ENSCI MIDTERM PDF
Document Details
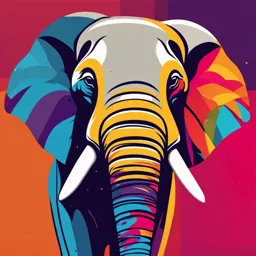
Uploaded by AlluringOklahomaCity7956
Tags
Summary
This document discusses the Scientific Revolution. It covers various aspects of astronomy from different cultures and eras, and explores theories about the structure of the universe, including the early Greek and Roman cosmologies. The document contains information about astronomers and their observations, such as the length of a year and the patterns of stars, concluding with a look into the history of astronomy and cosmology and introducing the works and ideas of key figures such as Copernicus and Galileo.
Full Transcript
SCIENTIFIC REVOLUTION Astronomy around the World Ancient Babylonian, Assyrian, and Egyptian astronomers knew the approximate length of the year. The Egyptians of 3000 years ago, for example, adopted a calendar based on a 365-day year. They kept careful track of the rising time o...
SCIENTIFIC REVOLUTION Astronomy around the World Ancient Babylonian, Assyrian, and Egyptian astronomers knew the approximate length of the year. The Egyptians of 3000 years ago, for example, adopted a calendar based on a 365-day year. They kept careful track of the rising time of the bright star Sirius in the predawn sky, which has a yearly cycle that corresponded with the flooding of the Nile River. The Chinese also had a working calendar; they determined the length of the year at about the same time as the Egyptians. The Chinese also recorded comets, bright meteors, and dark spots on the Sun. (Many types of astronomical objects were introduced in Science and the Universe: A Brief Tour. If you are not familiar with terms like comets and meteors, you may want to review that chapter.) Later, Chinese astronomers kept careful records of "guest stars"—those that are normally too faint to see but suddenly flare up to become visible to the unaided eye for a few weeks or months. We still use some of these records in studying stars that exploded a long time ago. The Mayan culture in Mexico and Central America developed a sophisticated calendar based on the planet Venus, and they made astronomical observations from sites dedicated to this purpose a thousand years ago. The Polynesians learned to navigate by the stars over hundreds of kilometers of open ocean—a skill that enabled them to colonize new islands far away from where they began. In Britain, before the widespread use of writing, ancient people used stones to keep track of the motions of the Sun and Moon. We still find some of the great stone circles they built for this purpose, dating from as far back as 2800 BCE. The best known of these is Stonehenge, which is discussed in Earth, Moon, and Sky. Early Greek and Roman Cosmology Our concept of the cosmos—its basic structure and origin—is called cosmology, a word with Greek roots. Before the invention of telescopes, humans had to depend on the simple evidence of their senses for a picture of the universe. The ancients developed cosmologies that combined their direct view of the heavens with a rich variety of philosophical and religious symbolism. At least 2000 years before Columbus, educated people in the eastern Mediterranean region knew Earth was round. Belief in a spherical Earth may have stemmed from the time of Pythagoras, a philosopher and mathematician who lived 2500 years ago. He believed circles and spheres to be "perfect forms" and suggested that Earth should therefore be a sphere. As evidence that the gods liked spheres, the Greeks cited the fact that the Moon is a sphere, using evidence we describe later. The writings of Aristotle (384–322 BCE), the tutor of Alexander the Great, summarize many of the ideas of his day. They describe how the progression of the Moon’s phases—its apparent changing shape—results from our seeing different portions of the Moon’s sunlit hemisphere as the month goes by (see Earth, Moon, and Sky). Aristotle also knew that the Sun has to be farther away from Earth than is the Moon because occasionally the Moon passed exactly between Earth and the Sun and hid the Sun temporarily from view. We call this a solar eclipse. Aristotle cited convincing arguments that Earth must be round. First is the fact that as the Moon enters or emerges from Earth’s shadow during an eclipse of the Moon, the shape of the shadow seen on the Moon is always round (Figure 1). Only a spherical object always produces a round shadow. If Earth were a disk, for example, there would be some occasions when the sunlight would strike it edge-on and its shadow on the Moon would be a line. As a second argument, Aristotle explained that travelers who go south a significant distance are able to observe stars that are not visible farther north. And the height of the North Star—the star nearest the north celestial pole—decreases as a traveler moves south. On a flat Earth, everyone would see the same stars overhead. The only possible explanation is that the traveler must have moved over a curved surface on Earth, showing stars from a different angle. (See "How Do We Know Earth Is Round?" below for more ideas on proving Earth is round.) One Greek thinker, Aristarchus of Samos (310–230 BCE), even suggested that Earth was moving around the Sun, but Aristotle and most of the ancient Greek scholars rejected this idea. One of the reasons for their conclusion was the thought that if Earth moved about the Sun, they would be observing the stars from different places along Earth’s orbit. As Earth moved along, nearby stars should shift their positions in the sky relative to more distant stars. In a similar way, we see foreground objects appear to move against a more distant background whenever we are in motion. When we ride on a train, the trees in the foreground appear to shift their position relative to distant hills as the train rolls by. Unconsciously, we use this phenomenon all of the time to estimate distances around us. The apparent shift in the direction of an object as a result of the motion of the observer is called parallax. We call the shift in the apparent direction of a star due to Earth’s orbital motion stellar parallax. The Greeks made dedicated efforts to observe stellar parallax, even enlisting the aid of Greek soldiers with the clearest vision, but to no avail. The brighter (and presumably nearer) stars just did not seem to shift as the Greeks observed them in the spring and then again in the fall (when Earth is on the opposite side of the Sun). This meant either that Earth was not moving or that the stars had to be so tremendously far away that the parallax shift was immeasurably small. A cosmos of such enormous extent required a leap of imagination that most ancient philosophers were not prepared to make, so they retreated to the safety of the Earth-centered view, which would dominate Western thinking for nearly two millennia. How Do We Know Earth Is Round? In addition to the two ways (from Aristotle’s writings) discussed in this chapter, you might also reason as follows: Let’s watch a ship leave its port and sail into the distance on a clear day. On a flat Earth, we would just see the ship get smaller and smaller as it sails away. But this isn’t what we actually observe. Instead, ships sink below the horizon, with the hull disappearing first and the mast remaining visible for a while longer. Eventually, only the top of the mast can be seen as the ship sails around the curvature of Earth. Finally, the ship disappears under the horizon. The International Space Station circles Earth once every 90 minutes or so. Photographs taken from the shuttle and other satellites show that Earth is round from every perspective. Suppose you made a friend in each time zone of Earth. You call all of them at the same hour and ask, "Where is the Sun?" On a flat Earth, each caller would give you roughly the same answer. But on a round Earth you would find that, for some friends, the Sun would be high in the sky whereas for others it would be rising, setting, or completely out of sight (and this last group of friends would be upset with you for waking them up). Measurement of Earth by Eratosthenes The Greeks not only knew Earth was round, but also they were able to measure its size. The first fairly accurate determination of Earth’s diameter was made in about 200 BCE by Eratosthenes (276–194 BCE), a Greek living in Alexandria, Egypt. His method was a geometric one, based on observations of the Sun. The Sun is so distant from us that all the light rays that strike our planet approach us along essentially parallel lines. To see why, look at Figure 2. Take a source of light near Earth—say, at position A. Its rays strike different parts of Earth along diverging paths. From a light source at B, or at C (which is still farther away), the angle between rays that strike opposite parts of Earth is smaller. The more distant the source, the smaller the angle between the rays. For a source infinitely distant, the rays travel along parallel lines. Figure 2: Light Rays from Space. The more distant an object, the more nearly parallel the rays of light coming from it. Of course, the Sun is not infinitely far away, but given its distance of 150 million kilometers, light rays striking Earth from a point on the Sun diverge from one another by an angle far too small to be observed with the unaided eye. As a consequence, if people all over Earth who could see the Sun were to point at it, their fingers would, essentially, all be parallel to one another. (The same is also true for the planets and stars—an idea we will use in our discussion of how telescopes work.) Eratosthenes was told that on the first day of summer at Syene, Egypt (near modern Aswan), sunlight struck the bottom of a vertical well at noon. This indicated that the Sun was directly over the well—meaning that Syene was on a direct line from the center of Earth to the Sun. At the corresponding time and date in Alexandria, Eratosthenes observed the shadow a column made and saw that the Sun was not directly overhead, but was slightly south of the zenith, so that its rays made an angle with the vertical equal to about 1/50 of a circle (7°). Because the Sun’s rays striking the two cities are parallel to one another, why would the two rays not make the same angle with Earth’s surface? Eratosthenes reasoned that the curvature of the round Earth meant that "straight up" was not the same in the two cities. And the measurement of the angle in Alexandria, he realized, allowed him to Figure out the size of Earth. Alexandria, he saw, must be 1/50 of Earth’s circumference north of Syene (Figure 3). Alexandria had been measured to be 5000 stadia north of Syene. (The stadium was a Greek unit of length, derived from the length of the racetrack in a stadium.) Eratosthenes thus found that Earth’s circumference must be 50 × 5000, or 250,000 stadia. It is not possible to evaluate precisely the accuracy of Eratosthenes solution because there is doubt about which of the various kinds of Greek stadia he used as his unit of distance. If it was the common Olympic stadium, his result is about 20% too large. According to another interpretation, he used a stadium equal to about 1/6 kilometer, in which case his Figure was within 1% of the correct value of 40,000 kilometers. Even if his measurement was not exact, his success at measuring the size of our planet by using only shadows, sunlight, and the power of human thought was one of the greatest intellectual achievements in history. Hipparchus and Precession Perhaps the greatest astronomer of antiquity was Hipparchus, born in Nicaea in what is present-day Turkey. He erected an observatory on the island of Rhodes around 150 BCE, when the Roman Republic was expanding its influence throughout the Mediterranean region. There he measured, as accurately as possible, the positions of objects in the sky, compiling a pioneering star catalog with about 850 entries. He designated celestial coordinates for each star, specifying its position in the sky, just as we specify the position of a point on Earth by giving its latitude and longitude. He also divided the stars into apparent magnitudes according to their apparent brightness. He called the brightest ones "stars of the first magnitude"; the next brightest group, "stars of the second magnitude"; and so forth. This rather arbitrary system, in modified form, still remains in use today (although it is less and less useful for professional astronomers). By observing the stars and comparing his data with older observations, Hipparchus made one of his most remarkable discoveries: the position in the sky of the north celestial pole had altered over the previous century and a half. Hipparchus deduced correctly that this had happened not only during the period covered by his observations, but was in fact happening all the time: the direction around which the sky appears to rotate changes slowly but continuously. Recall from the section on celestial poles and the celestial equator that the north celestial pole is just the projection of Earth’s North Pole into the sky. If the north celestial pole is wobbling around, then Earth itself must be doing the wobbling. Today, we understand that the direction in which Earth’s axis points does indeed change slowly but regularly—a motion we call precession. If you have ever watched a spinning top wobble, you observed a similar kind of motion. The top’s axis describes a path in the shape of a cone, as Earth’s gravity tries to topple it (Figure 4). Figure 4: Precession. Just as the axis of a rapidly spinning top wobbles slowly in a circle, so the axis of Earth wobbles in a 26,000-year cycle. Today the north celestial pole is near the star Polaris, but about 5000 years ago it was close to a star called Thuban, and in 14,000 years it will be closest to the star Vega. Because our planet is not an exact sphere, but bulges a bit at the equator, the pulls of the Sun and Moon cause it to wobble like a top. It takes about 26,000 years for Earth’s axis to complete one circle of precession. As a result of this motion, the point where our axis points in the sky changes as time goes on. While Polaris is the star closest to the north celestial pole today (it will reach its closest point around the year 2100), the star Vega in the constellation of Lyra will be the North Star in 14,000 years Ptolemy’s Model of the Solar System The last great astronomer of the Roman era was Claudius Ptolemy (or Ptolemaeus), who flourished in Alexandria in about the year 140. He wrote a mammoth compilation of astronomical knowledge, which today is called by its Arabic name, Almagest (meaning "The Greatest"). Almagest does not deal exclusively with Ptolemy’s own work; it includes a discussion of the astronomical achievements of the past, principally those of Hipparchus. Today, it is our main source of information about the work of Hipparchus and other Greek astronomers. Ptolemy’s most important contribution was a geometric representation of the solar system that predicted the positions of the planets for any desired date and time. Hipparchus, not having enough data on hand to solve the problem himself, had instead amassed observational material for posterity to use. Ptolemy supplemented this material with new observations of his own and produced a cosmological model that endured more than a thousand years, until the time of Copernicus. The complicating factor in explaining the motions of the planets is that their apparent wandering in the sky results from the combination of their own motions with Earth’s orbital revolution. As we watch the planets from our vantage point on the moving Earth, it is a little like watching a car race while you are competing in it. Sometimes opponents’ cars pass you, but at other times you pass them, making them appear to move backward for a while with respect to you. This retrograde simulation of Mars illustrates the motion of Mars as seen from Earth as well as Earth’s retrograde motion as seen from Mars. There is also an animation of the movement of the two planets relative to each other that creates the appearance of this motion. Normally, planets move eastward in the sky over the weeks and months as they orbit the Sun, but from positions B to D in Figure 5, as Earth passes the planets in our example, it appears to drift backward, moving west in the sky. Even though it is actually moving to the east, the faster-moving Earth has overtaken it and seems, from our perspective, to be leaving it behind. As Earth rounds its orbit toward position E, the planet again takes up its apparent eastward motion in the sky. The temporary apparent westward motion of a planet as Earth swings between it and the Sun is called retrograde motion. Such backward motion is much easier for us to understand today, now that we know Earth is one of the moving planets and not the unmoving center of all creation. But Ptolemy was faced with the far more complex problem of explaining such motion while assuming a stationary Earth. Furthermore, because the Greeks believed that celestial motions had to be circles, Ptolemy had to construct his model using circles alone. To do it, he needed dozens of circles, some moving around other circles, in a complex structure that makes a modern viewer dizzy. But we must not let our modern judgment cloud our admiration for Ptolemy’s achievement. In his day, a complex universe centered on Earth was perfectly reasonable and, in its own way, quite beautiful. However, as Alfonso X, the King of Castile, was reported to have said after having the Ptolemaic system of planet motions explained to him, "If the Lord Almighty had consulted me before embarking upon Creation, I should have recommended something simpler." Ptolemy solved the problem of explaining the observed motions of planets by having each planet revolve in a small orbit called an epicycle. The center of the epicycle then revolved about Earth on a circle called a deferent (Figure 6). When the planet is at position x in Figure 6 on the epicycle orbit, it is moving in the same direction as the center of the epicycle; from Earth, the planet appears to be moving eastward. When the planet is at y, however, its motion is in the direction opposite to the motion of the epicycle’s center around Earth. By choosing the right combination of speeds and distances, Ptolemy succeeded in having the planet moving westward at the correct speed and for the correct interval of time, thus replicating retrograde motion with his model. However, we shall see in Orbits and Gravity that the planets, like Earth, travel about the Sun in orbits that are ellipses, not circles. Their actual behavior cannot be represented accurately by a scheme of uniform circular motions. In order to match the observed motions of the planets, Ptolemy had to center the deferent circles, not on Earth, but at points some distance from Earth. In addition, he introduced uniform circular motion around yet another axis, called the equant point. All of these considerably complicated his scheme. It is a tribute to the genius of Ptolemy as a mathematician that he was able to develop such a complex system to account successfully for the observations of planets. It may be that Ptolemy did not intend for his cosmological model to describe reality, but merely to serve as a mathematical representation that allowed him to predict the positions of the planets at any time. Whatever his thinking, his model, with some modifications, was eventually accepted as authoritative in the Muslim world and (later) in Christian Europe. Ancient Greeks such as Aristotle recognized that Earth and the Moon are spheres, and understood the phases of the Moon, but because of their inability to detect stellar parallax, they rejected the idea that Earth moves. Eratosthenes measured the size of Earth with surprising precision. Hipparchus carried out many astronomical observations, making a star catalog, defining the system of stellar magnitudes, and discovering precession from the apparent shift in the position of the north celestial pole. Ptolemy of Alexandria summarized classic astronomy in his Almagest; he explained planetary motions, including retrograde motion, with remarkably good accuracy using a model centered on Earth. This geocentric model, based on combinations of uniform circular motion using epicycles, was accepted as authority for more than a thousand years. Glossary apparent magnitude: a measure of how bright a star looks in the sky; the larger the number, the dimmer the star appears to us cosmology: the study of the organization and evolution of the universe epicycle: the circular orbit of a body in the Ptolemaic system, the center of which revolves about another circle (the deferent) parallax: the apparent displacement of a nearby star that results from the motion of Earth around the Sun precession (of Earth): the slow, conical motion of Earth’s axis of rotation caused principally by the gravitational pull of the Moon and Sun on Earth’s equatorial bulge retrograde motion: the apparent westward motion of a planet on the celestial sphere or with respect to the stars - The Scientific Revolution, which spanned roughly from the late 16th to the 18th century, marked a pivotal turning point in human thought and inquiry, characterized by profound shifts in the understanding of nature and the universe. This period not only redefined the methodologies of science but also laid the foundations for modern science as we know it today. - The revolution is distinguished by significant figures such as Nicolaus Copernicus, who introduced a heliocentric model of the universe, challenging the long-held geocentric views of Aristotle and Ptolemy. This paradigm shift was further expanded upon by Johannes Kepler, who formulated the laws of planetary motion, and Galileo Galilei, whose use of the telescope provided concrete observations that contradicted established beliefs. Their works were instrumental in moving from a reliance on authority and dogma toward empirical evidence and experimentation. - One of the central themes of the Scientific Revolution is the rise of the scientific method, championed by philosophers like Francis Bacon and René Descartes. Bacon's emphasis on inductive reasoning and observation contrasted sharply with the deductive reasoning prevalent in scholastic traditions. Meanwhile, Descartes' analytical approach and philosophical inquiries about the nature of existence and knowledge greatly influenced subsequent scientific thought. - The revolution also had profound impacts beyond the field of science. It initiated a new ethos of inquiry that permeated through philosophy, religion, and society as a whole. The challenge to ecclesiastical authority and the embrace of rationalism paved the way for the Enlightenment, setting the stage for modern democratic ideals and the questioning of traditional power structures. - However, the Scientific Revolution was not without its contradictions and challenges. While it heralded the triumph of reason and empiricalism, it also led to conflicts, such as Galileo's infamous trial by the Inquisition. This tension between science and religion persists today, highlighting the ongoing struggle between established belief systems and new discoveries. - The Scientific Revolution was a momentous event that reshaped the trajectory of human understanding. Its legacy can still be felt in contemporary scientific practices, critical thinking, and the pursuit of knowledge. As we navigate an increasingly complex world, reflecting upon the lessons of this era encourages a commitment to inquiry, skepticism, and the relentless pursuit of truth. The Scientific Revolution not only changed how we view the universe but fundamentally altered our place within it, inspiring generations of thinkers to explore the unknown. COPERNICUS AND GALILEO - Astronomy made no major advances in strife-torn medieval Europe. The birth and expansion of Islam after the seventh century led to a flowering of Arabic and Jewish cultures that preserved, translated, and added to many of the astronomical ideas of the Greeks. Many of the names of the brightest stars, for example, are today taken from the Arabic, as are such astronomical terms as "zenith." Nicolaus Copernicus (1473–1543) - As European culture began to emerge from its long, dark age, trading with Arab countries led to a rediscovery of ancient texts such as Almagest and to a reawakening of interest in astronomical questions. This time of rebirth (in French, "renaissance") in astronomy was embodied in the work of Copernicus. - Copernicus was a cleric and scientist who played a leading role in the emergence of modern science. Although he could not prove that Earth revolves about the Sun, he presented such compelling arguments for this idea that he turned the tide of cosmological thought and laid the foundations upon which Galileo and Kepler so effectively built in the following century. - Nicolaus Copernicus was born in Torun, a mercantile town along the Vistula River. - His training was in law and medicine, but his main interests were astronomy and mathematics. - His great contribution to science was a critical reappraisal of the existing theories of planetary motion and the development of a new Sun-centered, or heliocentric, model of the solar system. - Copernicus concluded that Earth is a planet and that all the planets circle the Sun. Only the Moon orbits Earth - Copernicus described his ideas in detail in his book De Revolutionibus Orbium Coelestium (On the Revolution of Celestial Orbs), published in 1543, the year of his death. - He began with several assumptions that were common in his time, such as the idea that the motions of the heavenly bodies must be made up of combinations of uniform circular motions. But he did not assume (as most people did) that Earth had to be in the center of the universe, and he presented a defense of the heliocentric system that was elegant and persuasive. His ideas, although not widely accepted until more than a century after his death, were much discussed among scholars and, ultimately, had a profound influence on the course of world history. - He also reasoned that the apparent rotation of the celestial sphere could be explained by assuming that Earth rotates while the celestial sphere is stationary. To the objection that if Earth rotated about an axis it would fly into pieces, Copernicus answered that if such motion would tear Earth apart, the still faster motion of the much larger celestial sphere required by the geocentric hypothesis would be even more devastating. Heliocentric Model - The most important idea in Copernicus’ De Revolutionibus is that Earth is one of six (then-known) planets that revolve about the Sun. - Using this concept, he was able to work out the correct general picture of the solar system. He placed the planets, starting nearest the Sun, in the correct order: Mercury, Venus, Earth, Mars, Jupiter, and Saturn. - Further, he deduced that the nearer a planet is to the Sun, the greater its orbital speed. - With his theory, he was able to explain the complex retrograde motions of the planets without epicycles and to work out a roughly correct scale for the solar system. Copernicus could not prove that Earth revolves about the Sun. In fact, with some adjustments, the old Ptolemaic system could have accounted, as well, for the motions of the planets in the sky. But Copernicus pointed out that the Ptolemaic cosmology was clumsy and lacking the beauty and symmetry of its successor. Galileo Galilei (1564–1642). - Galileo advocated that we perform experiments or make observations to ask nature its ways. When Galileo turned the telescope to the sky, he found things were not the way philosophers had supposed.\ - Many of the modern scientific concepts of observation, experimentation, and the testing of hypotheses through careful quantitative measurements were pioneered by a man who lived nearly a century after Copernicus. Galileo Galilei (Figure 4), a contemporary of Shakespeare, was born in Pisa. Like Copernicus, he began training for a medical career, but he had little interest in the subject and later switched to mathematics. He held faculty positions at the University of Pisa and the University of Padua, and eventually became mathematician to the Grand Duke of Tuscany in Florence. - Galileo’s greatest contributions were in the field of mechanics, the study of motion and the actions of forces on bodies. It was familiar to all persons then, as it is to us now, that if something is at rest, it tends to remain at rest and requires some outside influence to start it in motion. Rest was thus generally regarded as the natural state of matter. Galileo showed, however, that rest is no more natural than motion. - If an object is slid along a rough horizontal floor, it soon comes to rest because friction between it and the floor acts as a retarding force. However, if the floor and the object are both highly polished, the object, given the same initial speed, will slide farther before stopping. On a smooth layer of ice, it will slide farther still. Galileo reasoned that if all resisting effects could be removed, the object would continue in a steady state of motion indefinitely. He argued that a force is required not only to start an object moving from rest but also to slow down, stop, speed up, or change the direction of a moving object. You will appreciate this if you have ever tried to stop a rolling car by leaning against it, or a moving boat by tugging on a line. - Galileo also studied the way objects accelerate—change their speed or direction of motion. Galileo watched objects as they fell freely or rolled down a ramp. He found that such objects accelerate uniformly; that is, in equal intervals of time they gain equal increments in speed. Galileo formulated these newly found laws in precise mathematical terms that enabled future experimenters to predict how far and how fast objects would move in various lengths of time. - In theory, if Galileo is right, a feather and a hammer, dropped at the same time from a height, should land at the same moment. On Earth, this experiment is not possible because air resistance and air movements make the feather flutter, instead of falling straight down, accelerated only by the force of gravity. For generations, physics teachers had said that the place to try this experiment is somewhere where there is no air, such as the Moon. In 1971, Apollo 15 astronaut David Scott took a hammer and feather to the Moon and tried it, to the delight of physics nerds everywhere. NASA provides the video of the hammer and feather as well as a brief explanation. - Sometime in the 1590s, Galileo adopted the Copernican hypothesis of a heliocentric solar system. In Roman Catholic Italy, this was not a popular philosophy, for Church authorities still upheld the ideas of Aristotle and Ptolemy, and they had powerful political and economic reasons for insisting that Earth was the center of creation. Galileo not only challenged this thinking but also had the audacity to write in Italian rather than scholarly Latin, and to lecture publicly on those topics. For him, there was no contradiction between the authority of the Church in matters of religion and morality, and the authority of nature (revealed by experiments) in matters of science. It was primarily because of Galileo and his "dangerous" opinions that, in 1616, the Church issued a prohibition decree stating that the Copernican doctrine was "false and absurd" and not to be held or defended. Galileo’s Astronomical Observations It is not certain who first conceived of the idea of combining two or more pieces of glass to produce an instrument that enlarged images of distant objects, making them appear nearer. The first such "spyglasses" (now called telescopes) that attracted much notice were made in 1608 by the Dutch spectacle maker Hans Lippershey (1570–1619). Galileo heard of the discovery and, without ever having seen an assembled telescope, constructed one of his own with a three-power magnification (3×), which made distant objects appear three times nearer and larger (Figure 5). Figure 5: Telescope Used by Galileo. The telescope has a wooden tube covered with paper and a lens 26 millimeters across. - On August 25, 1609, Galileo demonstrated a telescope with a magnification of 9× to government officials of the city-state of Venice. By a magnification of 9×, we mean the linear dimensions of the objects being viewed appeared nine times larger or, alternatively, the objects appeared nine times closer than they really were. There were obvious military advantages associated with a device for seeing distant objects. For his invention, Galileo’s salary was nearly doubled, and he was granted lifetime tenure as a professor. (His university colleagues were outraged, particularly because the invention was not even original.) - Others had used the telescope before Galileo to observe things on Earth. But in a flash of insight that changed the history of astronomy, Galileo realized that he could turn the power of the telescope toward the heavens. Before using his telescope for astronomical observations, Galileo had to devise a stable mount and improve the optics. He increased the magnification to 30×. Galileo also needed to acquire confidence in the telescope. - At that time, human eyes were believed to be the final arbiter of truth about size, shape, and color. Lenses, mirrors, and prisms were known to distort distant images by enlarging, reducing, or inverting them, or spreading the light into a spectrum (rainbow of colors). Galileo undertook repeated experiments to convince himself that what he saw through the telescope was identical to what he saw up close. Only then could he begin to believe that the miraculous phenomena the telescope revealed in the heavens were real. - Beginning his astronomical work late in 1609, Galileo found that many stars too faint to be seen with the unaided eye became visible with his telescope. In particular, he found that some nebulous blurs resolved into many stars, and that the Milky Way—the strip of whiteness across the night sky—was also made up of a multitude of individual stars. - Examining the planets, Galileo found four moons revolving about Jupiter in times ranging from just under 2 days to about 17 days. This discovery was particularly important because it showed that not everything has to revolve around Earth. Furthermore, it demonstrated that there could be centers of motion that are themselves in motion. Defenders of the geocentric view had argued that if Earth was in motion, then the Moon would be left behind because it could hardly keep up with a rapidly moving planet. Yet, here were Jupiter’s moons doing exactly that. (To recognize this discovery and honor his work, NASA named a spacecraft that explored the Jupiter system Galileo.) - With his telescope, Galileo was able to carry out the test of the Copernican theory mentioned earlier, based on the phases of Venus. Within a few months, he had found that Venus goes through phases like the Moon, showing that it must revolve about the Sun, so that we see different parts of its daylight side at different times (see Figure 3.) These observations could not be reconciled with Ptolemy’s model, in which Venus circled about Earth. In Ptolemy’s model, Venus could also show phases, but they were the wrong phases in the wrong order from what Galileo observed. - Galileo also observed the Moon and saw craters, mountain ranges, valleys, and flat, dark areas that he thought might be water. These discoveries showed that the Moon might be not so dissimilar to Earth—suggesting that Earth, too, could belong to the realm of celestial bodies. For more information about the life and work of Galileo, see the Galileo Project at Rice University. - After Galileo’s work, it became increasingly difficult to deny the Copernican view, and Earth was slowly dethroned from its central position in the universe and given its rightful place as one of the planets attending the Sun. Initially, however, Galileo met with a great deal of opposition. The Roman Catholic Church, still reeling from the Protestant Reformation, was looking to assert its authority and chose to make an example of Galileo. He had to appear before the Inquisition to answer charges that his work was heretical, and he was ultimately condemned to house arrest. His books were on the Church’s forbidden list until 1836, although in countries where the Roman Catholic Church held less sway, they were widely read and discussed. Not until 1992 did the Catholic Church admit publicly that it had erred in the matter of censoring Galileo’s ideas. - The new ideas of Copernicus and Galileo began a revolution in our conception of the cosmos. It eventually became evident that the universe is a vast place and that Earth’s role in it is relatively unimportant. The idea that Earth moves around the Sun like the other planets raised the possibility that they might be worlds themselves, perhaps even supporting life. As Earth was demoted from its position at the center of the universe, so, too, was humanity. The universe, despite what we may wish, does not revolve around us. - Most of us take these things for granted today, but four centuries ago such concepts were frightening and heretical for some, immensely stimulating for others. The pioneers of the Renaissance started the European world along the path toward science and technology that we still read today. For them, nature was rational and ultimately knowable, and experiments and observations provided the means to reveal its secrets. Observing the Planets - At most any time of the night, and at any season, you can spot one or more bright planets in the sky. All five of the planets known to the ancients—Mercury, Venus, Mars, Jupiter, and Saturn—are more prominent than any but the brightest stars, and they can be seen even from urban locations if you know where and when to look. One way to tell planets from bright stars is that planets twinkle less. - Venus, which stays close to the Sun from our perspective, appears either as an "evening star" in the west after sunset or as a "morning star" in the east before sunrise. It is the brightest object in the sky after the Sun and Moon. It far outshines any real star, and under the most favorable circumstances, it can even cast a visible shadow. Some young military recruits have tried to shoot Venus down as an approaching enemy craft or UFO. - Mars, with its distinctive red color, can be nearly as bright as Venus is when close to Earth, but normally it remains much less conspicuous. Jupiter is most often the second-brightest planet, approximately equaling in brilliance the brightest stars. Saturn is dimmer, and it varies considerably in brightness, depending on whether its large rings are seen nearly edge-on (faint) or more widely opened (bright). - Mercury is quite bright, but few people ever notice it because it never moves very far from the Sun (it’s never more than 28° away in the sky) and is always seen against bright twilight skies. - True to their name, the planets "wander" against the background of the "fixed" stars. Although their apparent motions are complex, they reflect an underlying order upon which the heliocentric model of the solar system, as described in this chapter, was based. The positions of the planets are often listed in newspapers (sometimes on the weather page), and clear maps and guides to their locations can be found each month in such magazines as Sky & Telescope and Astronomy (available at most libraries and online). There are also a number of computer programs and phone and tablet apps that allow you to display where the planets are on any night. - Nicolaus Copernicus introduced heliocentric cosmology to Renaissance Europe in his book De Revolutionibus. Although he retained the Aristotelian idea of uniform circular motion, Copernicus suggested that Earth is a planet and that the planets all circle about the Sun, dethroning Earth from its position at the center of the universe. Galileo was the father of both modern experimental physics and telescopic astronomy. He studied the acceleration of moving objects and, in 1610, began telescopic observations, discovering the nature of the Milky Way, the large-scale features of the Moon, the phases of Venus, and four moons of Jupiter. Although he was accused of heresy for his support of heliocentric cosmology, Galileo is credited with observations and brilliant writings that convinced most of his scientific contemporaries of the reality of the Copernican theory. SCIENTISM 1. The Nature and Critique of Scientism: The text defines scientism as a belief system that seeks to apply the methods of natural science to all areas of human life, often reducing complex human experiences and ethical considerations to mere materialistic explanations. Lewis strongly criticizes this ideology, arguing that it can lead to a narrow understanding of human experience. 2. Abuses of Science: Examples of scientific misapplication include Scientific Socialism, which Marxists claimed was based on scientific principles, and the racial theories that gained support in Nazi Germany. Lewis emphasizes that the misuse of scientific terminology and methods can lead to catastrophic societal consequences. 3. Similarities Between Science and Magic: Lewis suggests that science and magic are "twins" in three primary ways: - Functioning as Religion: Both can provide a sense of meaning and significance in life. - Encouragement of Gullibility: People can blindly accept scientific claims without critical examination, mirroring how magical thinking can lead to unquestioning belief. - Quest for Power: Both science and magic can be utilized to exert control over the world and others, risking ethical violations in the process. 4. Understanding Scientocracy: Scientocracy refers to a governmental or social system that claims authority based on scientific knowledge or expertise. Lewis warns of the dangers posed by a ruling class of experts who might dictate policy without sufficient ethical constraints. 5. Science's Potential for Good: While Lewis acknowledges that science can lead to significant advancements—such as medical breakthroughs and technological progress—he stresses the necessity of ethical considerations to ensure that these advances serve humanity rather than undermine it. 6. Limitation of Science: The text emphasizes that science, while powerful, does not provide moral or ethical guidance. Understanding how things work is distinct from questioning what ought to be done with that knowledge, a distinction that is crucial for effective public policy. 7. Intrinsic Dangers of Science: The text highlights the potential for science to be dangerous when it becomes dogmatic or unquestioned. Lewis feared that the unchecked pursuit of scientific knowledge could lead to authoritarian practices and violations of human dignity. 8. Rescuing Science from Scientism: The solution proposed involves creating a science that respects human rights and acknowledges ethical considerations beyond mere empirical investigation, ensuring that scientific inquiry serves humanity as a whole. QUESTIONS: 1. What is scientism? - Scientism is the ideology that advocates for the application of scientific methods to all domains of human life and thought, often reducing complex human experiences to purely materialistic explanations. 2. What are examples of abuses of science? - Notable examples include Scientific Socialism, the racial theories employed by the Nazis, and the misuse of psychoanalysis in Freud's work, where scientific jargon was manipulated to justify unethical practices 3. In what ways are science and magic "twins"? - Both can function as alternative religions offering meaning. Both encourage gullibility when claims are accepted without skepticism. Both can represent a quest for power over nature and humanity. 4. What is scientocracy? - Scientocracy is a form of governance where authority is derived from scientific knowledge, often leading to a ruling elite of scientists and experts who impose their views on society, sometimes disregarding ethical considerations. 5. How can science be good? - Science can be beneficial by driving advancements in health, technology, and understanding of the natural world, provided it is guided by ethical principles that prioritize human dignity and welfare. 6. Why is science alone not sufficient to solve society's problems? - Science does not address the moral and ethical dimensions of decision-making, which are essential for shaping public policy and ensuring that scientific advancements align with societal values and needs. 7. Why is science dangerous? - Science can be dangerous when it fosters an unquestioning adherence to perceived truths, leading to authoritarianism and the potential for misuse of scientific advancements that undermine individual rights and freedoms. 8. How can science be rescued from scientism? - By fostering a science that incorporates ethical considerations and recognizes the complexities of human experience, ensuring that scientific pursuits serve to enhance human life rather than control it. MODERN TECHNOLOGY He begins the essay by simplifying technology into its fundamental being in an attempt to uncover its essence. This is accomplished through defining technology in relation to explanations of instrumentality, causality and revealing: Instrumentality: Technology is an instrument humans use as “a means to an end” and “a human activity” to be mastered; it is a way of getting things done (4/5) Causality: Instruments are developed to cause, bring forth, occasion and presence an end (6) Revealing: bringing forth something; unconcealing something that was concealed (11) According to Heidegger, the essence of technology is a way of revealing. He considers the origin of the word “technology”, referring to both technique and knowledge. This understanding of technology extends the definition of the word from merely instrumental, to revealing. In contrast to the revealing definition of technology, Heidegger presents modern technology as a “challenge” to world’s resources through exploitative methods, however he states that it also reveals. Standing Reserve, Enframing and Free Relationship Standing reserve is a source of raw materials and reflects interdependence of resources and processes (natural and human) necessary for the creation of desired products. He states that “everywhere, everything is ordered to stand by, to be immediately at hand, indeed to stand there just so that it may be on call for a further ordering” (17). Heidegger discusses product life cycle processes to explain the relationship between humans and world resources. He refers to this relationship as enframing, which is an orientation or attitude humans have to the world. Humanity’s enframing is the essence of technology, “the way in which the real reveals itself as the standing-reserve” (23). Once we realize this orientation, the free relationship that Heidegger mentions at the beginning of the essay can be established. Heidegger believes that enframing is both a “danger” and a “saving power”. It is a “danger” as humanity can be destructive with too much control over nature and lose sight of the world revealing itself, and knowing truth. Enframing is a “saving power” as humanity’s orientation to the world demonstrates that we are responsible for it. By reorienting our relationship with nature, Heidegger believes that our actions towards the world will not be destructive. Heidegger proposes that humanity should try to view the world from an artist or poet’s perspective, and take the world as it reveals itself, as it is. In this way, we prevent the dangers of enframing and develop a free (and questioning) relationship with the technology in our lives. Since “everywhere we remain unfree and chained to technology, whether we passionately affirm or deny it.” (4). Focus questions for discussion posts on Vista: 1. How would you apply Heidegger’s assessment of “technology” in general to modern information technology, and to communications technology in particular? 2. How would you characterize the dangers of modern technology? How do your concerns compare with Heidegger’s? 3. How do we currently relate to technology? Summary: Primer for Defining and Theorizing Technology in Education Pt. 2 Petrina, S. & Feng, F. Sociotechnical Theories Defining and Theorizing Technology, pt. 2, begins with charting the development of sociotechnical theories. Sociotechnical theories are based on theories developed by Marx (industry and technology) and Weber (bureaucracy and rationality). Sociotechnical theories relate to the connections between humans and technologies as well as technological use and organization. During the 1950s, the definition focused on the interface between the social and the technical, and then the cybernetic and systems theory developed to record human and machine behaviour. Eventually cybernetic ideas shifted from a focus on human and machine behaviour to the relationship between the cultural, social and technical components. Some theories focus on technological determinism (Frankfurt School), while others argued that there are many facets of human nature that are independent of technology (Tavistock Institute of Human Relations). During the 1950s and 1960s, theorists such as Ellul and Althusser did not put humans above technology, as humans were completely integrated with technology. During the 1980s and 1990s, the idea of contextualism developed which highlights the interdependencies of cultural, social and psychological factors with technology. Technology shapes the context and the context shapes the technology at the same time. The idea of interactionism also developed in the 1980s and 1990s, which states that technologies and other systems develop together at the same time. Hybridity theories of the 1990s focus on the idea that “human-machine relations are never fully harmonious nor antagonistic” (4). Theorizing Cognition in a New Age of Technology The reports about cognition and technology that were reviewed were based on the idea that “learners are active in the construction of knowledge” (5). In the 1980s and 1990s, constructivists studying how humans learn were criticized for minimizing technology as tools for learning, rather than as integral to the process. Cognitive science began to be developed in the 1940s and 1950s as biology and technology converged. Following the 1950s there were shifts in sociotechnology approaches to explain “human-technology interaction” (7), then in the 1980s theories shifted again to “actor-network theory, cultural studies, cyborgs, hybridity and postsocial relations with objects and the world” (7), merging the foundations of learning with the foundations of technology design. In the 1990s, cognitive theory shifted “from individual minds (constructivism) to collective activity (situated cognition) to activity systems” (7). Theorists then positioned technologies as having a central role in collective cognition that is situated in an activity system. Defining technology, according to Heidegger ‘Technology’ is one of those words that’s so commonplace, yet it’s hard to define. Computers and smart devices are technologies, but so are books and notepads. Indeed, the definition of technology may span from simple tools and utensils (hammers and spoons) to powerful machines and media (car factories and artificial intelligence). How are we to say what technology is precisely? Enter Martin Heidegger. Heidegger on the “essence” of modern technology In his essay “The Question Concerning Technology,” Heidegger asks, what is the “essence” of technology? Directing that question at modern technology, especially powerful machines, he gives the following answer: “enframing.” Let’s unpack the meaning of that word. Heidegger on “enframing” For Heidegger, “enframing” [Gestell in German] is using technology to turn nature into a resource for efficient use. Modern technology, says Heidegger, lets us isolate nature and treat it as a “standing reserve” [Bestand]—that is, a resource to be stored for later utility. As an example, he gives the hydroelectric plant, which isolates a river and transforms it into a power source. In the enigmatic words of Heidegger, this isolation and transformation “sets upon nature … in the sense of challenging it.” This setting-upon that challenges forth the energies of nature is an expediting [Fordern], and in two ways. It expedites in that it unlocks and exposes. Yet that expediting is always itself directed from the beginning toward furthering something else, i.e., toward driving on the maximum yield at the minimum expense. Whoa, say what? Told you he was difficult to read. Allow me to translate. Essentially, Heiddeger is telling us technology is not just a thing. It’s how we relate to the world. Thus, it’s no surprise that different technologies are, in effect, different ways of relating to reality. In particular, modern technologies—namely, powerful machines—are expedient ways of conquering the world, because they objectify nature and turn it into a resource that can be quantified, calculated, and rationed. In short, we go from seeing nature as the phenomena we’re a part of… …to seeing it as natural resources for everyday business. That’s the essence of modern technology: using powerful machines to turn everything into a consumable or disposable resource. From natural to human resources Now, if that critique sounds radical, it’s worth mentioning Heidegger was no hippie. (Quite the opposite, but that’s another story.) There’s nothing necessarily wrong with using technology to “enframe” nature this way. After all, civilization requires resources to survive. However, there’s a danger when we take this line of reasoning too far. For instance, we may use technology to enframe ourselves. Says Heidegger, As soon as what is unconcealed no longer concerns man even as object, but does so, rather, exclusively as standing-reserve, and man in the midst of objectlessness is nothing but the orderer of the standing-reserve, then he comes to the very brink of a precipitous fall; that is, he comes to the point where he himself will have to be taken as standing-reserve. In other words, once we take enframing to an extreme, we may use technology to turn one another into “human resources” (which is, of course, how we refer to workers now, as opposed to the more dignified term “personnel”). At this point, we may feel like disengaged, powerless cogs in a machine. Since that feeling of disengagement is not uncommon in high-tech workplaces today (according to Gallup), I suspect Heidegger would have appreciated the movie Office Space. Rethinking technology What if some of us object to technology turning us into human resources? Fortunately, there’s an alternative. Instead of seeing technology as the means to turn everything (including ourselves) into resources, we can see technology as art. Technology as art Ultimately, Heidegger wanted to revive an earlier understanding of technology. According to Heidegger, understanding technology as enframing—turning everything into a consumable or disposable resource—ignores a more holistic understanding of technology. As he points out, technology, etymologically speaking, means artistic skill or craftsmanship (from the ancient Greek word techne, from which we also get the words technique and technics): techne is the name not only for the activities and skills of the craftsman, but also for the arts of the mind and the fine arts. Technology as a relationship Therefore, Heidegger suggests, if we see technology as art, we come across a valuable insight. As an art, technology is more than a thing. It’s a relationship as well—an aesthetic and ethical way of relating to nature and society. If that insight is right, then as we use technology to extract resources, we should also make sure we’re not inflicting damage in the process—for instance, on the environment (dumping pollution) or upon each other (treating workers like cogs in machines). As a philosopher, what Heidegger was implying is that, at the end of the day, we can’t separate technology from human values. And really, that’s what the philosophy of technology is all about. HAPPINESS AND GOOD LIFE Key Terms: Virtue: A character trait or quality deemed to be morally good and valued as a foundation for good moral being. Morality: The principles and rules that determine what is right and wrong behavior. Ethics: The branch of philosophy that deals with questions about what is morally right or wrong, good or bad. Ancient Greek vs Modern Perspectives Ancient Greek: - Aristotle: Eudaimonia as the highest good, achieved through virtue and rational activity. - Socrates: The moral life as essential to happiness; "Know thyself" emphasizes self-awareness. Modern Perspective: - Emphasis on personal fulfillment, self-expression, and individual rights. - Success linked to material achievement and personal happiness. - Influence of technology on values and well-being. - Challenge of establishing objective truths in a diverse society. Aristotle’s Human Flourishing The realization of human potential and well-being, encompassing emotional, social, and intellectual growth. Key Components Emotional Growth: Developing emotional intelligence and resilience. Social Growth: Building meaningful relationships and community connections. Intellectual Growth: Engaging in lifelong learning and critical thinking. Aristotle's Perspective Eudaimonia: Flourishing as eudaimonia, often translated as happiness but more accurately described as "living well" or "fulfilling one's potential. Virtue and the Mean: Flourishing is achieved through the practice of virtues, which lie between excess and deficiency. A virtuous life leads to a balanced and fulfilling existence. Role of Community: Human flourishing is not solely an individual endeavor; it is deeply connected to one’s community and relationships. Key Concepts: Phronesis and Sophia Phronesis (Practical Wisdom):The ability to make sound decisions based on experience and ethical considerations. It involves applying moral virtues to real-life situations and navigating complexities in daily life. Sophia (Theoretical Wisdom):Refers to the pursuit of knowledge and understanding of fundamental truths about the world. It involves deep contemplation and intellectual insight, contributing to a well-rounded understanding of life. Connection to S&T Facilitating Growth: Advancements in S&T provide tools and resources that enhance quality of life and enable individuals to pursue their goals. Promoting Well-being: Innovations in healthcare, education, and communication contribute to emotional, social, and intellectual flourishing, allowing individuals to thrive in various aspects of life. Western vs. Eastern Philosophy on the Good life Western Philosophy: Focus: Individualism and self-actualization. Key Thinkers: Aristotle: Emphasis on virtue and the pursuit of happiness through rational activity. C.S. Lewis: Highlights the importance of moral values, love, and community. Concepts: Eudaimonia: Achieving a fulfilling and meaningful life through virtue and rationality. Scientism: The belief that science is the ultimate path to knowledge and understanding. Eastern Philosophy Collectivism and harmony with the universe. Key Thinkers: Confucius: Advocates for moral integrity, social harmony, and the importance of relationships. Buddha: Emphasizes the cessation of suffering through understanding and mindfulness. Concepts: Dharma: The moral law and duty one must follow, leading to a harmonious life. Zen: Encourages mindfulness and being present in the moment as a path to enlightenment. Key Differences Approach to Life: Western philosophy often promotes the pursuit of personal goals and aspirations, while Eastern philosophy emphasizes community, balance, and interconnectedness. Understanding of Happiness: Western thought frequently associates happiness with achievement and success, whereas Eastern philosophies view happiness as a state of being that arises from harmony and fulfillment of one's duties. Other Philosophical Perspectives Socrates & Plato: The moral life is centered on virtue, emphasizing the importance of ethical conduct and inner harmony over external pleasures. - Virtue as the core of a good life. - The importance of self-examination and moral integrity. Epicurus: The Life of Pleasure: Hedonism focuses on maximizing pleasure and minimizing pain. - Misrepresentation of Epicureanism: a focus on higher pleasures (intellectual and emotional) rather than mere sensory indulgence. - Balance between pleasure and virtue for a fulfilling life. C.S. Lewis Primary Texts: - Scientism: Its Impact on Modern Thought - The Abolition of Man Main Ideas: - Critiques scientism, arguing it limits understanding of morality and meaning. - Advocates for moral absolutes, asserting that a meaningful life is grounded in objective values. - Warns against the dangers of neglecting moral considerations in favor of purely scientific reasoning. Scientism Definition: The belief that science is the ultimate authority on knowledge and that it can explain all aspects of life. Critique: While science provides valuable insights, overreliance on scientism can lead to neglecting moral, philosophical, and spiritual dimensions of existence. Martin Heidegger Primary Text: Being and Time Main Ideas: - Focus on the nature of Being and the significance of existence. - Emphasizes the importance of authenticity in life. - Advocates for confronting mortality to live meaningfully. Emrys Westacott Primary Text: What is a Good Life Main Ideas: - Provides an overview of the good life through various philosophical lenses. Discusses: - Moral life (Socrates and Plato) - Pleasure (Epicurus) - Fulfillment (Aristotle) - Highlights the balance between subjective happiness and objective conditions for living well. The Finished Life Call No Man Happy Until He’s Dead: This suggests that the true assessment of a life’s quality can only be made in retrospect. Key Ideas: The long-term view of a life well-lived emphasizes the implications of moral and ethical actions over time. Legacy: How one’s actions contribute to the flourishing of others and society. Interconnections Between Philosophy, Science, and magic How do philosophical ideas influence scientific inquiry and technological advancements? Definition of Magic: A metaphor for unexplained experiences and phenomena in life, often reflecting our yearning for understanding and connection. The role of science in fostering human flourishing, bridging gaps in knowledge and enhancing our existential experiences. Comparing Science and Magic Unexplained Phenomena: Both seek to explain phenomena that may initially seem mysterious, though through different approaches. Transformation of Understanding: Scientific discoveries often transform what was once considered magical into understandable concepts. Human Fascination: Both science and magic captivate the human imagination, representing our desire to explore the unknown. The good life synthesizes moral virtue, pleasure, engagement, and existential meaning. Insights from various philosophers enrich our understanding of a fulfilling life.