DNA Origami PDF
Document Details
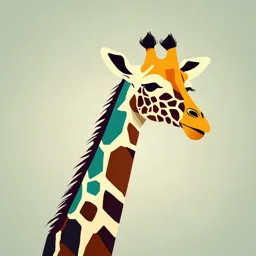
Uploaded by CommendableSard7063
Loyola College
Tags
Summary
This document provides an overview of DNA origami, a technique for folding DNA molecules into complex shapes. The method involves creating a framework (scaffold) and then attaching smaller strands (staples) to bind them, and shows promise in applications like targeted drug delivery for cancer therapy.
Full Transcript
# DNA ORIGAMI ## Introduction - The field of structural DNA nanotechnology started around 30 years ago when Ned Seeman performed pioneering research with DNA junctions and lattices. - The key player in the fast development of DNA nanotechnology was the invention of DNA origami in 2006. - The DNA or...
# DNA ORIGAMI ## Introduction - The field of structural DNA nanotechnology started around 30 years ago when Ned Seeman performed pioneering research with DNA junctions and lattices. - The key player in the fast development of DNA nanotechnology was the invention of DNA origami in 2006. - The DNA origami method is based on folding a long single-stranded 'DNA scaffold strand' into a customized shape with a set of short synthetic strands that act as 'staples' to bind the overall structure together. *Seeman, N.C. (1982) Nucleic acid junctions and lattices. J. Theor. Biol. 99, 237-247* ## Design of Scaffolded DNA Origami Five phases: Two manual design steps and three passes of the program. 1. **Generation of a block diagram** - This diagram represents a three-dimensional object, with the scaffold shown as cylinders along the helical axis. - Each cylinder represents one turn of the DNA helix, and they are placed in a grid to form a honeycomb crystal lattice. 2. **Generation of a folding path** - The folding path is a sequence of moves that the scaffold strand takes to form the final structure. - The scaffold strand is a long single-stranded DNA molecule that is typically 7,243 nucleotides long, capable of self-assembly into a designed shape. - The scaffold strand can be visualized as moving through the crystal lattice of cylinders, and the folding path is a sequence of these moves. - The folding path can be designed manually, but the program can also automatically generate it based on the block diagram. 3. **Design of staple strands** - Staple strands are short single-stranded DNA molecules that are used to hold the scaffold strand in place. - The staple strands contain sequences that are complementary to the scaffold strand. - The staple strands are typically 30-40 nucleotides in length. - These staple strands are designed to bind to the scaffold strand in the correct position. 4. **Refinement of the helical domain lengths** - Crossovers along the edges of the shape must be adjusted to minimize strain. - The twist of scaffold crossovers is calculated, and the position of the strand is adjusted (typically by one base pair) to minimize strain. - The staple sequences can be recomputed to accommodate these changes and create a better structure. 5. **Breaking and merging of strands** - Once the structure is refined, you can break and merge the staple strands. This can be done to improve the stability of the structure or to make it more flexible. - After this step, a more complex and precise structure can be designed, allowing for more complex and flexible shapes. ## One pot' reaction - ~200-250 staple and remainder strands + scaffold. - Annealed from 95°C to 20°C in a PCR machine <2h. - AFM imaging. ## Design of Three-dimensional DNA Origami - The first three-dimensional origami was designed in 2009. - This structure was a cube with a side length of approximately 40 nm. - One of the key challenges in constructing three-dimensional origami is the need to design staple strands that hold the structure together at the correct angles to each other. - The design process for three-dimensional origami is similar to the process for two-dimensional origami, with the addition of an extra dimension. ## caDNAno - This program facilitates the designing of the DNA origami. - This program can be used for: - **Slice panel**: Provides x-y cross-section view of the honeycomb lattice with helices represented as circles. - **Path panel**: Used for nucleotide-level editing of scaffold and stable-path connectivity. - **Render model**: Provides a real-time, 3D cylinder model for visualizing the shape. - **Auto-staple button**: Used to generate a default set of continuous staple paths, including crossovers. ## Role of DNA Origami in Cancer Therapy - DNA origami nanostructures are used as a biocompatible drug carrier system. - These structures can be designed to target tumor cells. - DNA origami can be used to deliver doxorubicin, a commonly used chemotherapy drug, to tumor cells. - The drug is loaded into the DNA origami structure and then delivered to the tumor cells, where it is released. - Doxorubicin is known for its cytotoxic properties that can kill cancer cells. - The use of DNA origami in cancer therapy has shown promise in clinical trials. ## DNA origami as an in vivo drug delivery vehicle for cancer therapy - DNA origami structures were effectively internalized by tumor cells. - Triangle-shaped DNA origami exhibits optimal tumor passive targeting accumulation. - M13 DNA, triangle, tube, and square DNA origami nanostructures were characterized. ## Biodistribution in a subcutaneous breast tumor model - M13 DNA and three DNA origami shapes, all incorporated with equivalent doses quantum dots, were used in this model. - Triangle DNA origami showed optimal accumulation at the tumor regions compared with square DNA origami and tube DNA origami. - Fluorescence signal was observed at the tumor sites. - In contrast, the fluorescence signal of the free QD and QD-M13 DNA at tumor sites was weak. ## Drug loading and distribution of Doxorubicin within tumor tissues - Triangle DNA origami was loaded with doxorubicin. - Accumulation of DOX and DOX/origami was observed at the tumor sites. - The fluorescence showed that there was relatively more doxorubicin at the tumor sites in the DOX/origami-treated group. - The drug delivered by DNA origami carriers, primarily surrounding blood vessels of tumor regions, was observed. - By combining passive accumulation with slow drug release in vitro, DOX/origami holds the potential to reduce the nonspecific distribution of doxorubicin during the in vivo delivery process, inducing controlled drug release in the tumor region. ## Conclusion - With the advent of DNA origami, the transition from platonic structures to nano-objects capable of performing predefined tasks and their sophisticated implementations has been extremely fast. - The advantages of using DNA-based nanostructures in therapeutics over the other accessible nanosized systems go beyond their intrinsic biocompatibility and biodegradability. - Modifications can be precisely controlled at a nanometer scale. - These superior and adjustable properties facilitate straightforward characterization of the DNA nanostructures (labeling/bioimaging), including the engineering features for targeting and releasing procedures for delivery purposes. - In summary, current and imminent DNA nanoassemblies will have a huge impact on advanced health sciences and clinical chemistry. ## Other applications - DNA structures for small interfering RNA (siRNA) delivery. - DNA structures for CpG triggered immunostimulation. - Rectangular DNA origamis coated with virus capsid proteins (CPs) for efficient cellular delivery. - A virus-inspired membrane-encapsulated spherical DNA origami vehicle for decreasing immune activation and enhancing pharmacokinetic bioavailability. - A DNA origami that can form an ion channel in a lipid membrane. - The structures that can be inserted into cell membranes, and the pores can kill cancer cells.