Elements of Mechanical Engineering (Internal Combustion Engines) PDF
Document Details
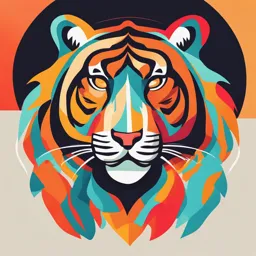
Uploaded by DeliciousConnemara5174
N.M.A.M. Institute of Technology, Nitte
Tags
Summary
These notes cover the fundamental concepts of internal combustion engines, including their classification, components, and basic terminology. The document details different types of IC engines, classification of engines, and parts of the engine.
Full Transcript
Elements of Mechanical Engineering INTERNAL COMBUSTION ENGINES Introduction A heat engine is a prime mover which derives the heat energy from the combustion of fuels any other source and converts this energy into mechanical work. In the heat engines, the mechanical work p...
Elements of Mechanical Engineering INTERNAL COMBUSTION ENGINES Introduction A heat engine is a prime mover which derives the heat energy from the combustion of fuels any other source and converts this energy into mechanical work. In the heat engines, the mechanical work produced is a linear work which in turn is converted into rotational work by the elements such as cylinder, piston, connecting rod, crank, etc. The heat engines are mainly classified into: (1) External combustion engines and (2) Internal combustion engines. In the external combustion engines known as E.C. Engines, the combustion the fuel takes place outside the engine cylinder, ex: steam engine. In the internal combustion engines known as I. C. Engines, the combustion of the fuel takes place inside the engine cylinder, ex: petrol engines, diesel engines. I.C. Engines: An internal combustion engine more popularly known as I.C. Engine, is a heat engine which converts the heat energy released by the combustion of the fuel taking place inside the engine cylinder into mechanical work. Its versatile advantages such as high efficiency, light weight, compactness, easy starting, adaptability, suitability for mobile applications, comparatively lower initial cost has made its use as an universal prime mover. Classification of IC engines I.C. Engines are classified according to: (i) Nature of Thermodynamic Cycle as.. 1. Otto cycle engine. 2. Diesel cycle engine. 3. Dual combustion cycle engine. (ii) Type of the Fuel- used as.. 1. Petrol engine. 2. Diesel engine. 3. Gas engine. 4. Bi-fuel Engine. 5. Dual Fuel Engine (iii) Number of Strokes as.. 1. Four stroke engine. 2. Two stroke engine. (iv) Method of Ignition as.. 1. Spark ignition engine (SI) engine 2. Compression ignition engine (CI) engine NMAMIT, Nitte Elements of Mechanical Engineering (v) Number of Cylinders as.. 1. Single cylinder engine. 2. Multi-cylinder engine. (vi) Position of the Cylinder as.. 1. Horizontal engine 2. Vertical engine. 3. Vee engine. 4. Opposed cylinder engine. 5. Radial engine. (vii) Method of Cooling as.. 1. Air cooled engine. 2. Water cooled engine. (viii) Speed of the Engine as.. 1. Low speed engine. 2. Medium speed engine. 3. High speed engine. NMAMIT, Nitte Elements of Mechanical Engineering Parts of Internal Combustion engine Parts of I. C. Engine a) Cylinder: The heart of the engine is the cylinder in which the fuel is burnt and fuel power is developed. The inside diameter is called bore. To prevent the wearing of the cylinder block, a sleeve will be fitted tightly in the cylinder. The piston reciprocates inside the cylinder. b) Piston: The piston is a close fitting hollow-cylindrical plunger moving to and-fro in the cylinder. The power developed by the combustion of the fuel is transmitted by the piston to the crank shaft through the connecting rod. c) Piston Rings: The piston rings are the metallic rings inserted into the circumferential grooves provided at the top end of the piston. These rings maintain a gas-tight joint between the piston and the cylinder while the piston is reciprocating in the cylinder. They also help in conducting the heat from the piston to the cylinder. d) Connecting Rod: It is a link that connects the piston and the crankshaft by means of pin joints. It converts the rectilinear motion of the piston into rotary motion of the crankshaft. e) Crank and Crankshaft: The crank is a lever that is connected to the end of the connecting rod by a NMAMIT, Nitte Elements of Mechanical Engineering pin joint with its other end connected rigidly to a shaft, called crankshaft. It rotates about the axis of the crankshaft and causes the connecting rod to oscillate. Crankshaft is s a shaft that transmits power from engine to wheels. f) Valves: The valves are the devices which controls the flow of the intake and the exhaust gases to and from the engine cylinder. They are also called poppet valves. These valves are operated by means of cams driven by the crankshaft through a timing gear or chain. g) Flywheel: It is a heavy wheel mounted on the crankshaft of the engine to maintain uniform rotation of the crankshaft. It stores the energy during power stroke and delivers the stored energy during the remaining strokes of the engine. h) Crankcase: It is the lower part of the engine serving as an enclosure for the crankshaft and also as a sump for lubricating oil. Basic IC engine terminology CLEARANCE VOLUME TDC EXTREME TOP POSITION OF PISTON STROKE LENGTH BORE BDC EXTREME BOTTOM POSITION OF PISTON Basic IC engine terminology a) Bore: The inside diameter of the cylinder is called the bore diameter. b) Stroke: the linear distance along the cylinder axis between the two limiting positions of the piston is called stroke or stroke length. a) Top Dead Centre (T.D.C): The top most position of the piston towards cover end side of the cylinder is called top dead centre. In case of horizontal engine, it is called as inner dead centre (I.D.C). b) Bottom Dead Centre (B.D.C): The lowest position of the piston towards the crank end side of the cylinder is called bottom dead centre. In case of horizontal engine, it is called outer dead centre (O.D.C). c) Clearance Volume: The volume contained in the cylinder above the top of the piston, when the piston is at the top dead centre is called clearance volume. d) Compression Ratio: It is the ratio of total cylinder volume to the clearance volume. NMAMIT, Nitte Elements of Mechanical Engineering Four-Stroke Petrol Engine (SI engine) The four-stroke cycle petrol engines work on the principle of theoretical Otto (constant volume) cycle as shown in fig 3.2. Since ignition in these engines is due to a spark, they are also called spark ignition engines. The piston performs four strokes to complete one working cycle. The four different strokes are: i) Suction stroke, ii) Compression stroke iii) Working or power or expansion stroke, and iv) Exhaust stroke.. NMAMIT, Nitte Elements of Mechanical Engineering Four stroke petrol engine Suction Stroke: During suction stroke, the inlet valve is open and exhaust is closed. The piston moves from the top dead centre to the bottom deadcentre and during this stroke the crankshaft revolves by half rotation. The energy required to perform this stroke is supplied by cranking only during the first cycle at the time of stating. At the beginning of this stroke, since the inlet is open, the pressure in the cylinder will be atmospheric. As the piston gradually moves from TDC to BDC the volume in the cylinder increases and pressure decreases. This sets up a pressure difference between the atmosphere and inside of cylinder due to which the proportionate air-petrol mixture is drawn into the cylinder through the carburetor thereby causing downward movement of the piston. This operation is represented by the line AB on the P-V diagram. At the end of this stroke, the cylinder will be completely filled with petrol air mixture and inlet is closed by inlet valve. Compression Stroke: During compression stroke, both the inlet and outlet valves are closed. The piston moves from bottom dead centre to the top dead centre and crankshaft revolves by half rotation. During this stroke the petrol and air mixture contained in the cylinder will be compressed and ratio of compression ranges from 1:7 to 1:11. Due to compression, the pressure and temperature areincreased and is shown by the line BC on the P- V diagram. Just before the end of this stroke the spark plug initiates a spark which ignites the mixture and combustion takes place at constant volume as shown by the line CD. Working Stroke: During this stroke both inlet and exhaust are closed. The piston moves from TDC to BDC and crankshaft revolves by half rotation. The high pressure burnt gases force the piston to perform this stroke called expansion or working or power stroke. The expansion of gases due to the heat of combustion exerts a pressure on the piston and as the piston moves the pressure of hot gases gradually decreases. The expansion of the burnt gases is shown by the curve DE. At or near the end of this stroke, the exhaust valve opens which will release burnt gases to the atmosphere that brings the pressure in the cylinder to atmospheric. This drop in pressure at constant volume is represented by vertical line EB. Exhaust Stroke: During this stroke, the inlet valve remains closed and the exhaust valve opens. The piston moves from bottom dead centre to top dead centre and crankshaft revolves by half rotation. The energy required to perform this stroke is supplied by flywheel. As the piston performs this stroke, the burnt gases will be released out of the cylinder at atmospheric pressure represented by horizontal line NMAMIT, Nitte Elements of Mechanical Engineering BA Four Stroke Cycle Diesel Engine (CI engine) The four-stroke diesel engines work on the principle of theoretical diesel cycle or constant pressure heat addition cycle as shown in fig Theoretical Diesel cycle Since ignition in these engines is due to the high temperature of the compressed air with compression ratio, they are also calledcompression ignition engines. The construction and working of the four stroke diesel engine is as shown. Four stroke diesel engine NMAMIT, Nitte Elements of Mechanical Engineering Suction Stroke: During suction stroke, the inlet valve is open and exhaust is closed. The piston moves from the top dead centre to the bottom deadcentre and during this stroke the crankshaft revolves by half rotation. The energy required to perform this stroke is supplied by cranking only during the first cycle at the time of stating. At the beginning of this stroke, since the inlet is open, the pressure in the cylinder will be atmospheric. As the piston gradually moves from TDC to BDC the volume in the cylinder increases and pressure decreases. This sets up a pressure difference between the atmosphere and inside of cylinder due to which only the atmospheric air is drawn into the cylinder through the air filter and inlet thereby causing downward movement of the piston. This operation is represented by the line AB on the P-V diagram. At the end of this stroke, the cylinder will be completely filled with air and inlet is closed by inlet valve. Compression Stroke: During compression stroke, both the inlet and outlet valves are closed. The piston moves from bottom dead centre to the top dead centre and crankshaft revolves by half rotation. During this stroke the air in the cylinder will be compressed and ratio of compression ranges from 1:20 to 1:22. Due to compression, the pressure and temperature areincreased and is shown by the line BC on the P- V diagram. The air as it is being compressed gets heated up and therefore its temperature also increases. At or near the end of this stroke a metered quantity of diesel oil is sprayed into the cylinder through the injector. The high temperature of the air ignites the diesel oil as soon as it is sprayed. Working Stroke: During this stroke both inlet and exhaust are closed. The piston moves from TDC to BDC and crankshaft revolves by half rotation. The auto ignition of diesel oil initiates combustion as a result hot gases are released. The burnt gases released by combustion of diesel oil exerts a pressure on the piston and forces it to perform earlier part of this stroke at constant pressure till injection of diesel oil gets completed. This constant pressure expansion with increase in the volume due to injection of diesel oil to the compressed air is represented by horizontal line CD. As the piston moves, the pressure of hot gases gradually decreases and this expansion of the burnt gases is shown by the curve DE. At or near the end of this stroke, the exhaust valve opens which will release burnt gases to the atmosphere that brings the pressure in the cylinder to atmospheric. This drop in pressure at constant volume is represented by vertical line EB. Exhaust Stroke: During this stroke, the inlet valve remains closed and the exhaust valve opens. The piston moves from bottom dead centre to top dead centre and crankshaft revolves by half rotation. The energy required to perform this stroke is supplied by flywheel. As the piston performs this stroke, the burnt gases will be released out of the cylinder at atmospheric pressure represented by horizontal line BA. Comparison between petrol engine and diesel engine Sl. No Principal Petrol Engine Diesel Engine 1. Theoretical Cycle Otto Cycle Diesel Cycle NMAMIT, Nitte Elements of Mechanical Engineering 2. Fuel used Petrol Diesel 3. Admission of fuel In first stroke the petrol enter Pressurized oil is the cylinder via carburetor injected 4. Charge drawn during Air + Petrol Only Air is drawn suction 5. Compression Ratio Low High 6. Ignition of fuel Spark plug Compression ignition or auto ignition 7. Engine speed High about 3000 rpm Low b/w 500 to 1500rpm 8. Power output Low High 9. Thermal efficiency Low High 10. Noise & Vibration Less High 11. Weight Less More 12 Initial Cost Same power input initial coat Initial cost is high is less 13 Operating Cost High operating Cost because Low operating cost as petrol is costly diesel is cheaper 14 Maintenance cost Less higher 15 Starting of engine Easily started in cold Difficult to start in cold condition weather 16 Exhaust gas pollution More Less 17 Uses Scooter, motor cycle, cars etc Trucks, Tractors, Bull dozers etc NMAMIT, Nitte Elements of Mechanical Engineering Indicated power is the power produced inside the IC engine cylinder that is transmitted through the piston, connecting rod andcrank. Therefore a certain fraction of the indicated power produced inside the cylinder will be lost dueto friction of the moving parts of the engine. Therefore net power available at the crankshaft will be equal to the difference between the Indicated power produced inside the engine cylinder and the powerlost due to friction. The net power available at the crankshaft is measured by applying the brake and is therefore called brake power. The amount of the power lost in friction is called friction power. The friction power is the difference between the indicated power and the brake power. Friction Power = Indicated power - Brake Power Brake power is calculated as follows: W = Net load acting on the brake drum, kg R = Radius of the brake drum, m N = Revolutions per Minute of the crankshaft T = Torque applied due to the net load W on the brake drum, N-m T = W*R kg-m T = 9.81*W*R N-m 2NT Brake Power = kW 60000 Mechanical Efficiency: It is the efficiency of the moving parts of the mechanism transmitting the indicated power to the crank shaft. Therefore it is defined as the ratio of the brake power and the indicated power. It is expressed in percentage. Mechanical Efficiency = ηmech = BP / IP * 100 Thermal Efficiency: It is the efficiency of conversion of the heat energy produced by the actual combustion of the fuel into the power output of the engine. Therefore it is defined as the ratio of the power developed by the engine to the heat supplied by the fuel in the same interval of time. It is expressed in percentage. Power Output Thermal Efficiency *100 Heat Energy Supplied by the Fuel The power output to be used in the above equation may be brake power or indicated power accordingly the thermal efficiency is called brake thermal efficiency or indicated thermal efficiency. The brake thermal efficiency is defined as the ratio of brake power to the heat supplied by the fuel. It is expressed in percentage. Brake Power BrakeThermal Efficiency *100 Heat Energy Supplied by the Fuel BP m f *CV bth NMAMIT, Nitte Elements of Mechanical Engineering *100 where mf = mass of the fuel supplied kg/s CV = Calorific Value of the fuel kJ/kg The indicated thermal efficiency is defined as the ratio of indicated power to the heat supplied by the fuel. It is expressed in percentage. Indicated Power Indicated Thermal Efficiency *100 Heat Energy Supplied by the Fuel NMAMIT, Nitte Unit II Insight into future mobility technology Electric Vehicles Electric Vehicles first came into existence in the mid-19th century, when electricity was among the preferred methods for motor vehicle propulsion, providing a level of comfort and ease of operation that could not be achieved by the gasoline cars of the time. In the 21st century, Electric Vehicles saw a resurgence due to technological developments and an increased focus on renewable energy. Electric vehicles (EVs) are a revolutionary mode of transportation that utilize electricity as their primary source of power instead of traditional internal combustion engines fueled by gasoline or diesel. EVs have gained significant popularity in recent years due to their potential to reduce greenhouse gas emissions, improve energy efficiency, and decrease our dependence on fossil fuels. EVs include road and rail vehicles, surface and underwater vessels, electric aircraft and electric spacecraft. Types of Electric Vehicles Battery Electric Vehicles (BEVs): BEVs are fully electric vehicles that rely solely on a rechargeable battery pack to store electricity. They produce zero tailpipe emissions and are charged by plugging into an electric power source, such as a home charger or a public charging station. Notable examples include the Tesla Model 3 and Nissan Leaf. Plug-in Hybrid Electric Vehicles (PHEVs): PHEVs combine an internal combustion engine with an electric motor and a battery. They can run on electricity for a certain distance before switching to gasoline or another fuel source. PHEVs offer flexibility and reduced emissions, with examples like the Chevrolet Volt and Toyota Prius Prime. Hybrid Electric Vehicles (HEVs): HEVs have both an internal combustion engine and an electric motor but cannot be charged externally. They primarily use regenerative 77 braking to charge their small battery, improving fuel efficiency. The Toyota Prius is a well-known HEV. Components of Electric Vehicles Battery Pack: The battery pack stores electrical energy and is the primary power source for EVs. It typically consists of numerous lithium-ion battery cells connected in series and parallel to provide the required voltage and capacity Electric Motor: The electric motor is the primary source of propulsion in electric vehicles. It converts electrical energy from the battery into mechanical energy to drive the wheels. There can be one or multiple electric motors, depending on the vehicle's design. Power Electronics: Power electronics components, such as inverters and converters, are responsible for controlling the flow of electricity between the battery, electric motor(s), and other vehicle systems. They convert DC power from the battery to AC power for the motor and vice versa Transmission: Electric vehicles often use single-speed transmissions or direct-drive systems, as electric motors provide a wide range of torque and power without the need for multiple gears. Some electric vehicles have a multi-speed transmission for efficiency or performance reasons. Charging System: EVs come equipped with a charging port and system to connect to external power sources. This system includes onboard chargers that convert AC power from charging stations to DC power for the battery. Charging ports vary in terms of compatibility and charging speed (Level 1, Level 2, and DC fast charging). Regenerative Braking System: Both electric and hybrid vehicles utilize regenerative braking systems to capture and convert kinetic energy during braking or coasting into electrical energy. This energy is then used to recharge the battery, improving energy efficiency. Thermal Management System: Maintaining an optimal temperature range for the battery and electric motor is crucial for their performance and longevity. Electric and hybrid vehicles employ cooling and heating systems to manage thermal conditions. 78 Advantages of Electric Vehicles Zero Emissions: EVs produce zero tailpipe emissions, reducing air pollution and greenhouse gas emissions. They are a cleaner and more environmentally friendly option, especially when powered by renewable energy sources. Energy Efficiency: Electric motors are highly efficient at converting electrical energy into motion. This efficiency results in lower energy consumption and reduced operating costs compared to ICEVs. Lower Operating Costs: EVs have fewer moving parts than ICEVs, which means reduced maintenance costs. There is no need for oil changes, and brake pads can last longer due to regenerative braking. Quiet Operation: Electric motors are quieter than internal combustion engines, providing a more peaceful and comfortable driving experience. Instant Torque: Electric motors deliver instant torque, resulting in quick acceleration and responsive performance. Home Charging: EVs can be conveniently charged at home using standard electrical outlets or dedicated home charging stations, reducing the need for trips to gas stations. Reduced Dependence on Fossil Fuels: EVs reduce the dependence on fossil fuels, which are finite and subject to price volatility. Incentives: Many governments offer incentives and subsidies to encourage the adoption of electric vehicles, including tax credits, rebates, and access to carpool lanes. Disdvantages of Electric Vehicles Limited Range: Although EV ranges have been improving, some models may still have limited driving ranges compared to ICEVs. Long road trips may require more planning. Charging Time: Charging an EV takes longer than refueling a gasoline vehicle. While home charging is convenient overnight, long trips might involve longer stops for charging. Upfront Cost: The initial purchase price of EVs can be higher than that of comparable ICEVs, primarily due to the cost of the battery. However, this cost difference is decreasing over time. Charging Infrastructure: In some regions, the availability of charging stations, especially in less urbanized areas, can be a concern. However, this is changing rapidly with increasing investment in charging infrastructure. Limited Model Variety: While the selection of EV models is growing, there may still be limited choices in certain vehicle categories and price ranges compared to ICEVs. Battery Degradation: Over time, the battery's capacity can degrade, reducing the vehicle's range. However, advancements in battery technology are mitigating this issue. Electricity Source: The environmental benefits of EVs depend on the source of electricity. If the electricity is generated from coal or other non-renewable sources, the carbon footprint may not be significantly reduced 79 Hybrid Vehicles A hybrid vehicle, often referred to simply as a "hybrid," is a type of vehicle that combines two or more distinct power sources to provide propulsion. The most common type of hybrid vehicle combines an internal combustion engine (usually gasoline or diesel) with an electric motor and a battery. Possible combinations include diesel/electric, gasoline/fly wheel, and fuel cell (FC)/battery. Typically, one energy source is storage, and the other is conversion of a fuel to energy. The key feature of hybrid vehicles is their ability to switch between these power sources to improve fuel efficiency, reduce emissions, and optimize performance. Components of Hybrid Vehicles Internal Combustion Engine (ICE): Hybrid vehicles have a traditional internal combustion engine, often smaller than that of a non-hybrid vehicle. The ICE provides primary power when needed, typically during high-speed driving or heavy acceleration. Electric Motor(s): Hybrid vehicles have an electric motor that assists the internal combustion engine. This motor can operate independently or work in conjunction with the engine to provide additional power and improve fuel efficiency. Battery Pack: Hybrid vehicles contain a smaller battery pack than pure electric vehicles. This battery stores electrical energy and is used to power the electric motor. It is charged through regenerative braking and excess engine power. Power Electronics: Power electronics components, such as inverters and converters, control the flow of electricity between the battery, electric motor, and engine. They convert DC power from the battery to AC power for the motor and vice versa. Transmission: Most hybrid vehicles use a specialized transmission, such as a continuously variable transmission (CVT) or an automatic transmission with integrated electric drive components. These transmissions manage the power from both the engine and electric motor. Regenerative Braking System: Hybrid vehicles use regenerative braking to capture and convert kinetic energy during braking or coasting into electrical energy, which is then used to recharge the battery. This system improves energy efficiency. 80 Engine Control Unit (ECU): The ECU coordinates the operation of the internal combustion engine and electric motor. It determines when to use each power source for optimal efficiency and performance. Advantages of Hybrid Vehicles Improved Fuel Efficiency: Hybrids are designed to optimize fuel efficiency by combining an internal combustion engine with an electric motor. This combination allows them to achieve higher miles per gallon (MPG) ratings than comparable non- hybrid vehicles. Reduced Emissions: Hybrids produce fewer tailpipe emissions than conventional gasoline or diesel vehicles. Regenerative Braking: Hybrids use regenerative braking systems to capture and convert kinetic energy during braking into electrical energy, which is then used to recharge the vehicle's battery. This technology not only improves fuel efficiency but also reduces wear and tear on the brake pads. Quiet Operation: Electric motors in hybrid vehicles operate quietly, providing a more peaceful and comfortable driving experience, especially in city traffic. Reduced Fuel Costs: While hybrids may have a higher upfront cost compared to traditional vehicles, their improved fuel efficiency can lead to significant fuel cost savings over the life of the vehicle, particularly for drivers with long commutes or frequent city driving. Extended Driving Range: Hybrids combine the advantages of gasoline engines with electric motors, providing an extended driving range. Incentives and Tax Benefits: Many governments offer incentives and tax benefits to encourage the adoption of hybrid vehicles. These incentives can include tax credits, rebates, and access to carpool lanes. Lower Maintenance Costs: Hybrids often require less maintenance than traditional vehicles because they use regenerative braking and have fewer moving parts in the powertrain. Resale Value: Hybrid vehicles tend to retain their value well in the used car market due to their fuel efficiency and environmentally friendly image. Technological Advancements: Automakers continue to invest in hybrid technology, leading to advancements in hybrid systems, battery technology, and overall vehicle performance. Environmental Benefits: By reducing fuel consumption and emissions, hybrids contribute to reducing air pollution and greenhouse gas emissions, helping combat climate change and improve air quality. Disadvantages of Hybrid Vehicles Higher Initial Cost: Hybrid vehicles typically have a higher upfront purchase price compared to their non-hybrid counterparts. Limited Electric-Only Range: Most hybrid vehicles, especially non-plug-in hybrids (HEVs), have limited electric-only driving ranges. This means they still rely on the 81 internal combustion engine for longer trips or highway driving, limiting the potential for all-electric operation. Battery Degradation: Over time, the hybrid battery's capacity can degrade, resulting in reduced electric-only range and overall performance. Charging Infrastructure for Plug-In Hybrids: Plug-in hybrid electric vehicles (PHEVs) require access to charging infrastructure for their electric mode. Maintenance Complexity: Hybrids have both an internal combustion engine and an electric motor, which can make maintenance and repairs more complex and potentially more expensive. Unit II Refrigeration & Air conditioning Refrigeration is defined as a method of reducing the temperature of a system below that of the surroundings and maintaining it at the lower temperature by continuously abstracting heat from it. In a refrigerator, the working fluid that continuously extracts heat from within the refrigerator which is required to be cooled is called a refrigerant. Refrigeration effect and Unit of refrigeration In a refrigeration system, the rate at which the heat is absorbed in a cycle from the interior space to be cooled is called a refrigerating effect. The unit of refrigeration is ton. It is also the capacity of refrigeration effect produced. A ton of refrigeration is defined as the quantity of heat absorbed in order to form one ton of ice in 24 hours when the initial temperature of the water is 0oC. One American ton (2000 pounds) is taken as the standard in refrigeration practice. But in S.I. system, 1 ton of refrigeration = 3.5 kW Coefficient of performance The performance of a refrigeration system is expressed by a factor known as the coeficient of peformance (COP). The COP of a refrigeration system is defined as the ratio of heat absorbed in a system to the work supplied. If Q = Heat absorbed or removed, kW & W = Work supplied to the motor, kW COP = Q/W Properties of a good refrigerant Boiling point: An ideal refrigerant must have low boiling temperature at atmospheric pressure. 82 Freezing point: It must have a very low freezing point because the refrigerant should not freeze at low evaporator temperatures. Thermal conductivity: It must have high thermal conductivity so that heat can be transferred to and from the refrigerant very easily. Specific heat: A good refrigerant must have low specific heat when it is in liquid state and high specific heat when it is vapourised. The low specific heat of the refrigerant helps in more heat absorption in the evaporator and high specific heat of the vapour helps in easy condensing. Both these desirable properties will increase the refrigerating effect. Specific volume: The specific volume of the refrigerant must be very low so that it occupies less volume upon vaporization. Viscosity: The viscosity of a refrigerant at both the liquid and vapour states must be very low as it improves the heat transfer and reduces the pumping effort required. Toxicity: A good refrigerant should be non-toxic. Any leakage of the toxic refrigerant increases suffocation and poisons the atmosphere or any food items stored. Corrosiveness: A good refrigerant should be non-corrosive to prevent the corrosion of the metallic parts of the refrigerators. Chemical stability: An ideal refrigerant must not decompose under operating conditions. Coefficient of Performance (COP): The COP of a refrigerant must be high so that the energy spent in refrigeration will be less. Odour: A good refrigerant must be odourless, otherwise food kept inside may lose their taste. Leakage: The refrigerant must be such that any leakage can be detected by simple tests. Commonly used refrigerants are: 1. Ammonia – It is used in ice plants and cold storage. Its melting point is and has low specific volume. It produces high refrigeration efects even in small refrigerators. It doesn’t harm the ozone. But it is toxic, flammable, irritating and destroys the food due to which it cannot be used for domestic refrigeration. 2. Carbon dioxide – It is used in marine refrigerators. The effeciency of CO2 is less, hence it is rarely used in domestic refrigerator. It is colourless, odourless, non toxic, non flammable and non corrosive. 3. Sulphur dioxide – Eariler sulphur dioxide was one of the most commonly used refrigerants in the refrigerators. But it was not used because its refrigeration effect was low and it had high specific volume due to which large capacity and high speed compressors were required. And also since it combines with water to form sulfuric acid which is corrosive. 4. Methyl chloride – Used in domestic and industrial refrigerators. Since it will burn under some conditions and is slightly toxic, it is not generally used. 83 5. Freon – Freon group of refrigerants is used almost universally in domestic refrigerators. These refrigerants are colourless, almost odourless, non toxic, non flammable, non explosive and non corrosive. Freon-12 and freon-22 are the two commonly used refrigerants in domestic refrigerator, water coolers, air conditioning plants, cold storage, food processing and storage etc. But it has been found that these refrigerants are major threat to ozone layer. Parts of a refrigerator To accomplish the task of producing the cooling effect, a refrigeraot must consist a eveporator, a condenser, a circulating device like pump or compressor and a expansion valve. Evaporator: This is the main part of the refrigeration system where the liquid refrigerant is evaporated by absorbing the heat from refrigeration space which has to be cooled. It consists of metal tubes which surrounds the freezing and cooling compartments. Circulating system: It consists of mechanical devices like pumps or compressors that are necessary to circulate the refrigerant to undergo the refrigeration cycle. They are generally driven by electric motors. The electrical energy input to the motor is the energy input to the refrigerator. Condenser: It is a device where heat from the refrigerant is rejected at higher temperature to another medium, usually the atmospheric air. Here the refrigerant vapours gets converted into liquid by rejecting the heat that was absorbed in the refrigeration space and in the compressor. Expansion device: This device reduces the pressure and temperature of the liquid refrigerant before it passes to the evaporator. Vapour compression refrigeration system 84 1. The system consists of evaporator, compressor, condenser and an throttle valve. 2. In this system, a liquid refrigerant alternatively undergoes a change of phase from vapour to liquid (condensation) and from liquid to vapour phase (evaporation) during the working cycle. 3. The liquid refrigerant in the evaporator absorbs the heat from the refrigeration space which is to be cooled and undergoes a change of phase form liquid to vapour. 4. This vapour at low temperature and pressure is drawn into the compressor where it is compressed to a high pressure and temperature. The compressed vapour then enters the condenser. 5. In the condenser the vapour refrigerant is cooled and condensed into liquid by giving its latent heat to the circulating cooling medium (air or water). 6. The high-pressure liquid refrigerant leaves the condenser and passes through the throttle valve where it is expanded to low pressure and temperature. The temperature of the refrigerant falls to a value less than that of the refrigerated space. 7. The low pressure-low temperature refrigerant again enters the evaporator where it absorbs the heat from the refrigeration space and evaporates. And the cycle repeats. 85 Air conditioning 1. Air conditioning is defined as providing a comfortable indoor atmosphere by simultaneous control of temperature, humidity, air filtering, air purification and recirculation of the air. 2. The air conditioning system consists of a compressor, condenser, evaporator, throttle valve, condenser and evaporator fans driven by the same motor. 3. The evaporator fan and the evaporator coils of the unit always lie inside the building or space which is to be conditioned. 4. Condenser and the condenser fan of the unit projects outside the building or space to enable heat transfer with the atmosphere. 5. The low pressure-low temperature refrigerant enters the evaporator coils. The evaporator fan continuously draws hot air from the conditioned space and circulates it over the evaporator coils. 6. The hot air passing through the air filter comes in contact with cold evaporator coils and exchanges its heat. The cool fresh air enters the conditioned space. 7. The refrigerant vapours enter the compressor and gets compressed to a higher pressure and temperature. 8. The high pressure refrigerant leaving the compressor enters the condenser coils. The latent heat of the refrigerant vapour is given to the surrounding atmosphere. 9. Condensation takes place due to this heat transfer as the condenser fan draws air from outside the building and circulates it over the condenser coils. 10. The high pressure liquid refrigerant enters the throttle valve and expands in it. The pressure of the refrigerant reduces. This refrigerant moves to the evaporator coils and the cycle repeats. 11. Desired temperature inside the room can be adjusted by thermostatic control device. 86 Elements of Mechanical Engineering 8. POWER TRANSMISSION Introduction The rotational motion can be transmitted from one mechanical element to the other with the help of certain systems known as transmission systems (Drives). Machines may be driven by any of the following two methods such as Individual Drive and Group drive. Individual Drive When an individual machine is fitted with its own electric motor then the drive is called individual drive. Each machine has its own electric motor and starter. The motor may drive the machine shaft through belt, chain, gears etc. It is used on machines that require considerable power operating at a full load. Group Drive When several machines are organized on a single shaft then the system is called group drive. A very powerful motor drives an overhead shaft (Main shaft), that runs from one end to other end of the shop. Main shaft drives countershaft, which in turn drives the machine drive shafts. It is most suitable, when power consumption of individual machines is extremely variable. The methods of power transmission are i. Belt drive. ii. Gear drive. iii. Rope drive. iv. Chain drive. NMAMIT, Nitte Elements of Mechanical Engineering Belt drives : It is one of the most common and effective devices of transmitting motion from one shaft to another by means of a thin inextensible band running over two pulleys. Uses: Mills & Factories. Belt materials: Materials of the belt are made up of leather for dry and wet conditions, rubber for damp conditions along with canvas and balata. There are 2 types of belt drives such as open belt drive and crossed belt drive Open Belt Drive: This type of belt drive is employed when the two parallel shafts have to rotate in the same direction. When the shafts are placed far apart, the lower side of the belt should be the tight side and the upper side must be the slack side. This is because, when the upper sidebecomes the slack side, it will sag due to its own weight and thus increases the arc of contact which in turn increases the capacity of the drive. Flat belt drives of the open system should always have their shaft axes either horizontal or inclined. They should never be vertical, for if so arranged the centrifugal force developed in the belt combined with the force of gravity causes the belt to stretch and tend to leave the rim of the pulleys, thereby losingcontact NMAMIT, Nitte Elements of Mechanical Engineering with their rim surfaces. Crossed Belt Drive: This type of belt drive is employed when the two parallel shafts have to rotate in the opposite direction. At the junction where the belt crosses, it rubs against itself and wears off. To avoid excess wear, the shafts must be placed at a maximum distance from each other end and must be operated at low speeds. The velocity ratio of a belt drive is defined as the ratio of the speed of the driven pulley to the speed of the driving pulley. Let d1 and d2 be the diameters of the driving and the driven pulleys respectively, and let N 1 and N2 be the speed of driving and driven pulleys in revolutions per minute. If there is no relative slip between the pulleys and the portions of the belt which are in contact with them, the speed at every point on the belt will be same. Thereforethe circumferential speeds of the driving and driven pulleys and the linear speed of the belt are equal. Linear speed Circumferential speed Circumferential speed of the belt = of the driving pulley = of driven pulley = Πd1N1 = Πd2N2 = d1N1 = d2N2 Velocity Ratio = N1 / N2 = d2 / d1 NMAMIT, Nitte Elements of Mechanical Engineering Ratio of belt tensions Initial tension in the belt drives NMAMIT, Nitte Elements of Mechanical Engineering Power transmitted in belt drives Difference between open belt and crossed belt drive Open Belt Drive Cross Belt Drive Open belt drive is employed when two parallel Cross belt drive is employed when two parallel shafts have to rotate in same direction shafts have to rotate in opposite direction In open belt drive, the belt proceeds from the top In cross belt drive, the belt proceeds from the top of of one pulley to the top of another pulley without one pulley to the bottom of another pulley and thus crossing. crosses itself. Here the entire belt remains in same plane in Here belt bends in two different planes in every every rotation. rotation. Angle of wrap or contact angle between belt and Angle of wrap is always above 180°. pulley is always below 180°. Due to smaller wrap angle, power transmission Due to larger wrap angle, power transmission capability is also lower. capability is also higher. Length of the belt in open belt arrangement is For same pulley size and centre distance, cross belt comparatively shorter. drive requires longer belt. Due to crossing between two pulleys, belt rubs Here belt does not touch itself. So no rubbing against itself and thus undergoes gradual wear. Thus takes place. Thus belt exhibits longer life. belt has shorter life. In between two pulleys, belt vibrates (whips) Cross belt arrangement is free from such vibration. when centre distance is more. NMAMIT, Nitte Elements of Mechanical Engineering Gear drives The different types of gears used are: 1. Spur Gears-For Parallel Axes shafts. 2. Helical Gears - For both Parallel and Non-parallel and Non-intersecting Axes shafts. 3. Spiral Gears - For Non-parallel and Non-intersecting Axes shafts. 4. Bevel Gears - For Intersecting Axes shafts. 5. Worm Gears - For Non-Parallel and Non-co-planar Axes shafts. 6. Rack and Pinion - For converting Rotary motion into Linear motion. 1. Spur Gears When the axes of the driving and driven shafts are parallel and co-planar and the teeth of the gear wheels are parallel to the axes, the gears are called spur gears. The contact between the mating gears will be along a line, hence spur gears can transmit higher power. Because of the instantaneous line contact when the teeth mesh, noise will be very high. They are widely used in machine tools, automobile gear boxes and in all general cases of power transmission where gear drives are preffered. NMAMIT, Nitte Elements of Mechanical Engineering 2. Helical Gears Helical gears are similar to the spur gears except that the teeth are cut in the form of the helix around the gear. Helical gears are used for transmitting power between two parallel shafts and also between non parallel, non-intersecting shafts. The contact between the mating gears will be along a curvilinear path. Helical gears are preferred to spur gears when smooth and quiet running at higher speeds are necessary. The main disadvantage of the helical gears is that it produces end thrusts on the driving and driven shafts. Generally they are used in automobile power transmission. 3. Spiral Gears Spiral gears are used to connect only two non-parallel, non-intersecting shafts. They differ from the helical gears in that there is a point contact in spiral gears whereas a curvilinear contact exists in helical gear drives. Because of the point contact the spiral gears are more suitable for transmitting less power. 4. Bevel gears When the axes of the two shafts are inclined to one another, and intersect when produced, bevel gears are used. Teeth of the bevel gears are cut on the conical surfaces. The most commonexamples of power transmission by bevel gears are those in which the axes of the two shafts are at rightangles to each other. When two bevel gears have their axes at right angles and are of equal sizes, they are called miter gears. 5. Worm and Worm Wheel Worm gears are used to transmit power between the driving and driven shafts having their axes at right angles and non-coplanar. A worm drive consists of a worm (essentially a screw) which may have one or more number of helical threads of trapezoidal shape cut on it and a worm wheel - a gear wheel with the tooth profile consisting of a small segment of a helix which engages with the worm. Worm gears are suitable for transmission of power when a high velocity ratio as high as 60 :1 is required. They are generally employed in machine tools, like lathe, milling, drilling machines etc, to get large speed reduction. Another important characteristic of the worm and worm wheel drive is that it offers self locking facility between the driven and the driving units when the direction of the drive is reversed. 6. Rack and Pinion When a rotary motion is to be converted into a linear motion, rack and pinion arrangement is used. Rack is a rectangular bar with a series of straight teeth cut on it. Theoretically rack is considered to be a spur gear of infinite diameter. Rack and pinion arrangement, find their applicationin machine tools, such as, lathe, drilling, planing machines, and on some steep rail tracks, where the teeth of the locomotive wheel mesh with a rack embedded in the ground, offering the locomotive improved traction. NMAMIT, Nitte Elements of Mechanical Engineering Advantages and Disadvantages of Gear Drives Advantages; 1. They are positive non-slip drives. 2. Most convenient for very small centre distances. 3. By using different types of gears, it will be possible to transmit the power when the axes of the shafts are not only parallel, but even when nonparallel, intersecting, non-intersecting and co-planar or non- coplanar. 4. The velocity ratio will remain constant throughout. ' 5. They can be employed conveniently for low, medium and high power transmission. 6. Any velocity ratio as high as, even upto 60 : 1 can be obtained. 7. They have very high transmission efficiency. 8. Gears can be cast in a wide range of both metallic and non-metallic materials. 9. If required gears may be cast integral with the shafts. 10. Gears are employed for wide range of applications like in watches, precision measuring instruments, machine tools, gear boxes fitted in automobiles, aero engines, etc. Disadvantages; 1. They are not suitable for shafts of very large centre distances. 2. They always require some kind of lubrication. 3. At very high speeds noise and vibrations will be more. 4. They are not economical because of the increased cost of production of precision gears. 5. Use of large number of gear wheels in gear trains increases the weight of the machine. Velocity ratio of Gear drive The velocity ratio of a gear drive is defined as the ratio of the speed of the driving gear to the speed of the driven gear. Let d1 and d2 be the pitch circle diameters of the driving and driven gear respectively. Let T1 and T2 be the number of teeth on the driving and driven gears respectively. Let N1 and N2 be their speeds in revolutions per minute. Since there is no slip between the pitch cylinders of the two gear wheels, the linear speed of the two pitch cylinders must be equal. Linearspeedof the pitch = Linearspeedof the pitch cylinderrepresenting theDrivinggear cylinderrepresenting drivengear π d1N1= π d2N2 𝑁1 𝑑2 …………………… (1) = 𝑁2 𝑑1 NMAMIT, Nitte Elements of Mechanical Engineering The circular pitch for both the meshing gears remains same. i.e. pc = d1 = d 2 T1 T2 𝑇2 i.e., 𝑑2 = ……………………..(2) 𝑑1 𝑇1 From equation (1) and (2) 𝑁1 𝑑2 𝑇2 Velocity Ratio of a Gear Drive = = = NMAMIT, Nitte Unit II Gear drives Gear trains A gear train is an arrangement of number of successively meshing gear wheels through which the power can be transmitted between the driving and driven shafts. The different types of gear trains are 1. Simple gear train 2. Compound gear train Simple gear train Gear A Gear B Gear C Gear D In a simple gear train, a series of gear wheels are mounted on different shafts between the driving and driven shafts and each shaft carries only one gear. A → Driving gear B → Intermediate gear C → Intermediate gear D → Driven gear Velocity ratio of a simple gear train Let NA = speed in RPM of gear A NB = speed in RPM of gear B NC = speed in RPM of gear C ND = speed in RPM of gear D TA = Number of teeth of gear A TB = Number of teeth of gear B TC = Number of teeth of gear C 87 TD = Number of teeth of gear D i. A drives B NB TA = NA TB ii. B drives C NC = TB NB TC iii. C drives D ND TC = NC TD Velocity ratio between the driving and driven gears is given by, ND N D. NC. NB =.... NA = NC NB NA Substituting from (i) (ii) and (iii) ND T A. TB. TC = NA = TB.. TC TD Velocity Ratio. ND = NA Compound gear train A compound gear train is one in which each shaft carries two or more gears which are keyed to it. B → Compound gear Gear A C → Compound gear Gear B i. A drives B Gear C NB TA NA = T Gear D B Since gears B and C are keyed to the same shaft both of them rotate at the same speed. 88 NB = Nc but TB Tc ii. C drives D N D TC NC TD Velocity ratio between the driving and driven gears is given by, ND ND NC = . N A NC. N A Substituting from (i) and (ii0 N D TC 𝑇 = 𝐴 N A TD 𝑇𝐵 89 Unit II Welding, Soldering and Brazing 1. Welding is a fabrication process in which two or more workpices, usually metal are joined permanently to form a single component. 2. The process is carried out by heating edges of the workpieces to a suitable temperature and then fusing together with or without the application of pressure. 3. Since a slight gap usually exists between the edges of workpieces, a filler metal is used to supply additional material to fill the gap. 4. But welding can also be carried out without the use of filler metal. 5. An ideal joint is achieved only when the contaminated surface layers on the workpiece are removed, recontamination is avoided and the two surfaces are made smooth, flat and fit each other exactly. Classification of welding process: 1. Gas welding a. Oxy-acetylene welding b. Oxy-hydrogen welding 2. Arc welding a. Manual metal arc welding / Flux shielded arc welding b. Tungsten inert gas welding (TIG) c. Metal inert gas welding (MIG) d. Submerged arc welding (SAW) e. Plasma arc welding (PAW) 3. Resistance welding a. Spot welding b. Seam welding c. Butt welding d. Projection welding 4. Solid state welding a. Explosive welding b. Ultrasonic welding 5. Thermo-chemical welding a. Atomic hydrogen welding b. Thermit welding 6. Radiant energy welding a. Laser beam welding b. Electron beam welding Applications: Welding finds its applications in ship building, automobiles, aircraft, power plants, building and bridge constructions, storage tanks, pressure vessels, machine tools and almost in all sectors where parts are fabricated as per the needs. 90 Apart from fabrication work, welding is also used in repair and maintenance work, for example joining broken parts and rebuilding worn out components. Advantages: 1. The strength of the joint obtained in welding is much stronger than the workpiece metal. 2. Metals with different chemical compositions can be welded easily. 3. Welding equipment are portable. Hence, the parts can be fabricated at the relevant location instead of transporting the entire assembly to its destination. 4. Complex shapes that are difficult to cast or machine can be easily assembled in parts by welding process. 5. Parts can be welded at reasonable cost. Limitations: 1) The process gives out harmful radiations, fumes and spatter. Hence care should be taken during welding. 2) Skilled operators are required to produce a good joint. 3) The high heat involved in the process causes changes in the structure of metal thereby lowering its properties. Arc welding Principle of arc: 1. When two conductors of an electric circuit are touched together momentarily and then separated slightly (0.5-2.5mm), assuming that there is a sufficient voltage in the circuit to maintain the flow of current, an electric arc is formed and concentrated heat is produced throughout the length of the arc at a temperature about 5000 to 6000 0 C. 2. The arc temperature and thus the arc heat can be increased or decreased by employing higher or lower arc currents. A high arc current with a smaller arc length produces a very intense heat. 3. The arc melts the electrode and the base metal. Material droplets are transferred from the electrode to the job, through the arc, and are deposited along the joint to be 91 welded. When the arc starts it should be on the same spot till the base metal starts melting and then it should be moved steadily along the joint. The two parts of the metal melt and join together to form a single component. Manual metal arc welding (MAW) / Flux shielded metal arc welding (FSMAW) 1. An electrode holder, which holds the electrode firmly, forms one pole of the electric circuit, while the workpiece to be welded forms the other pole. Either AC or DC can be used to supply the required current for welding. 2. The electrode used in this process is a metallic wire, which is made of the same material or nearly the same chemical composition as that of the workpiece metal. 3. The metallic wire is coated with a suitable flux material like calcium fluoride, rutile etc., which gives off gases as it decomposes. 4. In operation, an arc is struck by touching the tip of the electrode on the workpiece and instantly the electrode is separated by a small distance of 2-4mm such that the arc still remains between the electrode and workpiece. 5. The high heat at the tip of the arc melts the workpiece metal forming a small molten metal pool. At the same, the tip of the electrode also melts. The molten metal of the electrode is transferred into the molten metal of the workpiece in the form of globules. 6. The deposited metal fills the joint and bonds the joint to form a single piece of metal. 7. The electrode is moved along the surface to be welded to complete the joint. 8. The arc is extinguished by increasing the arc length, i.e., by widening the gap between the workpiece and the electrode. Advantages: 1. The process is simple and inexpensive. Hence, suitable for shop jobs and field work. 2. Eliminates skilled labor. 3. The process dominates other welding processes in maintenance and repair industry. 4. Used to weld ferrous and a few non ferrous metalis like aluminium, nickel, copper alloys etc. 92 Disadvantages: 1. Weld times are rather slow, since the consumable electrode must be frequently replaced. 2. Weld spatter, poor fusion, shallow penetration and cracking are a few major problems associated with the process. Applications: The process finds application is building and bridge construction, ship building, boiler and pressure vessel fabrication, joining of large pipes and penstock, and in almost all repair and maintenance work. Tungsten inert gas welding (TIG) 1. TIG equipment consists of a welding torch in a non-consumable tungsten alloy electrode held rigidly in the collet. The diameter of the electrode varies from 0.5- 6.4mm. 2. A shielding gas (argon or helium) is used to avoid atmospheric contamination of the molten weld pool. Shielding gas flow from the cylinder, through the passage in the electrode holder and impinge on the workpiece. 3. Welding current and inert gas supply are turned on. The arc is struck by touching the tip of tungsten electrode with workpiece, and instantaneously the electrode is separated from the workpiece by a small distance of 0.5-3mm such that the arc still remains between the electrode and the workpiece. 4. High intensity of the arc melts the workpiece metal forming a small molten metal pool. Filler metal in the form of a rod is added manually to the front end of the weld pool. 5. The deposited filler metal fills and bonds the joint to form a single piece of metal. 6. At the far end of the job, arc is broken by increasing the arc length. 93 7. The shielding gas is allowed to impinge on the solidified weld pool for few seconds, even after the arc is extinguished. This will avoid atmospheric contamination of the weld metal till it cools. Advantages: 1. No flux is used; hence there is no danger of flux entrapment. 2. This process can weld in all positions and produces smooth and high quality welds. 3. TIG welding process is very much suitable for high quality welding of thin materials (as thin as 0.125 mm). Disadvantages: 1. TIG is most difficult process compared to all the other welding processes. The welder must maintain short arc length, avoid contact between electrode and the workpiece and manually feed the filler metal with one hand while manipulating the torch with the other hand. 2. Tungsten if transferred to molten weld pool can contaminate the same (tungsten inclusion is hard and brittle). 3. Skilled operator is required. 4. Equipment costs are higher than that for flux shielded metal arc welding. Applications: 1. Welding sheet metal and thinner sections, welding aluminium, magnesium, copper, nickel and their alloys etc. 2. Precision welding in atomic energy, aircraft, chemical and instrument industries. 3. Rocket motor chamber fabrications in launch vehicles. Metal inert gas welding (MIG) or Gas metal arc welding (GMAW) 1. The equipment consists of a welding torch in which a bare (uncoated) consumable electrode in the form of a wire is held and guided by a guide tube. 2. The electrode material is of the same material or nearly the same chemical composition as that of the base metal. Its diameter varies from 0.7-2.4mm. 3. The electrode is fed continuously at a constant rate through feed rollers driven by an electric motor. 4. MIG makes use of shielding gas to prevent atmospheric contamination of the molten weld pool. Mixture of argon and carbon dioxide gas is commonly used. 94 5. An arc is struck by touching the tip of electrode with the workpiece, and instantaneously the electrode is separated from the workpiece by a small distance of 1.5-3mm such that the arc still remains between the electrode and the workpiece. 6. The high intensity of the arc melts the workpiece metal forming a small molten pool. At the same time, the tip of the electrode also melts and combines with the molten metal of the workpieces thereby filling the gap between the two workpieces. 7. The deposited metal upon solidification bonds the joint to form a single piece of metal. Advantages 1. Because of continuously fed electrode, MIG welding process is much faster as compared to TIG welding. 2. Thin and thick metals can be welded. 3. The process can be easily mechanized. 4. No flux is used, hence no slag formation. This results in clean welds. Disadvantages 1. The process is slightly more complex as compared to TIG welding process. 2. Welding equipment is more complex, more costly. 95 3. Weld metal cooling rates are higher than with the process that deposit slag over the weld metal. Applications 1. The process can be used for welding of carbon, silicon and low alloy steels, aluminium, magnesium, copper, nickel and their alloys etc. 2. For welding tool steel and dies. 3. For the manufacturing of refrigerator parts. 4. MIG welding has been used successfully in industries like aircraft, automobiles, pressure vessel and ship building. Gas welding In this process, the melting of the base metal is achieved by means of gas flame which derives its intense heat from the combustion of a fuel with oxygen. The most commonly used fuel is acetylene and hydrogen are sometimes used. Filler metal may or may not be used. Principle of Oxy-acetylene welding When acetylene is mixed with oxygen in correct proportion in the welding torch and ignited, the flame resulting at the tip of the torch is sufficiently hot to melt and join the parent metal. The oxy-acetylene flame reaches a temperature of about 32000C and this temperature is high enough to melt the job to be welded (all types of commercial metals). When the base metal starts melting molten metal from the workpiece (both sides of the two work pieces) flow together to form a complete bond. 96 A filler metal rod is generally added to the molten metal pool to build up the seam slightly far greater strength. Chemistry of oxy-acetylene flame (reaction in gas welding) The oxy-acetylene process depends on the chemical reaction which occurs in two stages. Stage 1: C2H2+O2=2CO +H2+448 KJ/mol 2C2H2+2O2=4CO+2H2 (18.75 MJ/m3 of acetylene) The primary combustion provides the actual flame for welding, with temperature up to 30920 C, which is in the inner core. Stage 2: 2CO+H2+1/2 O2=2CO2+H2O OR 4CO+2H2+3O2=4CO2+2H2O+812 KJ/mol (35.77 MJ/m3) This secondary combustion occurs at the outer portion of the flame. It protects the molten puddle from attack by air and helps to pre heat the base metal. Flame characteristics For the complete combustion of the acetylene, 2.5 volumes of oxygen are required for 1 volume of acetylene. By changing the supply of oxygen and acetylene (volumes), three different flames are obtained. 1. Neutral flame. 2. Carburizing flame. 3. Oxidizing flame. Neutral Flame A neutral flame (fig a) is obtained by supplying equal volumes of oxygen and acetylene. The neutral flame consists of an inner small whitish cone surrounded by sharply defined blue flame. Most of the oxy-acetylene welding is done with the use of neutral flame. The temperature of the neutral flame may be around 32600C (59000F). Oxidizing Flame 97 The oxidizing flame (fig b) is obtained when there is excess oxygen, having gas ratio as high as 1.15 to 1.50. In appearance, it resembles a neutral flame with the exception that the inner white cone flame is somewhat shorter. This is used for oxy-acetylene cutting and is not suitable for welding; since the weld metal will be oxidized (flame temperature of oxidizing flame may be around 34800C). Carburizing Flame A carburizing flame (fig c) (reducing flame) is obtained by supplying excess acetylene in the gas ratio 0.95 to 1. It has three cones; an inner white cone surrounded by an intermediate whitish cone known as “Intermediate flame feather” and a bluish envelope flame. The flame is generally used due to its reducing nature, for welding alloy steels, caste iron and aluminum to protect from the oxidizable elements (Flame temperature may be around 3038 0C). Advantages: 1. The strength of the joint obtained in welding is much stronger than the workpiece metal. 2. Metals with different chemical compositions can be welded easily. 3. Welding equipments are portable. Hence, the parts can be fabricated at the relevant location instead of transporting the entire assembly to its destination. 4. Complex shapes can be easily assembled in parts by welding process. 5. Parts can be welded at reasonable cost. Limitations: 1. The process gives out harmful radiations, fumes and spatter. Hence care should be taken during welding. 2. Skilled operators are required to produce a good joint. 3. The high heat involved in the process causes changes in the structure of metal thereby lowering its properties. Applications: 1. Welding is used in the fabrication of body parts in automobile and aircraft industries. 2. Used in cylinder, boiler and vessel manufacturing. 3. Used in constructions of bridges, buildings and ships. 4. Used in machine tool industry in building various mechanical, food processing, farming, earth moving and textile machineries. 5. Used in manufacturing railway equipment. Soldering 1. Soldering is the process of joining similar or dissimilar metals by means of a filler material whose melting temperature is below 450oC. 2. The filler metal used is called a solder which is an alloy of tin and lead. 3. Ordinary gas flames or electrical soldering iron may be used to supply the required heat. 98 4. The fluxes used with soldering include rosin, zinc chloride and ammonium chloride. Application of flux cleans the surface chemically and helps the solder in making a bond. 5. Soldering is commonly used to ensure good electrical contact in electric circuits, to secure fluid tightness, sheet metal work and in sealing thin metal containers. Advantages: 1. It is a simple and economical process. 2. Dissimilar parts can be joined easily. 3. Soldered joints are easy to repair or do rework. 4. Soldering requires low energy. 5. Thin parts can be joined. Disadvantages: 1. Flux residues should be removed after soldering, otherwise it may cause corrosion. 2. Thick parts cannot be joined efficiently. 3. Soldered joints cannot be used in high temperature applications. 4. Strength of the joint is low. Brazing 1. Brazing is a process of joining similar or dissimilar metals by means of a filler material whose melting temperature is above 450oC, but below the melting point of base material. 2. The filler metal when heated melts and fills the joint cavity. On solidification and cooling of the filler metal, a strong joint is formed. 3. Filler materials used here is called spelter, which may be copper alloys, silver alloys and aluminium alloys. 4. Fluxes like borax, boric acid, fluorides etc are used to prevent oxidation of base metal and filler metal when brazing. 5. Brazing is used for fastening of pipe fittings, tanks, radiators, heat exchangers etc. Advantages: 1. Dissimilar metals and parts having thin sections can be joined easily. 2. Stronger joints are obtained when compared to soldering. 3. It is an economical and quick process. 4. Less heating is required than welding. Disadvantages: 1. Flux residues should be removed after soldering, otherwise it may cause corrosion. 2. Very large parts cannot be joined efficiently. 3. Relatively expensive filler materials. Comparison between soldering and brazing Sl no. Soldering Brazing 1 Soldering is carried out below Soldering is carried out above 450oC 450oC 99 2 Filler metal used is a lead-tin alloy Filler metals used are non ferrous alloys called solder. called spelter. 3 Flux used is usually zinc chloride, Flux used is borax powder or boric acid. rosins and ammonium chloride. 4 Weaker joints compared to brazing. Stronger joints compared to soldering. 5 Suitable to join only thin metals. Suitable to join thicker metals. 6 Cost of the process is less. Cost of the process is higher than soldering. Comparison between brazing and welding Sl no. Brazing Welding 1 Brazing is a low temperature Welding is a high temperature operation. operation. 2 Relatively weaker joints are Strong joints are produced. produced. 3 Average operator skill level is High operator skill is required. required. 4 Not an economical process. Economical compared to brazing. 5 Filler metal is of different Filler material is of similar composition as composition than the base metal. that of base metal. 6 Brazing is used to join sheet metals, Welding is used in fabrication and repair pipes, work. 100