Exam Notes - Amino Acids and Proteins PDF
Document Details
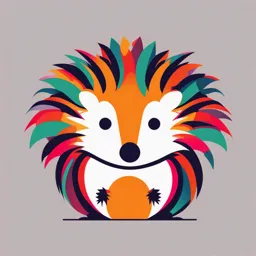
Uploaded by RefinedCaricature
Pomeranian Medical University in Szczecin
Emma
Tags
Summary
These notes cover amino acids and proteins, including their structure, classification, and roles in biological processes. Topics like sickle cell anemia, protein folding, collagen, and collagenopathies are explored. The document appears to be personal notes rather than a past paper.
Full Transcript
Emma notes 1. Amino acids and proteins Nonpolar side chains (hydrophobic) Uncharged (hydrophilic) Acidic Basic 1 Emma notes Structure Each amino acids contains - 1 carboxyl group (COO-) - 1 primary amino group (NH3) à except proline - 1 distinctive side chain (R group) Classified based on properties...
Emma notes 1. Amino acids and proteins Nonpolar side chains (hydrophobic) Uncharged (hydrophilic) Acidic Basic 1 Emma notes Structure Each amino acids contains - 1 carboxyl group (COO-) - 1 primary amino group (NH3) à except proline - 1 distinctive side chain (R group) Classified based on properties of their side chains à polar or nonpolar Nonpolar - In protein found in aqueous solutions, on interior surface - Also found on surface of proteins that interact with lipids (membrane proteins) - Hydrophobic - Proline § Differs from other AAs § Side chain and amino N form a rigid, five – membraned ring structure § A “Secondary” amino groups, instead of primary § Contributes to the fibrous structure of collagen § Often interrupts alpha – helices in globular proteins Uncharged - 0 net charge at physiologic pH - Cysteine and tyrosine can lose a proton in alkaline pH - Serine, threonine, and tyrosine each contain polar hydroxyl group à participate in hydrogen bond formation - Asparagine and glutamine contain carbonyl group and amide group à participate in hydrogen bond formation - Cysteine § Contains sulfhydryl group (- SH) § An important component of active site of many enzymes § Cysteine can be oxidized to form disulphide bonds (S – S) § Albumin is stabilized by disulphide bonds, and other extracellular proteins - Serine, threonine, and rarely tyrosine § Hydroxyl group is attachment site for phosphate Acidic - Glutamate and aspartate § Proton donors § Fully ionized at physiological pH § Contains negatively charged carboxylate group (- COO-) Basic - Accept donors - Lysine and arginine § Fully ionized and positively charged at physiological pH - Histidine § Weakly basic § Free, and largely unchanged in physiological pH § Buffering role in proteins, e.g., hemoglobin § Only AA with side chain that can ionize within physiologic pH range Uncharged, acidic, and basic are all found on outside of proteins that function in aqueous environment and interior of membrane – associated proteins Drug absorption At pH of stomach (1,5) at drug like aspirin (pH 3.5) will be largely pronated (COOH) and uncharged Uncharged drugs generally cross membranes more rapidly than do charged molecules 2 Emma notes Sickle cell anemia Sickling disease of RBCs Results from replacement of polar glutamate with nonpolar valine at 6th position of Beta subunit of hemoglobin Isomers All AAs found in mammalian proteins are of L configuration D – amino acids are found in some antibiotics and bacterial cell walls Racemases enzymatically interconverts the D – and L – isomers of free AAs Structure of proteins 20 AAs found in proteins are joined together by proteins Divided into 4 levels Primary structure - Sequence of amino acids - Peptide bonds § Amide linkages between carboxyl group and amino group of AAs § Resident to conditions that denature proteins à heating and high concentrations of urea § Characteristics; partial double – bond (shorter than a single bond), rigid and planar, trans configuration, and uncharged but polar - Cleavage of polypeptide § Many polypeptide have a primary structure of more than 100 AAs § These molecules cannot be sequenced directly from end to end § But they can be cleaved at specific sites à using cleaving agents § Enzymes that hydrolyze peptide bonds à peptidases (proteases) Secondary structure - Alpha – helix § Tightly packed, coiled polypeptide backbone with side chains of AAs, extended outward § Very diverse group of proteins contains helices, e.g., keratins, fibrous protein à in hair, skin § Myoglobin, also highly helical but globular protein § Stabilized by hydrogen bonds à individually weak but “strong” together § Each turn contains 3,6 AAs § Proline disrupts by inserting kink in chain § Large number of charged AAs (glutamate, aspartate, histidine, lysine, and arginine) disrupt by forming ionic bonds or by electrostatically repel each other § Glycine is also helix breaker § Glutamate and tryptophan, and valine have low helix propensity - Beta – sheet § All peptide bonds components are involved in hydrogen bonding § Composed of 2 or more polypeptide chains/segments § Or from segments of polypeptide chains that are arranged either antiparallel or parallel to each other § Also be formed by single polypeptide chain folding back on itself - Alpha – helix and beta – sheet structures provide maximal hydrogen bonding for peptide bond components within the interior of polypeptides - Beta bends 3 Emma notes Reverse the direction of polypeptide chain à helping it form compact, globular shape § Usually found in surface of protein molecules and often include charged residues § Generally composed of 4 AAs à one which may be proline § Glycine also frequently found in bends § Stabilized by formation of hydrogen and ionic bonds - Motifs/super secondary structures § Beta – meander § Beta – barrel § May be associated with particular functions à proteins that bind to DNA Tertiary structure - Domains § Fundamental functional and 3D structural units of polypeptides § Polypeptide with > 200 AAs in length = 2 or more domains § Core is built of motifs - Interactions § Disulphide bonds (- SH) § Hydrophobic interactions § Hydrogen bonds § Ionic interactions (- COO-) and (NH3+) Quaternary structure - Proteins may consist of 2 or more polypeptides that may be structurally identical or totally unrelated - Subunits are held together by noncovalent interactions § Hydrogen bonds § Ionic bonds § Hydrophobic interactions - Subunits may work independently or together (as in hemoglobin) § Protein folding Interactions between side chains of AAs determine how a long polypeptide chain folds into 3D shape of a functional protein Occurs within seconds to minutes Denaturation of proteins Unfolding and disorganization of a proteins secondary and tertiary structures à without hydrolysis of peptide bonds Denaturation agents include - Heat - Organic solvents - Strong acids and bases - Detergents - Heavy metals (e.g., lead) It may be reversible, under ideal conditions Denatured proteins are often insoluble and precipitate from solution Chaperons Specialized group of proteins Also called heart shock proteins (HSP) - Interact with polypeptide at various stages during folding Some chaperons bind hydrophobic regions of an extended polypeptide 4 Emma notes - Are important for keeping the protein unfolded until its synthesis is completed - Ex. Hsp70 Others form cage – like macromolecular structures composed of 2 stacked rings - Enters cage, binds central cavity through hydrophobic interactions, folds and is released - Ex. Hsp60 Protein misfolding Misfolded proteins are usually tagged and degraded within cell The quality control system is not perfect - Intracellular/extracellular aggregates of misfolded proteins accumulate à particularly as individuals age Misfolded proteins are associated with a number of diseases Amyloid disease - Accumulation of insoluble fibrous proteins à amyloids - Has been implicated in Parkinson and Alzheimer’s disease - Alzheimer´s § Amyloid beta accumulates § Extracellular peptide containing 40 – 42 AA residues § Other biological factor is accumulation of neurofibrillary tangles instead neurons à key component is tau protein § Defective tau blocks the actions of its normal counterpart - Parkinson’s § Amyloid is formed from alpha – synuclein protein Prion (proteinaceous infectious particles) diseases - Prion protein (PrP) is causative agents of transmissible spongiform encephalopathies (TSE) § Creutzfeldt – Jacob disease in humans § Scrapie’s in sheep § Bovine spongiform encephalopathy in cattle 1. Interaction with infectious PrP (contains beta – sheets) molecule with normal PrP (contains alpha – helix) causes the normal form to fold into the infectious form 2. These molecules dissociate and converts 2 additional non – infectious PrP molecules to the infectious form 3. This result in an exponential increase of the infectious form 5 Emma notes 2. Fibrous proteins Collagen The most abundant protein in human body Long, rigid structure where 3 polypeptides are wounded around each other Tough fiber with high tensile strength Types - Fibril – forming § Rope like structure - Network – forming § Sheet or meshwork - Fibril – associated § Bind to surface of collagen fibrils à linking them Type Tissue distribution Fibril – forming I Skin Bone Tendon Blood vessels Cornea II Cartilage Intervertebral disk Vitreous body III Blood vessels Skin Muscle Network – forming IV Basement membrane VIII Corneal and vascular endothelium Fibril associated IX Cartilage XII Tendon Ligaments Some other tissues Structure - Elongated, triple – helical structure - Stabilized by interchain hydrogen bonds - AA sequence § Proline à ring structure causes “kinks” in peptide chain à facilitates formation of helical confirmation of alpha chain (no helix) § Glycine à found every 3rd position of peptide chain § Gly – X – Y à X is often proline and Y often hydroxyproline - Hydroxyproline and hydroxylysine § Nonstandard AAs in collagen, not present in most proteins § Result from hydroxylation of lysine and proline residues after incorporation into polypeptide chains § It’s a posttranslational modification - Glycosylation 6 Emma notes § Hydroxyl (OH) group of hydroxylysine residues may be enzymatically glycosylated Biosynthesis of collagen 1. Genes of pro – alpha 1 and pro – alpha 2 chains are transcribed into mRNAs 2. mRNA is translated on the RER into prepro – alpha chains that are extruded into the lumen à where N – terminal signal sequence is removed, converting prepro to pro 3. Selected proline and lysine residues are hydroxylated 4. Selected hydroxylysine residues are glycosylated with glucose and galactose 5. Three pro alpha – chains assemble, intrachain and interchain disulphide bonds form at C – terminal propeptides extension 6. A triple helix is formed, and procollagen is produced 7. The procollagen is secreted from a golgi vacuole into the extracellular matrix 8. The N – terminal and C – terminal propeptides are cleaved by procollagen peptidases producing tropocollagen 9. Self – assembly of tropocollagen molecules into fibrils, with subsequent cross – linking, forms mature collagen fibers Degradation of collagen Collagen has half – life for as long as several years, highly stable Dynamic and responds to growth and injury Breakdown is dependent on of proteolytic action of collagenases Cleavage site for type 1 is specific, generating ¾ and ¼ length fragments à fragments are further degraded by other matrix proteinases Collagenopathies Ehlers – Danlos syndrome - Group of CT disorders that result from hereditable defects in metabolism of fibrous collagen - Can be caused by deficiency of collagen – processing enzymes à e.g., lysyl hydroxylase or N – procollagen peptidase - Or from mutations in AA sequence of collagen types I, III, and V - Classic form of EDS § Caused by defects in type V § AR inheritance § Characterized by skin extensibility and fragility, and joint hypermobility - Vascular form of EDS § Caused by defects in type III § AR inheritance § Most serious form of EDS à associated with lethal arterial rapture Osteogenesis imperfecta (OI) - “Brittle bones disease” à genetic disorder of bone fragility - Characterized by bones that break easily, with minor or no trauma - 80% + cases of OI are caused by dominant mutations of genes that encode alpha1 or alpha2 chains in type I collagen - Most common is replacement of glycine in Gly – x – y chain by AAs with bulky side chains - Type 1 § Most common § Characterized by mild bone fragility, hearing loss, and blue sclera - Type 2 § Most severe § Typically, lethal in perinatal period as a result of pulmonary complications 7 Emma notes - - Elastin Type 3 § Also, severe § Characterized by multiple fractures at birth, short fracture, spinal curvature leading to humped – back appearance, and blue sclera Scurvy § Vitamin C deficiency § Needed for hydroxylation of proline and lysine § Results in collagen with decreased tensile strength CT with rubber – like properties Elastic fibers are composed of elastin and glycoprotein microfibrils Found in walls of large arteries, and elastic ligaments Can be stretched to several times their normal length Structure - Insoluble protein polymer generated from tropoelastin - Rich in glycine, alanine, valine, proline, and lysine à little hydroxylysine and hydroxyproline - 3 allysyl side chains + 1 unaltered lysyl side chain from same of neighbouring polypeptide à form desmosine cross – link - Marfan syndrome § Mutations in fibril – 1 protein § Characterized by impaired structural integrity in skeleton, eye, and cardiovascular system § Abnormal fibrillin protein is incorporated into microfibrils along normal fibrillin à inhibiting formation of functional microfibrils Alpha1 – antitrypsin (AAT) Blood and other body fluid contains this protein Synthesized and secreted by liver Normally inhibits a number of proteolytic enzymes In lungs - Normally inhibits elastase released during phagocytosis by neutrophils present in alveoli of lungs - A deficiency of a1 – antitrypsin permits neutrophils elastase to destroy lung Mutations in AAT can lead to emphysema (lungs) and cirrhosis (lungs) à smoking increases risk 8 Emma notes 3. Enzymes Virtually all reactions in body are mediated by enzymes à protein catalyst that increases the rate of reactions without being changed in process Enzymes direct all metabolic events Nomenclature Assigned into 2 names - Short and easy - Complicated Recommended name - Uses enzymes names that ends with “ase” on the substrate of reaction à e.g., glucosidase and urease - Or a description of the action performed à lactate dehydrogenase and adenylyl cyclase Systemic name - Divided into 7 major groups 1. Oxidoreductase Catalyse oxidation – reduction reaction Transfer of H and O atoms or electrons from one substance to another 2. Transferases 3. Hydrolases 4. Lyases 5. Isomerases 6. Ligases Catalyse transfer of functional group from on substance to another Group may be methyl, acyl, amino or phosphate group Ex. Kinase and phosphorylase Catalyse formation of 2 products from a substrate by hydrolysis Catalyse non – hydrolytic addition or removal of groups from substrates C – C, C – O, C – S or C – N may be cleaved Catalyse intramolecular rearrangement of atoms Joint together 2 molecules by synthesis of new C – O, C – N or C – C with simultaneous breakdown of ATP 9 Emma notes 7. Translocases Catalyse movement of ions or molecules across membranes or their separation within membranes Potential confusing nomenclature Synthase à requires NO ATP Synthetase à ATP required Phosphatase à uses water to remove a phosphate group à a hydrolase Phosphorylase à uses inorganic phosphate (Pi) to break a bond and generate a phosphorylated product Dehydrogenase (NAD+/FAD) à an electron acceptor in redox reaction Oxidase à oxygen is acceptor and oxygen atoms are not incorporated into substrate H2O or H2O2 as products Oxygenase à one or both oxygen atoms are incorporated Properties Active site - A special pocket or cleft à formed by folding of protein, contains AA side chains that participate in substrate binding and catalysis - Bind enzyme noncovalently à forming enzyme – substrate (ES) complex Efficiency - Highly efficient Specificity - Reaction specificity § Enzyme catalyse only one type of reaction: § Transferase catalyse transfer of a functional group from one substrate to another - Bond specificity § Enzymes acts on substrates that are similar in structure and contain same type of bonds § Lipase – hydrolyses ester bonds in different TAGs § Amylase – hydrolyse alpha 1,4 glycosidic bonds in dextrins, starch, and glycogen - Substrate specificity § Enzymes acts only on one substrate § Arginase acts only in arginine § Lactase acts only on lactate § Sucrase acts only on sucrose Holoenzymes, apoenzymes, cofactors, and coenzymes - Holoenzyme à active enzyme with nonprotein component - Apoenzyme à inactive without nonprotein component - Cofactor à if nonprotein is metal ion (zinc or iron) - Cosubstrates à coenzyme that only transiently associate with the enzyme - Coenzyme à if nonprotein is a small morganic molecules à commonly derived from vitamins 10 Emma notes Prosthetic group à when the coenzyme is permanently associated with the enzyme and returned to its original form § Ex. NAD+ from niacin and FAD from riboflavin Enzymes can be regulated à increased or decreased Location - Intracellular (non-functional plasma enzymes) § Mitochondria à TCA, FA oxidation § Cytosol à glycolysis, PPP, FA synthesis § Nucleus à DNA and RNA synthesis § Lysosome à degradation - Extracellular § Secretory enzymes (functional plasma enzymes) § Excretory enzymes (non – functional plasma enzymes) Factors effecting reaction velocity Substrate concentration - The rate increases with the substrate concentration until it reaches maximal velocity (Vmax) Temperature - Velocity increases with the temperature - Velocity decreases with higher temperature à start to denature at 40+ degrees - Optimal temperature for human enzymes is 35 – 40 degree Celsius pH - Effects the active site on ionization à amino group is depronated in alkaline pH à rate declines - Extreme pH can lead to denaturation of enzyme - Enzymes have different optimal pH à some acidic and some alkaline Michaelis – Menten kinetics An equation that describes how reaction velocity varies with substrate concentration !"#$ [$] !! = K " + [S] Vo = initial velocity Vmax = maximal velocity Km = Michaelis constant [S] = substrate concentration The substrate concentration is much higher than enzyme concentration Km characteristics - Small Km reflects high affinity of the enzyme for substrate - High Km reflects low affinity of enzyme for substrate The rate of reaction is directly proportional to enzyme concentration because [S] is not limiting When [S] is > than Km à velocity of reaction is constant and equal to Vmax Enzyme inhibition Any substrate that can decrease velocity of reaction Can be reversible or irreversible Irreversible bind to enzymes through covalent bonds - E.g., lead forms covalent bonds with sulfhydryl side chain of cysteine Reversible bind to enzymes through noncovalent bonds à 2 types, competitive and non – competitive 11 Emma notes - - Competitive inhibition § When inhibitor binds reversibly to the same site that the substrate normally would occupy § Vmax is unchanged in presence competitive inhibitor § Km is increased in the presence of competitive inhibitor § Statin drugs is an example (atorvastatin, pravastatin) à inhibits rate – limiting step in cholesterol synthesis à inhibits HMG CoA reductase à lower plasma cholesterol levels Non – competitive inhibition § Occurs when inhibitor and substrate bind at different sites on enzyme § Can bind either free enzyme or enzyme – substrate complex à preventing reaction from occurring § Vmax is decreased in presence of non – competitive inhibitor § Km is unchanged in presence of non – competitive inhibitor § Antibiotics are non – competitive inhibitor à amoxicillin and penicillin inhibit enzymes involves in bacterial cell wall synthesis à aspirin inhibits cyclooxygenase à prostaglandin and thromboxane synthesis Enzyme regulation Allosteric enzyme - Regulated by molecules called effector that binds non – covalently at site other than active site - Can be negative effector or positive effectors - Allosteric enzymes often catalyse the rate – limiting step - Homotropic effectors § When substrate itself severs as an effector - Heterotopic effectors § Effector may be different from substrate § E.g., PFK – 1 is allosterically inhibited by citrate à which is not a substrate for the enzyme Covalent modification - Many enzymes are regulated this way à most often by removal/addition of phosphate group from specific serine, threonine, or tyrosine residue of enzyme - Phosphorylation/dephosphorylation § Catalysed by protein kinases § Use ATP as phosphate donor § Phosphate groups are cleaved from phosphorylated enzyme by phosphoprotein phosphatases § Phosphorylation/dephosphorylation can activate or inactivate enzyme à depending on the enzyme Isoenzymes Enzymes that catalyse same reaction à but not the same physical properties They have genetically differences in AA sequence Diagnostic value Increased plasma levels of certain intracellular enzymes may indicate tissue damage Alanine transferase (ALT) and aspartate transferase (AST) is abundant in plasma when there is possible liver damage Amylase (pancreatitis), lipase, alkaline phosphate can be used in diagnostics Creatine kinase 12 Emma notes - Isoenzymes CK1 = BB à all CK in the brain CK2 = MB à 1/3 is cardiac, rest skeletal muscle CK3=MM à skeletal mm Myocardial infraction (MI) § CK2 will appear in plasma within 4 – 8 hours following acute MI § Can be used together with elevated cTn (cardiac specific isoforms of troponin T) and ECG to diagnose MI 4. Nucleotide metabolism Purines Adenine Guanine Xanthine Caffeine Pyrimidines Thymine Cytosine Uracil (only RNA) Nucleotides is important for: DNA and RNA production Carrying activated intermediates of synthesis of carbohydrates, lipids and conjugated proteins Structural components of essential coenzymes (CoA, FADH2, NADH, NADPH) Second messengers in signal transduction pathways (cAMP and cGMP) Energy source for the cell Regulatory compounds for intermediary metabolism pathways, inhibiting or activating key enzymes Structure Purines – adenine, guanine Pyrimidines – thymine (methylated), cytosine, uracil N-glycosidic bond – between base and pentose sugar Modifications – methylation, glycosylation, acetylation, reduction First phosphate group is attached by ester linkage, second and third by high-energy bonds - Phosphate groups are responsible for negative charges of nucleotides Purine synthesis Atom sources – glutamine, aspartate, glycine, CO2, N10-formyl-THF Synthesized in liver 5-Phosphoribosyl-1-pyrophosphate (PRPP) – synthesized from ATP and ribose 5-phosphate by PRPP synthetase (activated by Pi, inhibited by purine ribonucleotides) Committed step: 5-Phosphoribosylamine synthesis 13 Emma notes By glutamine:phosphoribosylpyrophosphate amidotransferase (GPAT) – activated by PRPP, inhibited by AMP, GMP The next nine steps lead to synthesis of IMP (base is hypoxanthine) - Four steps require ATP, two steps require N10-formyl-THF - Enzymes: GAR synthetase, GAR formyltransferase, FGAM synthetase, AIR synthetase, AIR carboxylase, SAICAR synthetase, adenylosuccinate lyase, AICAR formyltransferase, cyclohydrolase Structural analogs of PABA (sulfonamides) and folic acid (methotrexate) are used to inhibit purine synthesis - Extremely toxic to tissues, especially to developing structures like fetus, or cells that replicate rapidly like bone marrow, skin, GI tract, immune system and hair follicles IMP is converted to AMP or GMP in a two-step, energy- and nitrogen-requiring pathway - AMP requires GTP and aspartate, enzymes – adenylosuccinate synthetase, adenylosuccinate lyase - GMP requires ATP and glutamine, enzymes – IMP dehydrogenase, GMP synthetase - Both reactions are inhibited by their product Base-specific nucleotide monophosphate kinases converts monophosphates to diphosphates. Nucleotide diphosphate kinase converts diphosphates to triphosphates - Both use ATP as energy source Purine salvage Purines from normal cell turnover or from diet that are not degraded can be converted to nucleoside triphosphates and used by the body Two enzymes involved: adenine phosphoribosyltransferase (APRT) and hypoxanthineguanine phosphoribosyltransferase (HGPRT) - Both use PRPP as source for ribose 5-phosphate - Hydrolysis of pyrophosphate by pyrophosphatase makes the reaction irreversible Lesch-Nyhan syndrome X-linked disorder caused by HGPRT deficiency – cause inability to salvage hypoxanthine and guanine, leads to overproduction of uric acid Increased PRPP levels, decreased IMP and GMP levels à increased de novo purine synthesis by GPAT Decreased degradation of purines, increased production of uric acid à hyperuricemia Formation of uric acid stones in kidneys (urolithiasis), deposits of urate crystals in joints (gouty arthritis) and soft tissues Motor dysfunction, cognitive deficits and behavioral disturbances like self-mutilation Deoxyribonucleotide synthesis Deoxyribonucleotides are produced ribonucleoside diphosphates by ribonucleotide reductase (activated by ATP, inhibited by dATP) Hydrogen donors are two -SH groups on the enzyme, which form a disulfide bond - Disulfide bond is reduced to continue production (on R1) by thioredoxin 14 Emma notes - Oxidized thioredoxin is converted back to reduced form by NADPH + H+ and thioredoxin reductase Ribonucleotide reductase Contains two nonidentical subunits (R1 and R2) Responsible for balanced supply of deoxyribonucleotides required for DNA synthesis Two allosteric sites in addition to catalytic site: - Activity site – binding of dATP, inhibits catalytic activity and prevents reduction of ribonucleoside diphosphates, thus prevent synthesis of DNA. ATP bound to activity site activates the enzyme - Substrate specificity site – binding of nucleoside triphosphates regulates substrate specificity, causing increased conversion of ribonucleotides to deoxyribonucleotides when needed for DNA synthesis. Binding of ex. dTTP cause change that allows reduction of GDP to dGDP at catalytic site when ATP is at activity site Ribonucleotide reductase is inhibited by hydroxyurea (used as cancer treatment and in sickle cell anemia) – inhibits generation of substrates for DNA synthesis Purine degradation Ribonucleases and deoxyribonucleases hydrolyze DNA and RNA to oligonucleotides, which are hydrolyzed to 3´- and 5´-mononucleotides by phosphodiesterases Nucleotidases removes phosphate groups, releasing nucleosides that are degraded by nucleosidases to free bases and (deoxy)ribose 1-phosphate Dietary purines are degraded to uric acid Uric acid formation 1. An amino group is removed from AMP to produce IMP by AMP deaminase or from adenosine to produce inosine by adenosine deaminase 2. IMP and GMP are converted into their respective nucleoside forms, inosine and guanosine, by 5´-nucleotidase 3. Purine nucleoside phosphorylase converts inosine and guanosine into their respective purine bases, hypoxanthine and guanine 4. Guanine is deaminated to form xanthine 5. Hypoxanthine is oxidized by molybdenum-containing xanthine oxidase to xanthine, which is further oxidized by XO to uric acid, the final product of human purine degradation (excreted in urine) Gout Disorder initiated by high uric acid levels in blood, caused by overproduction or underexcretion of uric acid Monosodium urate crystals can be disposed in joints à inflammatory response cause first acute and then chronic gouty arthritis Nodular masses of MSU crystals (tophi) may be deposed in soft tissues, resulting in chronic tophaceous gout. Kidney stones (urolithiasis) may also be seen 15 Emma notes Gout is always preceded by hyperuricemia Diagnosis – examination of synovial fluid from affected joint or material from tophus Treatment – anti-inflammatory agents (colchicine, steroidal and non-steroidal drugs), lowering uric acid level (below 6,5 mg/dl), increase renal secretion of uric acid (uricosuric agents like probenecid and sulfinpyrazone), allopurinol (structural analog of hypoxanthine, inhibits uric acid synthesis) Increased risk – diet rich in meat, seafood and ethanol, decreased risk – diet rich in low-fat dairy products Uric acid underexcretion > 90% of hyperuricemia cases Inherent excretory effects, defects in how kidneys handle urate, or environmental factors (drugs or exposure to lead) Uric acid overproduction Less common cause of hyperuricemia Mostly unknown cause, but also mutations in PRPP synthetase (increased Vmax, lower Km for ribose 5-phosphate or decreased sensitivity to purine nucleotides – its allosteric inhibitor) Secondary hyperuricemia – increased availability of purines Adenosine deaminase deficiency Results in accumulation of adenosine – converted to (deoxy)ribonucleotide forms by kinases Higher dATP levels inhibit ribonucleotide reductase, which prevents production of deoxyribonucleotides à cells cannot make DNA and divide Can cause SCID, involving decrease in T cells, B cells and NK cells Treatment – bone marrow transplantation, enzyme replacement therapy and gene therapy Without treatment, children usually die from infection by age 2 years Purine nucleoside phosphorylase deficiency Rarer and less severe than ADA deficiency Results in a less severe immunodeficiency, primarily involving T cells PNP-deficient individuals have recurrent infections and neurodevelopmental delay Pyrimidine synthesis Regulated step: Carbamoyl phosphate synthesis from glutamine and CO2 by CPS II (activated by PRPP, inhibited by UTP) Carbamoyl aspartate is formed by aspartate transcarbamoylase, then the pyrimidine ring is closed by dihydroorotase - CAD – single, multifunctional polypeptide consisting of CPS II, Aspartate transcarbamoylase and Dihydroorotase, Dihydroorotate is oxidized to orotic acid (orotate) by dihydroorotate dehydrogenase (the only mitochondrial enzyme in pyrimidine synthesis) The orotate ring is attached to ribose 5-phosphate (PRPP is donor) to form OMP by orotate phosphoribosyltransferase, releasing PPi (making reaction irreversible) 16 Emma notes OMP is decarboxylated to UMP by orotidylate (OMP) decarboxylase Orotate phosphoribosyltransferase and OMP decarboxylase make up the single polypeptide UMP synthase - Orotic aciduria – very rare disorder, caused by deficiency of one or both activities of this bifunctional enzyme, resulting in orotic acid in urine UMP is phosphorylated to UDP and UTP - UDP is substrate for ribonucleotide reductase, which generates dUDP à phosphorylated to dUTP à hydrolyzed to dUMP by UTP diphosphatase CTP is formed by amination of UTP (glutamine providing nitrogen) by CTP synthetase CTP is dephosphorylated to CDP – substrate for ribonucleotide reductase, produce dCDP, which can be phosphorylated to dCTP for DNA synthesis or dephosphorylates to dCMP dUMP is converted to dTMP by thymidylate synthase (inhibited by thymine analogs, 5fluorouracil – suicide inhibitor) - Methyl group source is N5-N10-methylene-THF Pyrimidine salvage and degradation Pyrimidine ring is opened and degraded to soluble products ()-alanine and )aminoisobutyrate), with production of ammonia and CO2 Bases can be salvaged to nucleosides, and be phosphorylated to nucleotides Salvage of pyrimidines is less clinically significant than purine salvage Variable Cellular location Pathway involved Source of nitrogen Regulators CPS I Mitochondria Urea cycle Ammonia Activator: N-acetylglutamate CPS II Cytosol Pyrimidine synthesis *-Amide group of glutamines Activator: PRPP, inhibitor: UTP 17 Emma notes 5. DNA Structure Structure DNA is present in chromosomes and mitochondria, and contains genetic information - Eukaryotic: in nucleus - Prokaryotic: in nucleoid Consist of phosphate group, deoxyribose molecule and bases – dNMPs covalently linked by 3´→ 5´-phosphodiester bonds Double-stranded molecule as a double helix. Chains are coiled around a helical axis in an antiparallel manner. Hydrophobic bases are perpendicular to the axis The two strands create grooves (major and minor) that provides access for binding of regulatory proteins Bases are paired: A-T (two H-bonds), G-C (three H-bonds) Hydrophobic interactions – between stacked bases in the double helix Van der Waals linkages – hold the two strands together Chargaff rule In any sample of dsDNA, the amount of A equals to the amount of T, and the amount of G equals the amount of C The total amount of purines (A+G) equals the total amount of pyrimidines (T+C) Melting temperature In the laboratory, dsDNA can be separated by altered pH (ionize bonds) or heating of solution - Phosphodiester bonds are not broken by this, but by enzymatic or chemical hydrolysis Melting temperature (Tm) – where half of the helix is lost (denaturation) Denaturation can be monitored by measuring its absorbance at 260 nm - ssDNA has higher relative absorbance than dsDNA Structural forms of DNA B form: right-handed helix, 10 bp per 360° turn. Planes of bases are perpendicular to the helical axis. Found in chromosomal DNA A form: produced by hydrating B form. Right-handed helix, 11 bp per turn. Planes of bases are tilted 20° away from the perpendicular to the helix. Found in DNA-RNA hybrids and RNARNA double stranded regions Z form: left-handed helix, 12 bp per turn. Named by the zigzags of the backbone. Found in DNA regions with alternating purines and pyrimidines 18 Emma notes Supercoiling Double helix crosses over on itself one or more times – can result in overwinding (positive supercoiling) or underwinding (negative supercoiling) Tertiary structure which compacts DNA Positive supercoils appear in front of the replication fork when the double helix is separated. Negative supercoils appear behind the form DNA topoisomerases removes supercoils by transiently cleaving one or both of the DNA strands - Type I – reversibly cleave one strand. Use energy from phosphodiester bond. A transient nick is created on one strand and the other strand passes through the break, thus relaxing accumulated supercoils - Type II – make transient breaks in both strands, then causes a second stretch to pass through the break, before resealing it. ATP-requiring process, can relieve both positive and negative supercoils - DNA gyrase – introduce negative supercoils to neutralize the positive supercoils introduced during opening of the double helix. Requires energy from ATP Anticancer agents, such as camptothecins, target human type I topoisomerases, whereas etoposide target human type II topoisomerases. Bacterial DNA gyrase is a unique target of a group of antimicrobial agents called fluoroquinolones (ex. ciprofloxacin) Factors participating in replication DNA polymerase – synthesize complementary sequence of each DNA strand. Requires Mg2+. Also has proofreading activity DnaA protein – initiates replication by binding to specific nucleotide sequences within oriC, causes AT-rich region to melt DNA helicase – unwinds the double helix. Require energy from ATP hydrolysis SSB protein – keep the two ssDNAs separated, also protects DNA from degrading nucleases DNA topoisomerases – removes supercoils in the helix by transiently cleaving one or both of the DNA strands RNA primer – pairs to the DNA strand and serves as the first acceptor of a deoxynucleotide DNA ligase – joining of the last two stretches of DNA, requires energy from ATP Replication fork formation DnaA protein binds to DnaA boxes at the origin of replication, and results in a short region of ssDNA As the two strands unwind and separates, two replication forks appear and move away from the origin in opposite directions, generating a replication bubble Okazaki fragments DNA polymerase can only synthesize in 5´→ 3´ directions 19 Emma notes The strand that is copied away from the replication fork is synthesized discontinuously (lagging strand), with small fragments of DNA being copied near the replication fork – these are called okazaki fragments. They are joined together by ligase to a continuous strand The strand that is being copied continuously in the direction of the advancing replication fork is called the leading strand Activity of exonucleases 3´→ 5´ exonucleases (activity of DNA pol III) proofread newly added nucleotides and removes errors in the opposite direction to polymerization. Correct nucleotides are then added by 5´→ 3´ polymerases 5´→ 3´ exonucleases (DNA pol I activity) hydrolytically remove the RNA primer. DNA pol I replaces the ribonucleotides with deoxyribonucleotides Eukaryotic replication Long linear DNA – multiple origins of replication Origin recognition proteins, SSB proteins and ATP-dependent helicases is similar as in prokaryotic replication RNA primers are removed by RNase H and flap endonuclease 1 (FEN1) rather than by DNA pol Eukaryotic DNA pols Pol , – initiates DNA synthesis, contains primase. No proofreading activity Pol ) – DNA repair. No proofreading activity Pol - – elongates Okazaki fragments on lagging strand Pol. – elongates leading strand Pol * – replicates mitochondrial DNA Telomeres Complexes (DNA + proteins) on the chromosome ends They prevent attacks by nucleases, and allow repair systems to distinguish a true end from a break Telomeric DNA – several thousand tandem repeats of a non-coding sequence (AGGGTT). Grich strand is longer than its C-rich complement Telomeres shorten with every cell division until they are too short to divide further – become senescent (except germ cells, stem cells and cancers) 20 Emma notes Telomeres may be viewed as mitotic clocks in that their length in most cells is inversely related to the number of times the cells have been divided. The study of telomeres provides insight into the biology of normal aging, diseases of premature aging, and cancer Telomerase Maintains telomeric length in germ cells, stem cells and cancer cells RNA template (Terc) base-pairs with G-rich telomeric DNA. Reverse transcriptase synthesizes DNA on already longer 3´-end Telomerase translocates newly synthesized end, then primase uses this template to synthesize RNA primer Reverse transcriptase RNA-directed DNA pols, involved in replication of retroviruses like HIV Reverse transcriptase uses viral RNA as template for 5´→3´ synthesis of DNA Also seen with transposons – transcribed to RNA, used as a template for DNA synthesis. DNA is randomly inserted into the genome Nucleoside analogs DNA chain growth can be blocked by analogs that have been modified on the sugar portion Cytarabine – used in anticancer therapy Vidarabine – antiviral agent Azidothymidine (AZT) – terminates DNA chain elongation Allopurinol – used in hyperuricemia and gout, inhibits purine synthesis and xanthine oxidase activity Histones and nucleosomes Five classes of histones: H1 (most tissue specific), H2A, H2B, H3 and H4 Histones are small, positively charged proteins (due to lysine and arginine), which form ionic bond with negatively charged DNA and help neutralize negatively charged phosphate groups in DNA N-terminal can be methylated, acetylated or phosphorylated – affect gene expression Octameric core: two molecules of each H2A, H2B, H3 and H4, surrounded by wounded dsDNA (prevent helical turn and negative supercoiling) Neighboring nucleosomes are joined by linker DNA (50 bp), to which H1 is bound Nucleofilament: tightly packed nucleosomes, coil shaped, often referred to as 30-nm fiber. Organized into loops anchored by a nuclear scaffold Parental nucleosomes are disassembled to allow access to DNA during replication DNA repair DNA can be damaged by environmental agents (chemicals or radiation) or by spontaneous alterations of bases If damage is not repaired, permanent mutations can result in deleterious effects, including loss of control over proliferation – leading to cancer 21 Emma notes Most repair systems involve recognition of damage, removal or excision of the damage, replacement or filling the gap, and ligation. They can remove one to tens of nucleotides Mismatch repair: - Mediated by Mut proteins, which identify mispaired nucleotides by recognizing the mismatched strand based on methylation - GATC sequences (found every thousand nucleotides) are methylated on adenine residue by DNA adenine methylase (DAM) - The methylation occurs on the parental strand after synthesis. This strand is assumed to be correct, and the daughter strand gets repaired - Endonuclease nicks the strand and mismatched nucleotides are removed by an exonuclease. Additional nucleotides at the 5´- and 3´-ends are also removed. The gap left is filled by a DNA pol (typically III), using the sister strand as a template. The 3´-hydroxyl of the newly synthesized DNA is joined to the 5´-phosphate of the remaining stretch of the original DNA strand by DNA ligase Mutation to proteins involved in MMR in humans is associated with hereditary nonpolyposis colorectal cancer (HNPCC), also known as Lynch syndrome. Although HNPCC confers an increased risk for developing colon cancer, only about 5% of all colon cancer is result of mutations in MMR Nucleotide excision repair - UV radiation can cause covalent joining of two adjacent pyrimidines, producing a dimer. These intrachain cross-links prevent DNA pol from replicating the DNA strand - Thymine dimers are excised by UvrABC proteins by nucleotide excision repair (NER) - UV-specific endonuclease (called uvrABC excinuclease) recognizes the bulky dimer and cleaved the damaged strand on both 5´- and 3´-side. The gap left is filled by DNA pol I and DNA ligase - Xeroderma pigmentosum – rare genetic disease, in which cells cannot repair damaged DNA, resulting in extensive accumulation of mutation and cancers. Caused by defects in any of several gene’s coding for XP proteins required for NER Base excision repair - DNA bases can be altered spontaneously (deamination of cytosine to uracil) or by deaminating or alkylating compounds. Bases can also be lost spontaneously à Nitrous acid deaminates cytosine and adenine to hypoxanthine, and guanine to xanthine) à Dimethyl sulfates alkylate adenine - Lesions involving base alterations or loss can be corrected by base excision repair (BER) - In BER, abnormal bases are recognized by specific DNA glycosylases that hydrolytically cleave them from the backbone. This leaves an apyrimidinic site (or apurinic), referred to as AP site - Specific AP endonucleases recognize that a base is missing and initiate the process of excision and gap filling by making an endonucleolytic cut just to the 5´-side of the AP site. A deoxyribose phosphate lyase removes the single, base-free, sugar phosphate residue. DNA pol I and DNA ligase complete the repair process 22 Emma notes Double-strand break repair - Ionizing radiation, chemotherapeutic agents (such as doxorubicin) and oxidative free radicals can cause double strand breaks in DNA - Due to both strands being damaged, there is no template for replacing missing nucleotides. Instead, they are repaired by either NHEJ (nonhomologous end joining) or HR (homologous recombination) - NHEJ – mediates recognition, processing and ligation of the ends of two DNA fragments. Some DNA is lost in the process – error prone and mutagenic mechanism, associated with predisposition to cancer and immunodeficiency syndromes - HR – uses enzymes that normally perform genetic recombination between homologous chromosomes during meiosis. Less error prone, because lost DNA is replaced using homologous DNA as a template. Occurs in late S and G2. Mutations to BRCA1 or BRCA2 is involved in HR 6. RNA structure Difference between DNA and RNA RNA is smaller than DNA, and contains ribose instead of deoxyribose and uracil instead of thymine RNA exists as single strands, capable of complex folding rRNA (80%) Exist as different species: 23S, 16S and 5S in prokaryotes, 28S, 18S, 5,8S and 5S in eukaryotes rRNA molecules are produced from a single pre-rRNA molecule (except eukaryotic 5S – synthesized by RNA pol III) Ribozymes are RNA with catalytic activity (ribonucleic acid enzymes), like rRNA in protein synthesis. They function as catalysts in reactions, like RNA splicing and peptide bond formation. Examples: RNase P, peptidyl transferase 23S rRNA tRNA (15%) The smallest of the three major RNA (4S) Has a high percentage of unusual bases (dihydrouracil, pseudouracil) and extensive intrachain base pairing à secondary (cloverleaf) and tertiary (folded) structure - Cloverleaf: D-loop, anticodon-loop, anticodon, variable loop, T/C loop, 5´- and 3´-ends (CCA) At least one type of tRNA or each of the 20 amino acids Each tRNA carries its specific amino acid on the 3´-end, to the site of protein synthesis, recognizes genetic code on mRNA and add the amino acid to the growing chain mRNA (5%) Consist of base sequences complementary to DNA, with untranslated regions at the 3´- and 5´-ends. Eukaryotic mRNA has a poly-A tail at 3´-end and a 7-methylguanosine cap at 5´-end mRNA carries genetic information from DNA for use in protein synthesis (out of nucleus and into cytosol in eukaryotes) Polycistronic – mRNA carries info from more than one gene, in prokaryotes 23 Emma notes Monocistronic – mRNA carries info from one gene, in eukaryotes Transcription unit The stretch from the promoter to the termination region on DNA. It encodes at least one gene The initial product of transcription by RNA pol is termed the primary transcript Prokaryotic RNA polymerase Magnesium-requiring, multisubunit enzyme Synthesizes all of RNA except short RNA primers Recognizes promoter and termination regions on DNA, and makes a complementary RNA copy of template strand RNA is complementary to DNA template (antisense, minus) and identical to the coding (sense, plus), with U replacing T For a given gene, only one DNA strand can serve as a template, determined by location of the promoter Holoenzyme Core enzyme + sigma factor makes up the holoenzyme, which is involved in prokaryotic RNA pol Core enzyme - five subunits – 2 ,, 1 ), 1 )´, 1 0: - Assembly of enzymes: ,, 0 - Template binding: )´ - 5´→ 3´ polymerase activity: ) 2 subunit (sigma factor) – enables RNA pol to recognize the promoter region Steps of prokaryotic transcription 1. Initiation: RNA pol binds to promoter, which contains consensus sequences à -35 Sequence (5´-TTGACA-3´): initial contact point for holoenzyme, a closed complex is formed à Pribnow box (5´-TATAAT-3´): site of unwinding of a short stretch of DNA. Converts closed complex to an open complex – transcription bubble 2. Elongation Local unwinding continues by polymerase Sigma factor is released, and the core enzyme move along the template strand. Short DNARNA hybrid helix is formed Nucleoside monophosphates are added to the growing chain and pyrophosphate is released Transcription is always in 5´→ 3´ directions. RNA pol don´t have a primer and 3´→ 5´ exonucleases for proofreading à higher error rate 3. Termination 3-Independent: 24 Emma notes - Requires that a sequence in the DNA template generates a sequence in RNA that is selfcomplementary - RNA fold back on itself, forming a GC-rich stem + a “hairpin” loop – has a string of Us on 3´-end, which facilitates separation of new RNA from DNA 3-Dependent: - Rho, hexameric ATPase with helicase activity, is required - Rho binds a C-rich rho utilization (rut) site near the 5´-end, and moves along the RNA until it reaches RNA pol paused at the termination site - The ATP-dependent helicase activity separates the RNA-DNA hybrid helix, causing the release of RNA Antibiotics Can inhibit RNA synthesis, thus prevent bacterial cell growth Rifampin (rifampicin) – binds to ) subunit of RNA pol, prevent chain growth beyond three nucleotides. Used in treatment of tuberculosis Dactinomycin (actinomycin D) – first antibiotic to find therapeutic application in tumor chemotherapy. It inserts between the DNA bases – inhibits initiation and elongation Eukaryotic transcription More complicated, involves separate polymerases for synthesis of rRNA, tRNA and mRNA Transcription factors (TR) are involved – bind to distinct sites within the core promoter, required for assembly of transcription initiation complex and determination of which genes are to be transcribed Chromatic structure For TF to recognize and bind to their specific DNA sequences, the chromatic structure in that region must be decondensed to allow access Euchromatic – decondensed chromatin, most actively transcribed genes are found here Heterochromatic – highly condensed, has inactive segments Chromatin remodeling – interconversion of eu- and heterochromatin - Covalent modification of histones: acetylation of lysine residue by histone acetyltransferases (HAT), eliminates positive charge on lysine, thereby decreasing interaction of histone with negatively charged DNA. Acetyl group is removed by histone deacetylases (HDAC), which restores positive charge à Acetyl CoA provides the acetyl group Eukaryotic RNA polymerases RNA pol I – synthesizes precursor of 28S, 18S and 5,8S rRNA in the nucleolus RNA pol II – synthesizes precursor of mRNA and certain small ncRNA (snoRNA, snRNA and miRNA) RNA pol III – synthesizes tRNA, 5S rRNA and some snRNA and snoRNA RNA pol II – promoters TATA box (Hogness): similar to Pribnow box. Found -25 upstream of transcription start site Inr (initiator), DPE (downstream promoter element): 25 Emma notes - Found on the same molecule of DNA as the transcribed gene à cis acting Serve as binding sites for general transcription factors General transcription factors Minimal requirements for recognition of promoter, recruitment of RNA pol II to the promoter, formation of preinitiation complex and initiation of transcription Encoded by different genes, synthesized in cytosol and diffuse to their action site à transacting TFIID, a GTF containing TATA-binding protein, recognize and bind the TATA box TFIIF brings polymerase to the promoter Helicase activity of TFIIH melts the DNA, and its kinase activity phosphorylates polymerase, allowing it to clear the promoter Regulatory elements and transcriptional activators CAAT, GC boxes – proximal regulatory elements close to the core promoter Enhancers – farther away from core promoter, distal regulatory elements Transcriptional activators – specific transcription factors (STF) - Binds regulatory elements, proximal to regulate frequency of initiation, and distal to mediate response to signals such as hormones and regulate which genes are expressed at a given point - STF have two binding domains, a DNA-binding domain and a transcription activation domain, which recruits GTF to the core promoter as well as coactivator proteins such as HAT Transcriptional activators bind DNA through a variety of motifs, such as the helix-loop-helix, zinc finger, and leucine zipper Enhancers Increase the rate of initiation by RNA pol II Typically, on the same chromosome as the transcripted gene, but can be located upstream or downstream of transcription site, close to or thousands of base pairs away from promoter, and occur on either strand of DNA Contains DNA sequences called response element that bind STF, which can interact with other TF bound to a promoter and with RNA pol II, thereby stimulating transcription Mediators also bind enhancers RNA pol II inhibitor ,- Amanitin – toxin from poisonous mushroom (Amanita phalloides, “death cap”), binds RNA pol II tightly and slows its movement, thereby inhibiting mRNA synthesis Posttranscriptional modifications rRNA: 26 Emma notes Pre-rRNA are cleaved by ribonucleases to yield intermediate-sized pieces of rRNA, and then further processed by exonucleases to produce the required RNA species tRNA: - Sequences of both ends are removed, and if present, an intron is removed from the anticodon loop by nucleases - Addition of -CCA on 3´-end by nucleotidyltransferase - Modification of some bases to unusual bases mRNA: - Addition of 7-methylguanosine cap on 5´-end by 5´→ 5´ triphosphate linkage, catalyzed by guanylyltransferase and guanine-7-methyltransferase à S-Adenosylmethionine is the source of methyl group à Creation requires removal of * phosphoryl group from the 5´-triphosphate of premRNA, then addition of GMP à Addition of cap stabilizes the mRNA and permits efficient initiation of translation - Addition of poly-A tail on 3´-end by polyadenylate polymerase, using ATP as a substrate à Chain of 40-250 adenylates at 3´-end of mRNA à Pre-mRNA is cleaved downstream of a consensus sequence, called polyadenylation signal sequence (AAUAAA), found near the 3´-end of RNA, and the poly-A tail is added to the new 3´-end à Tailing terminates eukaryotic transcription à Tails stabilize mRNA, facilitate its exit from the nucleus and aid in translation - Splicing – removal of introns (non-coding parts) and joining of exons. Done by spliceosomes. Splicing is facilitated by snRNA à Five snRNP bind to primary transcript, U1 to 5´-donor site, U2 to branch A and 3´acceptor site, U4-U6 completes the complex. U1 and U4 leaves à The 2´-OH of the branch site A attacks the 5´-phosphate at the splice-donor site of the intron, forming a 2´→ 5-phosphodiester bond and a lariat à The free 3´-OH of exon 1 then attacks the 5´-phosphate at the splice-acceptor site, forming a phosphodiester bond that joins exons 1 and 2 - Alternative splicing – produce isoforms of a protein Function of snRNA Small, non-coding RNA in nucleus Synthesized by RNA pol II and III Uracil-rich snRNA forms five small nuclear ribonucleoprotein particles (snRNP/”snurp”) – U1, U2, U4, U5 and U6, which mediate splicing - Facilitate removal of introns – form base pairs with consensus sequences at each end of the intron In systemic lupus erythematosus, patients produce antibodies against their own nuclear proteins such as snRNP 27 Emma notes 7. Regulation of gene expression Regulation of genes Gene expression results in the production of a functional gene product (RNA or protein). Genes can be constitutive (housekeeping genes) or regulated (expressed under certain conditions in all cells or in a subset of cells) The ability to appropriately induce (positively regulate) or repress (negatively regulate) genes is essential in all organisms Regulation occurs primarily at transcription in both prokaryotes and eukaryotes and is mediated through trans-acting proteins binding to cis-acting regulatory DNA elements Operon A set of genes, as well as the regulatory DNA sequences for those genes – the promoter (RNA pol) and operator (repressor) Found only in prokaryotes, coordinate regulation of genes whose protein products are required for a particular process Lac operon Contains Z, Y and A structural genes - lacZ – codes for )-galactosidase, which hydrolyzes lactose to galactose and glucose - lacY – codes for permease, which facilitates movement of lactose into the cell - lacA – codes for thiogalactoside transacetylase, which acetylates lactose When glucose is available, the operon is repressed by binding of the repressor protein (product of lacI gene) to the operator, thus preventing transcription When only lactose is present, the operon is induced by an isomer of lactose (allactose) that binds the repressor protein, preventing it from binding to the operator. In addition, cAMP binds the catabolite activator protein (CAP), and the complex binds the DNA at the CAP site. This increases promoter efficiency and results in the expression of the structural genes through the production of a polycistronic mRNA When both glucose and lactose are present, glucose prevent formation of cAMP, and transcription of these genes is negligible Trp operon Contain five genes needed for synthesis of tryptophan and is regulated by negative control When trp is plentiful, transcription is regulated by attenuation, in which mRNA synthesis that escaped repression by Trp is terminated before completion. This is done by the formation of an attenuator, a hairpin (stem-loop) structure in the mRNA. Attenuation can also result in a truncated, nonfunctional peptide If Trp become scarce, the operon is expressed Stringent response Response to amino acid starvation 28 Emma notes Binding of uncharged tRNA to A site of ribosome leads to production of alarmone guanosine triphosphate (ppGpp) from GDP, catalyzed by stringent factor (RelA) Elevated levels of ppGpp results in inhibition of rRNA synthesis (+ tRNA and some mRNA) Regulatory ribosomal proteins Operons for ribosomal proteins (r-proteins) can be inhibited by an excess of their own protein products. For each operon, one specific r-protein functions in the repression of translation of the polycistronic mRNA from that operon The r-protein does so by binding to the Shine-Dalgarno (SD) sequence located on the mRNA just upstream of the first initiating AUG codon and acting as a physical impediment to the binding of the small ribosomal subunit to the SD sequence. Thus, one r-protein inhibits synthesis of all the r-proteins of the operon Galactose circuit Use of galactose when glucose is unavailable Gal4 – a STF with DNA-binding domain (DBD) and transcription activation domain (TAD). It binds to a DNA sequence upstream of genes for metabolism of galactose Gal4 binds to UASGal both in absence and presence of galactose - When absent, Gal80 binds Gal4 at its TAD and inhibit transcription - When present, Gal3 binds Gal80 (inhibits inhibition of Gal4), and allows Gal4 to activate transcription Intracellular receptors Includes steroid hormone, vitamin D, retinoid acid, and thyroid hormone receptors, function as STF Binding of hormone to the receptor causes a conformational change that activates the receptor. Receptor-hormone complex enters nucleus, dimerizes, and binds via a zinc finger at the hormone response element (HRE) Binding allows recruitment of coactivators to TAD and results in expression of genes The HRE can be located upstream or downstream of the genes it regulates, and in great distance from them Cell-surface receptors – glucagon Glucagon binds to its G protein-coupled plasma membrane receptor. The extracellular signal is transduced to intracellular cAMP (from ATP) In response to a rise in cAMP, a trans-acting factor, CREB (cAMP response element-binding protein), is phosphorylated and activated Active CREB binds to a cis-acting regulatory element, CRE (cAMP response element), resulting in transcription of target genes mRNA processing Alternative splicing – exon skipping, intron retention, use of alternative splice-donor or acceptor sites. Over 90% of all human genes undergo alternative splicing - Example is tropomyosin, alternative splicing yields a number of isoforms 29 Emma notes Alternative polyadenylation (APA) – generates mRNA with different 3´-ends, altering the untranslated region or the coding sequence. APA is involved in production of IgM mRNA editing Posttranscriptional modification, in which a base in the mRNA is altered Ex. if the C base in the CAA (glutamine) codon that codes for ApoB (essential for chylomicrons and VLDL) is deaminated to uracil, the stop codon UAA is made. This results in a shorter protein (ApoB-48) being made à only in intestine, protein in liver is ApoB-100 Iron metabolism When iron concentration in the cell is low, iron regulatory protein (IRP) binds to the 3´-IRE (iron responsive element) and stabilize the mRNA for Transferrin receptor (TfR), allowing TfR synthesis When iron levels are high, IRP dissociate and result in decreased TrF synthesis Low iron also causes IRP to bind to 5´-IRE on the mRNA for ferritin and prevents its use à less ferritin is made. When iron accumulates in the cell, IRP dissociate, allowing synthesis of ferritin molecules to store the excess iron RNA interference RNAi is a mechanism of gene silencing through decreased expression of mRNA, either by repression of translation or by increased degradation Mediated by short noncoding miRNA à arise from longer primary miRNA, that are partially processed in the nucleus to pre-miRNA by endonucleases (Drosha) then transported to the cytoplasm. There, an endonuclease (Dicer) completes the processing and generates short, double-stranded miRNA A single strand of miRNA associates with cytosolic RNA-induced silencing complex (RISC) and hybridizes a complementary sequence in the 3´-UTR of a full-length target mRNA, bringing RISC to the mRNA. This can result in repression of translation of mRNA or its degradation by endonuclease (Aronaute/Ago/Slicer) of the RISC Age-related macular degradation Triggered by overproduction of vascular endothelial growth factor (VEGF), leading to sprouting of excess blood vessels behind the retina The vessels leak, clouding and often destroys vision An siRNA was designed to target the mRNA of VEGF and promote its degradation No RNAi products for treatment have gone to the market mRNA translation Regulation of gene expression at mRNA translation level Phosphorylation of eIF-2 inhibits its function and so inhibits translation at initiation step Catalyzed by kinases in response to amino acid starvation, heme deficiency, presence of dsRNA (viral infection) and accumulation of misfolded proteins in RER Regulation through variations in DNA 30 Emma notes Access to DNA: - Active, decondensed chromatin contains histone proteins that have been covalently modified by reversible methylation, acetylation or phosphorylation. Such modifications decrease their positive charge, thereby decreasing the strength of their association with negatively charged DNA – relaxes nucleosome - Nucleosomes can also be repositioned, an ATP-requiring process - Methylation of cytosine bases in CG-rich regions (CpG islands) by methyltransferases results in silencing of gene expression. SAM is the methyl donor. Transcriptionally active genes are less methylated Amount of DNA Arrangement of DNA Mobile DNA elements 31 Emma notes 8. Protein synthesis Features of genetic code Specificity – the genetic code is unambiguous, the codon always code for the same amino acid Universality – the genetic code is universal for all living organisms; it has been conserved from early stages of evolution (mitochondria is an exception) Degeneracy – the genetic code is degenerate à a given amino acid may have more than one triplet coding for it (ex. Phe – UUU, UUC) Nonoverlapping and commaless – the code is read from a fixed starting point as a continuous sequence of bases, taken three at a time without any punctuations (AGCUGGAUACAU is read AGC UGG AUA CAU) Types of mutations Point mutations 1. Silent mutation – codon with changed base code for the same amino acid, no detectible effect 2. Missense mutation – codon with changed base code for different amino acid 3. Nonsense mutation – codon become a stop codon, cause premature termination of translation and a shortened protein (stop codons: UAA, UAG, UGA) Trinucleotide repeat expansion – a sequence of triplets that is repeated in tandem is amplified, cause too many copies of an amino acid in the protein. Can cause disease, like Huntington and fragile X syndrome Splice-site mutation – can alter the way introns are removed, producing aberrant proteins Frame-shift mutation – one or two nucleotides are added or deleted, which alter the reading frame, resulting in a different protein or a truncated product à If a triplet is added or deleted, the reading frame is not changed but can result in serious pathology Trinucleotide repeat expansion – Huntington disease If a sequence of three bases repeated in tandem gets amplified, too many copies of the amino acid will occur in the protein Huntington is a disease where the exon 1 in the gene for huntingtin protein contains too many copies of the CAG codon (glutamine) - Normal repeats < 27, in Huntington > 36) The additional glutamines result in an abnormally long protein that is cleaved, producing toxic fragments that aggregate in neurons and cause neurodegenerative problems Trinucleotide repeat expansion in UTR´s can result in decrease of the amount of protein produced, seen in fragile X syndrome (CGG > 200) and myotonic dystrophy (CUG – 100-1000) Cystic fibrosis A chronic, inherited disease (AR), often caused by deletion of three nucleotides from the coding region of a gene 32 Emma notes This causes loss of phenylalanine at the 508th (∆F508) position in the CF transmembrane conductance regulator (CFTR) protein, which prevents normal folding, leading to its destruction by the proteasome CFTR usually function as a chloride channel in epithelial cells (pancreas, lungs, testes and sweat glands), and its loss results in production of thick, sticky secretions in lungs and pancreas, leading to lung damage and digestive deficiencies Clogging of pancreatic ducts lead to pancreatic insufficiency à treated with replacement of pancreatic enzymes and supplementation with fat-soluble vitamins Components required for protein synthesis Amino acids – if an AA is missing, translation stops mRNA – template for the synthesis tRNA – carboxyl group of AAS is in an ester linkage with 3´-hydroxyl of the ribose portion of the A nucleotide in the -CCA sequence. When an AA is attached, tRNA is charged Functional ribosomes – proteins and rRNA. Consists of a small and a large subunit Energy sources – 2 ATP and 2 GTP for elongation, GTP (+ ATP in eukaryotes) for initiation, GTP for termination Enzymes – aminoacyl-tRNA synthetases, catalyze reaction of binding amino acids to tRNA Protein factors – catalytic or stabilizing functions for initiation, elongation, and termination Aminoacyl-tRNA synthesis Each aminoacyl-tRNA synthetase recognizes a specific amino acid and all the tRNA that corresponds to that amino acid The ,-carboxyl group of an amino acid attaches in an ester linkage to the A in the -CCA sequence at the 3´-end of its corresponding tRNA Reaction requires ATP that is cleaved to AMP + PPi (and then 2Pi) Aminoacyl-tRNA synthetase have a proofreading activity à removes incorrect amino acids from the enzyme or tRNA Ribosomes Consist of proteins and rRNA Has a small and a large subunit: 50S + 30S (prokaryotes), 60S + 40S (eukaryotes) Has three binding sites for tRNA: - A site – binds incoming aminoacyl-tRNA. The codon of the A site specifies the next amino acid to be added - P site – occupied by peptidyl-tRNA, which carries the chain of amino acids that has already been synthesized - E site – occupied by empty tRNA as it is about to exit Ribosomes are either free in cytosol or associated with RER - Cytosolic proteins – destined for cytosol, nucleus, mitochondria, or peroxisomes - RER-proteins – exported from the cell, incorporated into membranes, or imported into lysosomes The small subunit of ribosome binds mRNA and determines the accuracy of translation 33 Emma notes High-energy bonds used for peptide bond formation Cleavage of four high-energy bonds is required for the addition of one amino acid to the polypeptide chain Two from ATP – in aminoacyl-tRNA synthetase reaction: - One in the removal of PPI - One in hydrolysis of PPi to 2Pi, by pyrophosphatase Two from GTP: - One for binding aminoacyl-tRNA to the A site - One for the translocation step Wobble hypothesis The two first bases of the codon follow the regular rule for base-pairing (C-G, A-U) with the anticodon The third base of the codon and the first base of the anticodon may have non-traditional base-pairing, which allows a tRNA to recognize more than one codon for a specific amino acid The result of wobble is that 61 tRNA species are not required to read the 61 amino acids Hypoxanthine is the product of adenine deamination and the base in inosine monophosphate – can pair with U, C and A Initiation Assembly of components – two ribosomal subunits, mRNA, specified aminoacyl-tRNA, GTP and initiation factors Initiation factors are IF-1, IF-2 and IF3 in prokaryotes, and many, designated eIF in eukaryotes (which also require ATP) Initiation is facilitated by two mechanisms: 1. Prokaryotes: Purine-rich Shine-Dalgarno sequence is located six to ten bases upstream of the AUG codon, near the 5´-end. The 3´-end of the 16S rRNA component of the small ribosomal subunit form complementary base pairs with the SD-sequence, which facilitate positioning of the 30S subunit close to the start codon 2. Eukaryotes: The small ribosomal subunit binds close to the cap structure at the 5´-end of mRNA and moves 5´→ 3´ along the mRNA until it reaches the AUG codon. This process requires ATP. Cap-independent initiation can occur if the 40S subunit binds to an internal ribosome entry site close to the start codon IF-2-GTP in prokaryotes and eIF-2-GTP in eukaryotes facilitates recognition of the initiating AUG by initiator tRNA (tRNAi), which is distinguished from tRNA used for internal AUG by base modifications, and goes directly to the P site The Met on tRNAi in prokaryotes and mitochondria is formylated by transformylase (carbon donor is N10-formyl-THF). Eukaryotic Met is not formylated GTP on (e)IF-2 gets hydrolyzed to GDP Elongation 34 Emma notes Delivery of aminoacyl-tRNA with the next amino acid to the ribosomal A site is facilitated by elongation factors EF-Tu-GTP and EF-Ts, and requires GTP hydrolysis (EF-1,-GTP and EF-1)* in eukaryotes) Peptidyltransferase catalyzes peptide-bond formation between ,-carboxyl group of the amino acid in the P site and the ,-amino group of the amino acid in the A site - Peptidyltransferase is a part of rRNA and is a ribozyme The peptide on the tRNA at the P site is transferred to the amino acid on the tRNA at the A site, a process called transpeptidation The ribosomes then advance three nucleotides toward the 3´-end, a process called translocation – requires EF-G-GTP (prokaryotes) or EF-2-GTP (eukaryotes) and GTP hydrolysis. Translocation moves uncharged tRNA from P site to E site for release, and peptidyl-tRNA from A site to P site Termination One of three termination codons (UAA, UAG, UGA) moves into the A site Release factors (RF-1 and RF-2, eRF) recognizes the codons and binds to the A site - RF-1 recognizes UAA and UAG, RF-2 recognizes UGA and UAA, eRF recognizes all Hydrolysis of the bond linking the peptide to the tRNA at the P site à nascent protein is released from the ribosome (e)RF-3-GTP causes the release of the release factors (RF-1, RF-2, eRF) as GTP is hydrolyzed Ribosomal subunits, mRNA, tRNA and protein factors can be recycled and used to synthesize another polypeptide Translation regulation Most commonly regulated at transcriptional level, but translation may also be regulated An important mechanism is phosphorylation of eIF-2, makes it inactive Protein targeting Many proteins perform their functions within subcellular organelles or outside the cell. Such proteins normally contain amino acid sequences that direct the proteins to their final locations For example, secreted proteins are targeted during synthesis to the RER by the presence of N-terminal hydrophobic signal sequence, which is recognized by the signal recognition particle (SRP), a ribonucleoprotein that binds the ribosome, halts elongation and delivers the ribosome-peptide complex to an RER membrane channel (translocon) via interaction with the SRP receptor. Translation resumes, the protein enters the RER lumen, and its signal sequence is cleaved. The protein moves through the RER and Golgi, is processed, packaged into vesicles and secreted Proteins targeted after synthesis include nuclear proteins that contain an internal, short, basic nuclear localization signal, mitochondrial matrix proteins that contain an N-terminal, amphipathic, ,-helical mitochondrial entry sequence, and peroxisomal proteins that contain a C-terminal tripeptide signal Posttranslational modifications 35 Emma notes Trimming – inactive precursor molecules require removal of portions by endoproteases to become active proteins. Some are cleaved in RER or Golgi, other in secretory vesicles (insulin) and some after secretion (collagen) Covalent attachments: 1. Phosphorylation – occurs on hydroxyl groups of serine, threonine and tyrosine, catalyzed by protein kinases and reversed by protein phosphatases. Phosphorylation increase or decrease functional activity of the protein 2. Glycosylation – carbohydrate chains added to the amide nitrogen of asparagine (N-linked in RER) or built sequentially on the hydroxyl group of serine threonine or hydroxylysine (O-linked in Golgi). Many glycosylated proteins are destined for membranes or to be secreted from a cell 3. Hydroxylation – proline and lysine residues of the ,-chain of collagen are extensively hydroxylated by vitamin C-dependent hydroxylases in RER 4. Other modifications – carboxylation, acetylation and attachment of lipids Protein degradation Defective proteins are often marked for destruction by ubiquitination (covalent attachment of small, highly conserved protein called ubiquitin) Marked proteins are rapidly degraded by the proteasome (cytosolic macromolecular, ATPdependent, proteolytic system) Antibiotics Antibiotics can inhibit the different stages of protein synthesis Streptomycin – binds to 30S subunit and distorts its structure, interfering with initiation Tetracycline – interact with 30S subunit, blocking access of the aminoacyl-tRNA to the A site, thereby inhibiting elongation Puromycin – bears a structural resemblance to aminoacyl-tRNA and accepts a peptide from the P site, causing inhibition of elongation and resulting in premature termination in both prokaryotes and eukaryotes Chloramphenicol – inhibits prokaryotic peptidyltransferase. High levels may also inhibit mitochondrial protein synthesis Erythromycin – binds irreversibly to a site on the 50S subunit and blocks the tunnel by which the peptide leaves the ribosome, thereby inhibiting translocation Diphtheria toxin – inactivates EF-2, thereby inhibiting the translocation phase of elongation. In eukaryotes Ricin – a toxin from castor beans, removes a specific A from the 28S rRNA in the large subunit, thereby inhibiting ribosomal function. In eukaryotes Prokaryotic vs eukaryotic protein synthesis In prokaryotes, transcription and translation is temporally linked due to lack of nuclear membrane Initiation - Prokaryotes: IF-1, IF-2, IF-3, GTP, SD-sequence, fMet - Eukaryotes: many eIF, GTP + ATP, 5´-Cap, Met Elongation 36 Emma notes - Prokaryotes: EF-Tu-GTP, EF-Ts, EF-G-GTP - Eukaryotes: EF-1,-GTP, EF-1)*, EF-2-GTP Termination - Prokaryotes: RF-1, RF-2, RF-3-GTP - Eukaryotes: eRF, eRf-3-GTP 9. Catabolism of amino acids: nitrogen Nitrogen metabolism Amino acids are not stored in body AAs must be obtained from diet, synthesized de novo, or produced from normal protein degradation Nitrogen enters body in variety of ways à most importantly through AAs Nitrogen leaves as urea, ammonia, and other products derived from amino acids metabolism Amino acid pool - Free AAs are present in blood and ECF - Small (90 – 100 g) - In healthy persons à input and output is balanced - AA pool is supplied by 3 sources 1. AA provided by degradation of body proteins 2. AA derived from dietary protein 3. Synthesis of nonessential AAs from simple intermediates of metabolism - AA pool is depleted by 3 routes 1. Synthesis of body protein 2. AAs consumed as precursors of essential nitrogen – containing small molecules 3. Conversion of AAs to glucose, glycogen, FA, ketone bodies or CO2 + H2O Protein turnover - Proteins are constantly being synthesized and degraded - In healthy persons, total amount of protein in body is constant à rate of synthesis is sufficient to replace degradation - 300 – 400 g of protein each day is hydrolysed and resynthesized - Short – lived proteins (regulatory proteins and misfolded) have half – lives of min – hours - Long – lived proteins (majority of proteins in cells) have half – life of days – weeks - Structural proteins (collagen) have half – life in months – years - 2 major enzyme system degrade proteins: 1. Lysosome (ATP – independent) § Extracellular proteins § Acid hydrolases 2. Ubiquitin – proteasome proteolytic pathway § ATP – dependent § Endogenous proteins § Protein selected for degradation is tagged with molecules of ubiquitin § Ubiquitinated proteins are recognized by cytosolic proteasome, which unfolds, deubiquitinates, and transports the protein to its proteolytic core § Peptide fragments produced by the proteasome are degraded to amino acids in the cytosol - Signals for degradation § Half – life is influence by N – terminal residue of AA § Serine as N – terminal is long lived (20h) § Aspartate has only 3 minutes 37 Emma notes § Proline, glutamate, serine, threonine sequence (PEST) containing proteins are rapidly degraded Digestion of dietary proteins Proteins are too large to be digested by intestines à hydrolysed to di and tripeptides as well as AAs Gastric digestion - Hydrochloric acid § Kill bacteria and denature proteins - Pepsin § Endopeptidase § Secreted by chief cells of stomach as inactive zymogen à pepsinogen § Pepsinogen is activated to pepsin, either by HCl or autocatalytically by other pepsin molecules that already is activated § Release pepsin and few AAs Pancreatic digestion - Pancreatic proteases § Endopeptidases – cleave within à trypsin, chymotrypsin, elastase § Exopeptidases – cleaves at end à carboxypeptidase A and B - Each enzyme har specificity for amino acid R – groups adjacent to peptide bond - Trypsin and carboxypeptidase B cleaves when carbonyl group is arginine or lysine - Chymotrypsin cleaves when its tryptophan, tyrosine, phenylalanine, methionine, or leucine - Elastase cleaves alanine, glycine, and serine - Carboxypeptidase A cleaves alanine, isoleucine, leucine, or valine - Cholecystokinin mediates secretion and activation of pancreatic zymogens - Enteropeptidase (enterokinase) – serine protease – converts pancreatic zymogen trypsinogen into trypsin - Deficiency of pancreatic secretion § CF, chronic pancreatitis, or removed pancreas § Digestion and absorption of fat and protein are incomplete § Results in abnormal appearance of lipid in feces à steatorrhea Intestinal digestion - Aminopeptidase (exopeptidase) on luminal surface of intestines - Cleaves N – terminal residue from oligopeptides to produce smaller peptides and free AAs AAs and small peptide absorption - AAs are taken into enterocytes via sodium – dependent secondary active transport by solute carrier (SLC) proteins - Di – and tripeptide are taken up by proton – linked peptide transporter (PepT1) and hydrolysed to free AAs - The AAs are transported into blood by sodium – independent transporters à only free AAs are found in portal vein after a meal containing protein - AAs are metabolized by liver or released into circulation (BCAA are not metabolized in liver but to muscle via blood) Celiac disease Malabsorption disease Resulting from immune – mediated damage to small intestine in response to ingestion of gluten à protein found in wheat, barley, and rye 38 Emma notes Absorption abnormalities Cystinuria - Disorder of proximal tubule reabsorption of filtered cysteine, ornithine, arginine, and lysine (COAL) Cystinosis - Rare defect in transport of cysteine out of lysosome - Results in formation of cysteine crystals within lysosome and widespread tissue damage Hartnup disorder - Defects in uptake of tryptophan by a neutral amino acid transporter - Pellagra like dermatologic and neurologic symptoms Removal of nitrogen from amino acids Removing NH3 is essential for producing energy from any amino acid and is an obligatory step in catabolism of AAs Transamination - Transfer of NH3 group to alpha – ketoglutarate producing alpha – keto acid and glutamate - Catalysed by aminotransferases (transaminases) § Found in cytosol and mitochondria throughout body § Requires pyridoxal phosphate (PLP) (B6) à linked to specific lysine residue - All AAs except lysine and threonine participate in transamination at some point in their catabolism - Each aminotransferase is specific for the amino donor group - Alanine aminotransferase (ALT) § Present in many tissues § Catalyse transfer of NH3 of alanine to alpha – ketoglutarate à resulting in pyruvate and glutamate § Reversible reaction § Acts in direction of glutamate during AA catabolism - Aspartate aminotransferase (AST) § Exception to rule that aminotransferases funnel amino groups in direction of glutamate § AST primarily transfers NH3 from glutamate to oxaloacetate à forming aspartate, used as nitrogen source in urea cycle § Reversible like other transamination’s - Diagnostic value § Elevated levels of intracellular ALT and AST may indicate liver disease or damage to cells rich in these enzymes (hepatocytes) § ALT is more specific for liver disease, but AST is more sensitive sine liver contains large amounts of AST § Aminotransferases may be elevated in myocardial infarction and muscle disorders Oxidative deamination - Results in liberation of NH3 as free ammonia - Reactions occur primarily in liver and kidney - Provide alpha – keto acids that can enter central pathways of energy metabolism and ammonia à source of nitrogen in hepatic urea synthesis - Ammonia exists primarily as ammonium (NH4+) à unionized NH3 that cross membranes - Glutamate dehydrogenase (GDH) § Glutamate is only AA that undergoes rapid oxidative deamination 39 Emma notes Mitochondrial enzyme that uses either NAD+ or NADPH as coenzyme NAD+ primarily in oxidative deamination à loss of ammonia NADPH in reductive amination à gain of ammonia Direction depends on concentrations of glutamate, alpha – ketoglutarate, and ammonia and ratio of NAD/NADPH § After protein rich meal à glutamate rich à direction of ammonia formation § GTP allosterically inhibits GDH while ADP activates - D – amino acid oxidase (DAO) § D – amino acids are supplied by diet but not used in synthesis of mammalian proteins § They are metabolized to alpha – keto acids, ammonia, and hydrogen peroxide in peroxisomes of liver and kidney by FAD dependent DAO Ammonia transport to liver - 2 mechanisms are available - Glutamine synthetase § Combines ammonia with glutamate to form glutamine à nontoxic transport form of ammonia § Glutamine is transported in blood to liver à cleaved by glutaminase to glutamate and ammonia § Glutamate is oxidatively deaminated à NH3 and alpha – ketoglutarate § NH3 à urea - Formation of alanine § Transamination of pyruvate produced from aerobic glycolysis and metabolism of succinyl CoA generated by catabolism of BCAA isoleucine and valine § Alanine is transported in blood to liver à transaminated by ALT to pyruvate § Pyruvate is used to synthesize glucose à can enter blood and be used by muscle à glucose – alanine cycle § § § § Urea cycle Major disposal form of amino groups from AAs 90% of nitrogen – containing components in urine Produced by liver and transported in blood to kidneys and excretion in urine Reactions (green cytosolic) (blue mitochondrial) 1. Carbamoyl phosphate is formed by carbamoyl phosphate synthetase I à cleavage of 2 ATP with free NH3 (first nitrogen of urea) and CO2 CPS I require n – acetylglutamate as allosteric activator 2. Carbamoyl phosphate is transported to ornithine, by ornithine transcarbamylase to form citrulline à transported into cytosol 3. Argininosuccinate synthetase combines citrulline with aspartate (2nd nitrogen) à form argininosuccinate, driven by ATP cleavage to AMP + PPi 4. Argininosuccinate is cleaved by argininosuccinate lyase to provide arginine and fumarate (converted to malate à OAA) 5. Arginine is cleaved by arginase to ornithine and urea (occurs almost exclusively in liver) 6. Urea diffuses from liver and is transported in blood to kidneys à urine Portion of urea diffuses from blood into intestines à cleaved by bacterial urease to CO2 and NH3 Aspartate + NH3 + CO2 + 3ATP + H2O à urea + fumarate + 2 ADP + AMP + 2Pi + PPi N – acetylglutamate is essential activator of CPS – 1 à rate – limiting step of urea - Its synthesized from acetyl CoA and glutamate by N – acetylglutamate synthase - Increases after protein rich meal à urea synthesis increases 40 Emma notes Metabolism of ammonia Sources of ammonia - Glutamine § Kidneys generate NH3 from glutamine by glutaminase and glutamate dehydrogenase à excreted in urine as NH4+ à acid – base balance § Intestinal glutaminase can also generate NH3 from glutamine - Bacterial action § NH3 is formed from urea by action of bacterial urease - Amines § Obtained from diet and monoamines that serve as hormones or neurotransmitters give rise to ammonia by action of amine oxidase - Purines and pyrimidines § AAs attached to ring atoms are released as ammonia in purines/pyrimidines catabolism Transport of ammonia in circulation - Urea § Most important disposal routes § Travels in blood from liver to kidneys à into glomerular filtrate - Glutamine § Nontoxic storage and transport form § Glutamine synthetase converts glutamine from glutamate and ammonia à in skeletal muscle and liver, but most important in CNS à major removal mechanism Hyperammonaemia Normal level is 5 – 35 µmol/l Above 1000 µmol/l is a medical emergency à direct neurotoxic effect on CNS Symptoms include tremors, slurred speech, somnolence, vomiting, cerebral edema, and blurring of vision à death and coma at very high concentrations 2 types of hyperammonaemia - Acquired § Liver disease à hepatitis or hepatotoxins (alcohol) § Cirrhosis - Congenital § Genetic deficiencies of all 5 enzymes of urea cycle § X – linked OTC deficiency is most common § All other urea cycle disorder follows AR pattern § Failure to synthesize urea leads to hyperammonaemia during first weeks following birth § Arginase deficiency is less severe because arginine contains 2 waste nitrogen and can be excreted in urine 41 Emma notes 10. Catabolism and synthesis of AAs Nonessential Glucogenic Alanine AAs that yield pyruvate or Arginine other intermediates of Asparagine glycolysis Aspartate Cysteine Glutamate Glutamine Glycine Proline Serine Glucogenic + ketogenic Tyrosine Ketogenic Yield acetoacetate or acetyl/acetoacetyl CoA Essential Histidine Methionine Threonine Valine Isoleucine Phenylalanine Tryptophan Leucine Lysine AAs that form oxaloacetate Asparagine is hydrolysed by asparaginase à NH3 and aspartate Aspartate is transaminated into OAA by AST AAs that form alpha – ketoglutarate via glutamate Glutamate is converted to alpha – ketoglutarate by transamination or glutamate dehydrogenase Glutamine à hydrolysed to glutamate and NH3 by glutaminase Proline à oxidized to glutamate Arginine à hydrolysed to ornithine + urea à ornithine is converted to a - ketoglutarate Histidine à oxidatively deaminated by histidase to urocanic acid à form F – formiminoglutamate à glutamate AAs that form pyruvate Alanine à transamination to pyruvate by ALT Tryptophan à produced alanine à pyruvate Serine à converted to glycine as THF (tetrahydrofolate) becomes N5, N10 – methylenetetrahydrofolate à also pyruvate Glycine à converted to serine by addition of methylene group from N5, N10 – MTHF Cysteine à desulfurization to form pyruvate à can also be oxidized to cysteine Threonine à converted to pyruvate but minor pathway in humans AAs that form fumarate 42 Emma notes Phenylalanine and tyrosine à hydroxylation of phenylalanine produces tyrosine catalysed (irreversibly) by phenylalanine hydroxylase (PAH) which requires tetrahydrobiopterin AAs that form succinyl CoA Methionine - Condenses with ATP à forming SAM - Methyl group attached to sulphur in SAM is activated and can be transferred by methyltransferases to NE in synthesis of Epi à produces S – adenosylhomocysteine (SAH) - SAH is hydrolysed to Hcy and adenosine - Hcy can be resynthesized to methionine à requires methyl cobalamin (B12) - Or Hcy can condense with serine forming cystathionine à hydrolysed to alpha – ketobutyrate and cysteine - Hcy levels promote oxidative damage, inflammation, and endothelial dysfunction à risk factor for occlusive vascular disease and stroke Elevated Hcy and decreased folic acid levels in pregnant women are associated with increased incidence of neural tube defects (spina bifida) Valine and isoleucine (BCAA) generate propionyl CoA à converted to methylmalonyl CoA à then succinyl CoA by biotin and vit. B12 requiring reactions Threonine is dehydrated to alpha – ketobutyrate à converted to propionyl CoA and then succinyl CoA AAs that form acetyl CoA or acetoacetyl CoA Tryptophan à alanine and acetoacetyl CoA Leucine à acetoacetyl and acetyl CoA Isoleucine à acetyl CoA and propionyl CoA Lysine à acetoacetyl CoA BCAA degradation Leucine, isoleucine, and valine are BCAA à catabolized in peripheral tissues primarily muscle Transamination - Transfer of NH3 of all 3 to alpha – ketoglutarate à by BCAA aminotransferase requires PLP (vit. B6) - Primarily skeletal muscle Oxidative decarboxylation - Removal of COOH group of alpha – keto acids derived from BCAA is catalysed by branched chain alpha – keto acid dehydrogenase (BCKD) complex - Uses TPP, lipoic acid, FAD, NAD+, and CoA as coenzymes and produce NADH Dehydrogenation - Oxidation of products produced from BCKD reaction produces alpha – beta – unsaturated acyl CoA derivatives and FADH2 End products - Acetyl CoA and succinyl CoA à isoleucine - Succinyl CoA à valine - Acetoacetate and acetyl CoA à leucine One – carbon metabolism Some synthetic pathways require addition of single carbon groups à formyl, methylene, methenyl, or methyl 43 Emma notes They can be transferred from carrier compounds like THF and SAM Tetrahydrofolate (THF) is active form of folic acid. Produced from folate by dihydrofolate reductase in 2 - step requiring NADPH 1 C carried by THF is bound to N5 or N10 or both CO2 from HCO2 is carried by vitamin biotin à prosthetic group for most carboxylation reactions but not a member of one – carbon pool Synthesis of non – essential amino acids Alanine, aspartate, and glutamate are synthesized by transfer of amino group to alpha – keto acids pyruvate, OAA, and alpha – ketoglutarate Glutamine contains amide linkage with NH3 at g - carboxyl is formed from glutamate, by glutamate synthetase à driven by ATP hydrolysis Asparagine contains amide linkage with NH3 at b - carboxyl is formed from aspartate, by asparagine synthetase à glutamine as amide donor à requires ATP Proline is formed from glutamate via glutamate semialdehyde Serine arises from 3 – phosphoglycerate, can also be formed from glycine Glycine is synthesized from serine by removal of hydroxymethyl group by serine hydroxymethyltransferase à THF is one – carbon donor Cysteine is synthesized by 2 reactions where Hcy combines with serine à forming cystathionine which is hydrolysed to alpha – ketobutyrate and cystine Tyrosine is formed from phenylalanine by PAH à requires 2 O2 and tetrahydrobiopterin (BH4) Metabolic defects in amino acid metabolism Phenylketonuria Deficiency of PAH Most common AA metabolism inborn error Hyperphenylalaninemia in blood, urine, and body tissues Tyrosine is deficient New – born screening is done Characteristics are - Elevated phenylpyruvate, phenylacetate, and phenylacetate à mousy odor - Disability, developmental delay, microcephaly, and seizures - Low IQ - Hypopigmentation Treatment - Replace proteins with synthetic amino acid preparations free of phenylalanine - Must begin 7 – 10 days after birth to avoid cognitive impairment Albinism Defect in tyrosine metabolism à deficiency if melanin Partial or full absence of pigment in hair, skin, and eyes Can be AR (primary), AD, or X – linked Type 1 albinism à tyrosinase – negative oculocutaneous albinism from absent of defective tyrosinase (Cu – requiring) Alkaptonuria Rare organic aciduria involving deficiency of homogentisic acid oxidase Homogentisic acid (HA) accumulates Characteristics - Homogentisic aciduria (HA accumulates in urine and turns very dark) - Early onset of arthritis in large joints - Deposition of black pigment (ochronosis) in cartilage and collagenous tissue 44 Emma notes Treatment includes dietary restriction of phenylalanine and tyrosine to reduce HA levels Tyrosinemia type 1 Deficiency of fumarylacetoacetate hydrolase Fumarylacetoacetate and succinyl acetone accumulates in urine Characteristics cabbage – like odor Liver failure and renal tubular acidosis Treatment includes dietary restriction of phenylalanine and tyrosine and substrate reduction therapy Methylmalonic acidemia Deficiency in methylmalonyl CoA mutase or adenosylcobalamin Elevated levels of methylmalonic acid in blood Metabolic acidosis and developmental problems occur Histidinemia Deficiency of histidase Elevated levels of histidine occur in blood and urine Benign condition in most individuals Cystathioninuria Deficiency of cystathionase Accumulation of cystathionine Benign Homocystinuria Deficiency of cystathionine synthase Accumulation of Hcy in urine Methionine and Hcy are elevated in blood, cysteine low Skeletal abnormalities, increased risk of clotting, lens dislocations, and intellectual disability occur Maple syrup urine disease Deficiency of branched – chain alpha – keto acid dehydrogenase Levels of BCAA and their alpha – keto analogs are elevated in plasma and urine Neurologic problems are common à high mortality rate Treatments include restricted dietary intake of BCAA 45 Emma notes 11. Conversion of AAs to specialized products Porphyrin metabolism Porphyrins are cyclic compounds that binds metal ions à ferrous (Fe2+) or ferric (Fe3+) iron Most prevalent metalloporphyrin in humans à heme Heme is prosthetic group of hemoglobin, myoglobin, cytochromes, cytochrome P450 monooxygenase system, catalase, nitric oxide synthase, and peroxidases Structure of porphyrin - Cyclic planar molecular formed by linkage of 4 pyrrole rings by methylene bridges - Different porphyrins have different side chains attached to each 4 pyrrole rings - Can be § Acetate and propionate § Methyl and propionate § Vinyl (2), methyl (4), and propionate (2) à protoporphyrin IX (most common heme) - Porphyrinogens § Porphyrin precursor (ex. Uroporphyrinogen) exists in chemically reduced, colourless form Heme biosynthesis Major sites; liver and bone marrow (85% BM) Delta – aminolevulinic acid formation (mitochondria) - Glycine and succinyl CoA condense to form d - aminolevulinic acid (ALA) - Catalysed by ALA synthase in rate – limiting step - ALA synthase 1 present in all tissues, while ALA synthase 2 is erythroid specific - Heme decreases activity and amount of ALAS1 - Large number of drugs results in significant increase in hepatic ALAS1 activity Porphobilinogen formation (PBG) (cytosol) - 2 ALA to form PBG by ALA dehydratase (zinc containing) - Inhibited by lead (replace zinc) à elevates ALA and cause anemia Uroporphyrinogen formation (cytosol) - Condensation of 4 PBG produces hydroxymethylbilane by hydroxymethylbilane synthase - Hydroxymethylbilane is isomerized to uroporphyrinogen III by uroporphyrinogen III synthase - Uroporphyrinogen III is decarboxylated by uroporphyrinogen III decarboxylase (UROD) generating coproporphyrinogen III Heme formation (coproporphyrinogen III moves into mitochondria) - Coproporphyrinogen III has 2 propionate side chains which are decarboxylated into vinyl groups forming protoporphyrinogen IX, by coproporphyrinogen III oxidase - Porphyrinogen IX is oxidized to protoporphyrin IX by protoporphyrinogen oxidase - Introduction of Fe2+ to protoporphyrin produced heme - This is spontaneously but is enhanced by ferrochelatase - Can be inhibited by lead 46 Emma notes Porphyrias Hepatic Acute intermittent porphyria - Acute AD caused by deficiency of hydroxymethylbilane synthase - Porphobilinogen and ALA accumulates in urine - Urine darkens on exposure to light - Not photosensitive Varigate porphyria - Acute AD caused by deficiency of protoporphyrinogen oxidase - Protoporphyrinogen IX and other intermediates accumulates in urine Hereditary coproporphyria - Acute AD caused by deficiency of coproporphyrinogen III oxidase - Coproporphyrinogen III and other intermediates accumulates in urine - Photosensitive Porphyria cutanea tarda - Chronic AD deficiency in uroporphyrinogen decarboxylase - Uroporphyrin accumulates in urine - Most common porphyria - Photosensitive Bone marrow Erythropoietic protoporphyria - Chronic AS and AR caused by deficiency of ferrochelatase - Protoporphyrin accumulates in RBCs, bone marrow, and plasma - Photosensitive Congenital erythropoietic porphyria - Chronic AR caused by deficiency of uroporphyrinogen III synthase - Uroporphyrinogen I and coproporphyrinogen I accumulates in urine Lead poisoning Ferrochelatase and ALA dehydratase are sensitive to lead inhibition Proporphyrin and ALA accumulates in urine ALAD deficiency porphyria is a very rare AR acute hepatic porphyria Heme degradation After ca. 120 days in circulations à RBCs are taken up and degraded by mononuclear phagocyte system (MPS) à liver and spleen Bilirubin formation - Heme is catalysed to biliverdin by heme oxygenase - Biliverdin is reduced to bilirubin (bile pigments) by biliverdin reductase 1. Senescent red cells are a major source of hemeproteins 2. Breakdown of heme to bilirubin occurs in macrophages of the MPS in liver and spleen 3. Unconjugated bilirubin is transported through the blood (complexed to albumin) to liver 4. Bilirubin is taken up via facilitated diffusion by liver and conjugated with glucuronic acid by bilirubin UDP – glucuronosyltransferase 5. Conjugated bilirubin is actively secreted into bile and then the intestines 6. In the intestines, glucuronic acid is removed by bacteria. The resulting bilirubin is converted to urobilinogen (colourless) 7. Some of the urobilinogen is reabsorbed from gut and enters portal blood 8. A portion of urobilinogen participates in enterohepatic urobilinogen 47 Emma notes 9. Reminder of urobilinogen is transported by blood to kidneys, where its converted to yellow urobilin and excreted, giving urine its characteristic colour Bilirubin, unique to mammals, appears to function at low levels as an antioxidant. In this role, its oxidized to biliverdin, which is then reduced by biliverdin reductase, regenerating bilirubin Cringler – Najjar I and II Gene mutation in the bilirubin UDP – glucuronosyltransferase which converts bilirubin into conjugated form Type I is most severe Unconjugated hyperbilirubinemia Gilbert syndrome A form of bilirubin UDP – glucuronosyltransferase deficiency Unconjugated hyperbilirubinemia Rotor syndrome Impaired hepatocellular storage of conjugated bilirubin à leaks into blood Conjugated hyperbilirubinemia Liver cells are not pigmented like in Dubin Johnsen Dubin – Johnsen syndrome Deficiency of protein required for conjugated bilirubin transport out of liver Conjugated hyperbilirubinemia centrilobular hepatocytes contain abnormal black pigment that may be derived from epinephrine Hemolytic jaundice Extensive hemolysis (sickle cell anemia or pyruvate kinase/G6PD deficiency) can cause production of bilirubin to be faster than it can be conjugated Unconjugated hyperbilirubinemia Urinary urobilinogen increased Urinary bilirubin absent Hepatocellular jaundice Damage to liver - Cirrhosis - Hepatitis Can cause unconjugated hyperbilirubinemia as result of decreased conjugation Obstructive jaundice Caused by obstruction of the common bile duct - Bile stones - Tumour Blocking the bile duct, preventing passage of CB into intestines Urinary urobilinogen increased Conjugated hyperbilirubinemia GI pain, nausea, and produce of stool that is pale, clay Urine darkens, stool may be pale, clay colour CB is excreted into urine ALT and AST plasma levels are elevated Urinary bilirubin present Urinary urobilinogen absent Conjugated hyper