplished via two stroke volume, and cardiac output are typical values point is18 discussed in more detail in Research Focus 9.1. Arteriovenous mechanisms: (1) an increased cardiac output and (2) O2 difference (ml 100ml−1) a redistribution of blood flow from inactive organs to the working skeletal muscle. 12 204 Section One Physiology of Exercise 6 Changes in Cardiac Output 25 50 75 100 During Exercise ˙ 2 max Percent VO Cardiac output increases during exercise in direct pro- Figure 9.22 Changes in blood pressure, stroke volume, pow23534_ch09_185-214.indd 204 cardiac output, heart rate, and the arterial–mixed ve- 24/07/14 3:04 PM portion to the metabolic rate required to perform the exercise task. This is shown in Fig. 9.22. Note that the nous oxygen difference as a function of relative work relationship between cardiac output and percent max- rates. See text for details. imal oxygen uptake is essentially linear. The increase in cardiac output during exercise in the upright posi- tion is achieved by an increase in both stroke volume for a 70-kg, active (but not highly trained) college-age and heart rate. However, in untrained or moderately male. See Table 9.2 for examples of maximal stroke vol- trained subjects, stroke volume does not increase be- ume and cardiac output for trained men and women. yond a workload of 40% to 60% of V̇O2 max (Fig. 9.22). Note that although most experts agree that stroke Therefore, at work rates greater than 40% to 60% V̇O2 volume reaches a plateau between 40% to 60% of V̇O2 max, the rise in cardiac output in these individuals max in untrained and moderately trained individuals, is achieved by increases in heart rate alone. The ex- evidence exists that stroke volume does not plateau in amples presented in Fig. 9.22 for maximal heart rate, highly trained athletes during running exercise. This stroke volume, and cardiac output are typical values point is discussed in more detail in Research Focus 9.1. Q11 204 Section One Physiology of Exercise pow23534_ch09_185-214.indd 204 24/07/14 3:04 PM 12 4 Q12 Heart Rate Variability (HRV) The time between heart beats R R R R Balance between SNS and PNS Sympathovagal balance (active regulation) High variation in HRV is considered “healthy” Exercise training promotes high levels of HRV Low HRV is a predictor of cardiovascular morbidity and mortality In patients with existing cardiovascular disease 13 End-Diastolic Volume Frank-Starling mechanism Greater EDV results in a more forceful contraction Due to stretch of ventricles Dependent on venous return (blood flow back to the heart) Venous return increased by: Q13 Venoconstriction Via SNS Vein Skeletal muscle pump Rhythmic skeletal muscle contractions force blood in the extremities toward the heart One-way valves in veins prevent backflow of blood Respiratory pump Changes in thoracic pressure pull blood toward heart 14 Prolonged Exercise Q14 Cardiac output is maintained Gradual decrease in stroke volume Due to dehydration and reduced plasma volume →↓ venous return Gradual increase in heart rate Particularly in heat Cardiovascular drift 15 5 16 Function of Respiratory Function System of the Lung Means of gas exchange between the external environment and the body Replacing O 2 Removing CO 2 Diffusion Regulation of acid-base balance Q1 Ventilation Mechanical process of moving air into and out of lungs gases Diffusion Random movement of molecules from an area of high concentration to an area of lower concentration CONFIRMING PAGES 17 Number of branches Conducting zone Respiratory zone Terminal bronchiole (1) Trachea (2) Primary bronchus Respiratory bronchioles (500,000) Bronchial tree Alveolar sacs (8 million) Alveolus (60,000) Terminal bronchioles Figure 10.4 The bronchial tree consists of the passageways that connect the trachea and the alveoli. functions to filter and humidify the air as it moves IN SUMMARY toward the respiratory zone of the lung. Regardless of Anatomically, the pulmonary system consists of the temperature or humidity of the environment, the a group of passages that filter air and transport air that reaches the lung is warmed and is saturated Type II Alveolar Cells it into the lungs where gas exchange occurs with water vapor (67, 69). This warming and humidi- within tiny air sacs called alveoli. fication of air prevents the delicate lung tissue from The air passages of the respiratory system are desiccation (drying out) during exercise when breath- divided into two functional zones: (1) conduct- ing is increased (67, 98). ing zone; and (2) respiratory zone. Respiratory Zone Fluid with surfactant Gas exchange in the lungs occurs across about Type I alveolar cell Type II alveolar cell Fragility of tiny alveoli due totiny 300 million surface tension alveoli. The enormous number of these structures provides the lung with a large sur- face area for diffusion. Indeed, it is estimated that the total surface area available for diffusion in the Q2 human lung is 60 to 80 square meters (i.e., size of Three types of alveolar cell a tennis court). The rate of gas diffusion is further Alveolus - Type I and II in alveoli wall assisted by the fact that each alveolus is only one cell layer thick, so the total blood-gas barrier is only two cell layers thick (alveolar cell and capillary cell) White blood cell Macrophage - Alveolar macrophage (see Fig. 10.5). Red blood cell Although the alveoli provide the ideal structure for gas exchange, the fragility of these tiny “bubbles” presents some problems for the lung. For example, Type II cells because of the surface tension (pressure exerted due to the properties of water) of the liquid lining the - Release pulmonary surfactant alveoli, relatively large forces develop, which tend to collapse alveoli. Fortunately, some of the alveolar - Lowers surface tension of alveoli cells (called type II, see Fig. 10.5) synthesize and re- Capillary lease a material called surfactant, which lowers the - Prevents collapse of alveoli surface tension of the alveoli and thus prevents their Figure 10.5 Surface tension Relationship between type II alveolar cells collapse (60). and the alveolus. 218 Section One Physiology of Exercise Surfactant Surface tension: pow23534_ch10_215-245.indd tendency of liquid 218 surface to shrink 24/07/14 3:08 PM Water molecule 18 6 Mechanics of Inspiration Movement of air into the lungs Inspiration Q3 Inspiration Diaphragm pushes downward, ribs lift outward Volume of lungs increases Intrapulmonary pressure lowered Diaphragm (contraction) 19 Airway Resistance Q4 Airflow depends on: Pressure difference between two ends of airway Resistance of airways P1=Pressure in the alveoli P1 – P2 Airflow = P2= Atmospheric pressure outside the body Resistance Resistance in airway Airway resistance depends on diameter of airway Chronic obstructive pulmonary disease (COPD) Asthma and exercise-induced asthma 20 Exercise and Chronic Obstructive Pulmonary Disease Chronic obstructive pulmonary disease (COPD) Q5 Increased airway resistance Bronchitis Emphysema Due to constant airway narrowing Decreased expiratory airflow Includes two lung diseases: Chronic bronchitis Excessive mucus blocks airways (cough with mucus, shortness of breath) Emphysema Alveolar damage - reduced lung surface, airway collapse and increased resistance Increased work of breathing Leads to shortness of breath May interfere with exercise and activities of daily living 21 7 Pulmonary Ventilation Q6 Alveolar ventilation (VA) Alveoli Volume of air that reaches the respiratory zone Dead-space ventilation (VD) Anatom ical dead space Volume of air remaining in conducting airways V = VA + VD Conducting Zone VD Respiratory Zone VA 22 Spirometry Measurement of pulmonary volumes and rate of expired airflow Useful for diagnosing lung diseases Chronic obstructive pulmonary disease (COPD) ↓Vital capacity, ↓rate of expired airflow Q7 CONFIRMING PAGES Spirometric tests Vital capacity (VC) Maximal volume of air that can be expired after maximal inspiration CO2 O2 Ventilation Blood flow Ratio (L/min) (L/min) Forced expiratory volume (FEV1) Apex 0.24 0.07 3.43 Pulmonary Volume of air expired in the 1st second during maximal expiration circulation Pulmonary Lungs Pulmonary artery vein FEV1/VC ratio R.A. L.A. R.V. ≥ 80 % is normal for healthy individuals Heart L.V. Hepatic 23 artery Base 0.82 1.29 0.64 Vena cava Systemic Aorta Liver circulation Hepatic portal vein Intestines Figure 10.14 The relationship between ventilation and blood flow (ventilation/perfusion ratios) at the top Kidneys (apex) and the base of the lung. The ratios indicate that the base of the lung is overperfused relative to Tissue capillaries ventilation and that the apex is underperfused relative to ventilation. This uneven matching of blood flow to ventilation results in less-than-perfect gas exchange. CO2 O2 Fluid Fluid generally not equal to 1.0 throughout the lung, but varies depending on the section of the lung being con- Tissue cells sidered (32, 40, 57, 67, 117). This concept is illustrated Figure 10.12 The pulmonary circulation is a low- in Fig. 10.14, where the V/Q ratio at the top and the pressure system that pumps mixed venous blood base of the lung is calculated for resting conditions. through the pulmonary capillaries for gas exchange. Let’s discuss the V/Q ratio in the apex of the lung first. Here, the ventilation (at rest) in the upper Regional Blood Flow within the Lung After the completion of gas exchange, this oxygen- ated blood is returned to the left heart chambers to be region of the lung is estimated to be 0.24 liter/ circulated throughout the body. minute, whereas the blood flow is predicted to be 0.07 liter/minute. Thus, the V/Q ratio is 3.4 (i.e., 0.24/0.07 5 3.4). A large V/Q ratio represents a dis- proportionately high ventilation relative to blood Q8 flow, which results in poor gas exchange. In contrast, the ventilation at the base of the lung (Fig. 10.14) is When standing, most of the blood flow is Top 0.82 liter/minute, with a blood flow of 1.29 liters/ minute (V/Q ratio 5 0.82/1.29 5 0.64). A V/Q ratio distributed to the bottom of the lung less than 1.0 represents a greater blood flow than Due to gravitational force ventilation to the region in question. Although V/Q ratios less than 1.0 are not indicative of ideal con- Blood flow Bottom ditions for gas exchange, in most cases, V/Q ratios greater than 0.50 are adequate to meet the gas ex- During exercise, more blood flow to the top change demands at rest (117). What effect does exercise have on the V/Q ratio? Light-to-moderate exercise improves the V/Q relationship, whereas heavy exercise may result in a Uneven blood distribution affects gas Bottom 5 4 3 2 Top small V/Q inequality and thus, a minor impairment in gas exchange (56). Whether the increase in V/Q exchange Rib number inequality is due to low ventilation or low perfu- Figure 10.13 Regional blood flow within the lung. Note sion is not clear. The possible effects of this V/Q that there is a linear decrease in blood flow from the mismatch on blood gases will be discussed later in lower regions of the lung toward the upper regions. the chapter. www.mhhe.com/powers9e Chapter Ten Respiration During Exercise 227 24 pow23534_ch10_215-245.indd 227 24/07/14 3:08 PM 8 O2 Transport in the Blood Q9 Hemoglobin 99% of O 2 is transported bound to hemoglobin (Hb) Oxyhemoglobin: Hb bound to O2 (four) P o ly p e p tid e ch a in H em e Deoxyhemoglobin: Hb not bound to O2 O2 transport depends on the Hb concentration Iro n O xygen Each gram of Hb can transport 1.34 ml of O2 m o le cu le Red blood cell Oxygen content of blood (100% Hb saturation) Males: 150 g Hb/L of blood x 1.34 ml O2/g Hb = 200 ml O2/L of blood Females: 130 g Hb/L of blood x 1.34 ml O2/g Hb = 174 ml O2/L of blood 25 Factors that influence the O2-Hb Dissociation Curve Q10 pH Body temperature 2,3-diphosphoglycerate Oxyhem oglobin Deoxyhem oglobin 26 O2 Transport in Muscle Q11 Myoglobin (Mb) Shuttles between the muscle cell membrane and the mitochondria Large quantities in slow muscle fibers Mb has a higher affinity for O2 than hemoglobin Even at low PO 2 Allows Mb to store O 2 O2 reserve for muscle Buffers O2 needs at onset of exercise until cardiopulmonary system increases O2 delivery 27 9 (pH would decrease). The role of the pulmonary system in acid-base balance is discussed in detail in Chap. 11. 0 Rest 0 1 2 3 4 5 6 IN SUMMARY Exercise time (min) An increase in pulmonary ventilation causes Figure 10.20 The changes in ventilation and partial exhalation of additional CO2, which results in pressures of O2 and CO2 in the transition from rest to a reduction of blood PCO2 and a lowering of steady-state submaximal exercise. hydrogen ion concentration (i.e., pH increases). Myoglobin is the oxygen-binding protein found exercise (below the lactate threshold) in two different in muscle and acts as a shuttle to move O2 from environmental conditions. The neutral environment the muscle cell membrane to the mitochondria. represents exercise in a cool, low-relative-humidity environment (19°C, 45% relative humidity). The sec- VENTILATORY AND ond condition represented in Fig. 10.21 is a hot/ BLOOD-GAS RESPONSES high-humidity environment, which hampers heat loss from the body. The major point to appreciate from TO EXERCISE Fig. 10.21 is that ventilation tends to “drift” upward during prolonged work. The mechanism to explain Before discussing ventilatory control during exercise, this increase in V̇E during work in the heat is an in- we should examine the ventilatory response to sev- crease in blood temperature, which directly affects eral types of exercise. the respiratory control center (88). Prolonged Exercise in a Hot Environment Another interesting point illustrated in Fig. 10.21 Rest-to-Work Transitions is that although ventilation is greater during exercise in a hot/humid environment when compared to work The Q12 change in pulmonary ventilation observed in the transition from rest to constant-load submaximal exercise During prolonged submaximal (i.e., below the lactate threshold) is pictured in Fig. 10.20. 41 Note thatexercise in a hot/humid expired ventilation (V̇E ) increases abruptly at 40 environment: the beginning of exercise, followed by a slower rise to- PCO2 39 ward a steady-state value (8, 21, 22, 38, 53, 80, 87, 120). (mm Hg) 38 Hot/humid environment Figure 10.20 also points out that arterial tensions of PCO2 and PO2 are relatively unchanged during this Cool environment (Neutral) Ventilation tends to drift upward type of exercise (35, 37, 113). However, note that arte- 60 Increased rial PO2 decreases blood and arterial temperature PCO2 tends to increase slightly in theaffects transitionrespiratory control center from rest to steady-state ex- V˙ E 40 (,/min) ercise (37). This observation suggests that the increase in alveolar ventilation at the beginning of exercise is 20 Little not as rapid as the change increase in in PCO2 metabolism. 0 Higher ventilation not due to 0 10 20 30 40 50 Prolonged Exercise in aPCO increased Hot Exercise time (min) Environment Figure 10.21Changes in ventilation and blood gas tensions Figure 10.21 illustrates the change in pulmonary ven- during prolonged, submaximal exercise in a hot/humid 28 tilation during prolonged, constant-load, submaximal environment. 232 Section One Physiology of Exercise pow23534_ch10_215-245.indd 232 24/07/14 3:08 PM Control of Ventilation at Rest Inspiration Produced by contraction of diaphragm Expiration CONFIRMING PAGES Produced by relaxation of diaphragm Q13 Respiratory muscles are controlled by somatic motor neurons in the spinal cord Controlled by respiratory control center In medulla oblongata and pons Midbrain Brain stem respiratory Pons Pneumotaxic center Caudal pons CONFIRMING PAGES centers Retrotrapezoid nucleus PreBötzinger complex Medulla oblongata Figure 10.23 Locations of the brain stem respiratory control centers. 29 stimuli reaching a specialized chemoreceptor. This re- ceptor reacts to the strength of the stimuli and sends the appropriate message to the medulla. A brief over- view of each of these receptors will be presented prior to a discussion of ventilatory control during exercise. bodies, and those found in the carotid artery are carotid bodies (Fig. 10.24). These peripheral che- moreceptors respond to increases in arterial H1 con- centrations and PCO2 (1, 4, 10, 49, 109, 116, 122). In addition, the carotid bodies are sensitive to increases in blood potassium levels and decreases in arterial 20 Humoral Chemoreceptors Chemoreceptors are specialized neurons that are capable of responding to PO2 (17, 49, 75). When comparing these two sets of peripheral chemoreceptors, it appears that the carotid changes in the internal environment. Traditionally, bodies are more important (5, 11, 16, 35, 49, 50–52, respiratory chemoreceptors are classified according to 67, 103, 105, 113, 115). their location as being either central chemoreceptors or peripheral chemoreceptors. Effect of Blood PCO2, PO2, and Potassium on Ventilation 15 Central Chemoreceptors The central chemore- ceptors are located in the medulla (anatomically sepa- How do the central and peripheral chemoreceptors rate from the respiratory center) and are affected by respond to changes in chemical stimuli? The effects V˙ E ( /min) changes in PCO2 and H1 of the cerebrospinal fluid (CSF). of increases in arterial PCO2 on minute ventilation is An increase in either PCO2 or H1 of the CSF results in shown in Fig. 10.25. Note that V̇E increases as a linear the central chemoreceptors sending afferent input into function of arterial PCO2. In general, a 1 mm Hg rise in the respiratory center to increase ventilation (35). PCO2 results in a 2 liter/minute increase in V̇E (35). The 10 Peripheral Chemoreceptors The primary pe- increase in V̇E that results from a rise in arterial PCO2 is likely due to CO2 stimulation of both the carotid bod- ripheral chemoreceptors are located in the aortic arch ies and the central chemoreceptors (35, 76). and at the bifurcation of the common carotid artery. In healthy individuals breathing at sea level, The receptors located in the aorta are called aortic changes in arterial PO2 have little effect on the control 5 www.mhhe.com/powers9e Chapter Ten Respiration During Exercise 235 pow23534_ch10_215-245.indd 235 24/07/14 3:08 PM 40 41 42 43 44 45 Arterial PCO2 (mm Hg) Figure 10.25 Changes in ventilation as a function Effect of Arterial PO 2 onarterial of increasing Ventilation PCO. Notice that ventilation 2 increases as a linear function of increasing PCO2. Sensory nerve fibers (in glossopharyngeal nerve) 30 Low PO2 stimulates the carotid bodies at the high Carotid altitude body 20 Q14 V˙ E ( /min) Carotid sinus “Hypoxic threshold” Hypoxic threshold: Sensory nerve fibers 10 Common carotid artery VE begins to(inrise vagusrapidly nerve) around an arterial PO2 of 60~75 mmHg | | | | | 0 0 20 40 60 80 100 Arterial PO2 (mm Hg) Aortic bodies Figure 10.26 Changes in ventilation as a function of decreasing arterial PO2. Note the existence of a “hypoxic Aorta threshold” for ventilation as arterial PO2 declines. 30 to as the hypoxic threshold (hypoxic means low PO2) (2). This hypoxic threshold usually occurs around an arterial PO2 of 60 to 75 mm Hg. The chemoreceptors Heart responsible for the increase in V̇E following exposure to low PO2 are the carotid bodies because the aor- tic and central chemoreceptors in humans do not re- spond to changes in PO2 (35, 67). Increases in blood levels of potassium can also stimulate the carotid bodies and promote an increase Figure 10.24 Illustration of the anatomical location of in ventilation (17, 75). Because blood potassium lev- the peripheral chemoreceptors (i.e., aortic bodies and carotid bodies). The aortic chemoreceptors respond to els rise during exercise due to a net potassium efflux from the contracting muscle, some investigators have 10 arterial changes in arterial H1 ion concentration (i.e., suggested that potassium may play a role in regulat- pH) and PCO2. The carotid bodies also respond to ing ventilation during exercise (17, 75). More will be arterial changes in arterial pH, PCO , and also changes Training Reduces the Ventilatory Response to Exercise Q15 No effect on lung structure Ventilation is lower during exercise following training Exercise ventilation is 20~30% lower at the same submaxima