DNA Nanotechnology Lecture 2024 PDF
Document Details
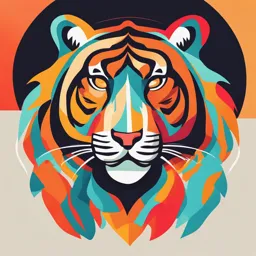
Uploaded by ComelyLaplace
Ain Shams University
2024
Sara Aly, Ph.D.
Tags
Summary
This lecture covers DNA nanotechnology, focusing on self-assembly of DNA nanostructures, 1D,2D, and 3D structures, and various types of DNA constructions like DNA Origami and nanotubes. These topics are presented with supporting diagrams and figures.
Full Transcript
Contact: [email protected] 2024 Self-Assembly of DNA Nanostructures Difference between 1D, 2D and 3D structures Difference between 1D, 2D and 3D structures Reciprocal exchange Basic tiles OR basic concepts (Key motifs) I. Via reciprocal exchange Key Motifs in St...
Contact: [email protected] 2024 Self-Assembly of DNA Nanostructures Difference between 1D, 2D and 3D structures Difference between 1D, 2D and 3D structures Reciprocal exchange Basic tiles OR basic concepts (Key motifs) I. Via reciprocal exchange Key Motifs in Structural DNA Nanotechnology. Holliday junction (HJ): a 4-arm junction that results from a single reciprocal exchange between 2 double helices. Double crossover (DX): molecule resulting from a double exchange between 2 double helices. Triple crossover (TX): molecule that results from two successive double reciprocal exchanges between 3 double helices. The HJ, the DX and the TX molecules all contain exchanges between strands of opposite polarity ( 3’ to 5’ with 5’ to 3’). Paranemic crossover (PX): molecule where two double helices exchange strands at every possible point where the helices come into proximity. JX2 molecule: molecule that lacks two of the crossovers of the PX molecule. The exchanges in the PX and JX2 molecule are between strands of the same polarity (i.e. 3’ to 5’ and 3’ to 5’ ) Basic Concepts (Key motifs) II. Via Combination of branched motifs and sticky ends Each of the four double helical arms has been tailed by a sticky end. Sticky-ended cohesion of DNA is the most predictable intermolecular interaction of any known molecular system, in the senses of affinity, diversity and structure. A quadrilateral could be assembled by the cohesion of the sticky ends tailing four of these junctions. Moreover, there are also sticky ends visible on the outside of the structure, so that this arrangement could be extended to yield 2D and 3D crystalline arrays. Thus, in principle, branched DNA molecules (tiles) tailed in sticky ends can be assembled into 2D and 3D objects and lattices. Two-Dimensional DNA Array Structures 1.DNA Lattice Structures A DNA triangle tile that is composed of three four-arm junctions is shown on the left, and a two-dimensional array that was formed by self- assembly of these DNA triangles is shown on the right. Two-Dimensional DNA Array Structures 1. DNA Lattice (Array) Structures (cont.) Schematic drawing and atomic force micrograph of a single hexagonal structure composed of six equilateral triangle complexes that are attached to one another. Two-Dimensional DNA Array Structures 1.DNA Lattice (Array) Structures (cont.) Schematic drawing and atomic force micrograph of a 5 x 5 fixed- size array that was assembled from a cross-shaped tile structure with C4 symmetry. Two-Dimensional DNA Array Structures 1.DNA Lattice Structures (cont.) A three-pointed star DNA motif is shown on the left, and hexagonal two-dimensional DNA lattices that were formed by self- assembly of three-point-star motifs with sticky ends are shown on the right. Two-Dimensional DNA Array Structures 2.DNA Origami Structures Arbitrary, complex, two-dimensional shape can be fabricated using a long, single-stranded DNA from a virus with a known genetic sequence (Rothemund, 2006). Two-Dimensional DNA Array Structures 2. DNA Origami Structures (cont.) For example, Rothemund chose a 7 kilobase circular genomic DNA from the virus M13mp18 as a scaffold material. In order to hold the scaffold in place, hundreds of short (30 to 70 nucleotide long) ‘staple’ DNA strands were used to attach multiple parts of the long scaffold strand. Two-Dimensional DNA Array Structures 2.DNA Origami Structures (cont.) Each ‘staple’ strand has two arms, which have independently complementary bases to two different sequences of the long scaffold strand. As a result, these strands may be used to fold the long scaffold strand back and forth into any desired shape. Two-Dimensional DNA Array Structures 2. DNA Origami Structures (cont.) As a result, complex patterns, including words or images, may be formed. Two-Dimensional DNA Array Structures 2. DNA Origami Structures (cont.) Two-Dimensional DNA Array Structures 3. DNA Nanotube Structures DNA nanotubes are a promising template for biomimicry (Nanotubes are used for the reproduction of microtubules, which serve as structural components within cells). Two-Dimensional DNA Array Structures 3. DNA Nanotube Structures The DAE-E tile contains five DNA strands that hybridize into a rigid rectangular core, in which there is a single-stranded five-base long sticky end at each corner. Two-Dimensional DNA Array Structures 3. DNA Nanotube Structures Three-Dimensional DNA Nanostructures 1. Tetrahedra A family of rigid three-dimensional DNA tetrahedra was constructed, which possessed edge lengths of 10 nm (Goodman et al., 2005). These structures self- assembled in seconds, and could be connected by programmable DNA linkers. Three-Dimensional DNA Nanostructures 1. Tetrahedra Each tetrahedron was formed from four short single DNA stands, which were designed to favor the construction of 3 tetrahedron edges in a 2 1 hierarchical molecular model. 4 Three-Dimensional DNA Nanostructures 2. DNA cubic box with a controllable lid It was designed by Andersen et al., they used an entire M13 strand that was divided into six sections, and each section was used to build one sheet (face). Three-Dimensional DNA Nanostructures 2. DNA cubic box with a controllable lid Two neighboring faces in the box were connected by the “hinge” strands on corresponding sheet edges. Subsequently the entire box was formed by hinging six interconnected sheets. The lid was functionalized with a lock–key system to control its opening which could be read out by FRET switches. Three-Dimensional DNA Nanostructures The above DNA origami 3D nanostructures were all constructed by. This design principle is simple and straightforward Since planar DNA origami has intrinsic flexibility, it would be difficult to build rigid and various 3D nano-objects. Three-Dimensional DNA Nanostructures The next breakthrough in this field was reported by Shih’s group. They used a different strategy to achieve the building of custom 3D shapes. The key in their design principle is that the 3D shapes are composed of.