Copy of Histology-Nervous System chp1 4.pdf
Document Details
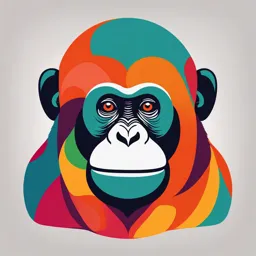
Uploaded by Lynn abi aad
Alte University
Tags
Related
- BMS2-1 Central Nervous System Histology-Dr. Gözde Öğütçü 08.11.2022 -10_00-11_50. (1).pdf
- Copy of Histology-Nervous System chp1.pdf
- Copy of Histology-Nervous System chp1 2.pdf
- Copy of Histology-Nervous System chp1 3.pdf
- LECTURE 3 - Histology of Central and Peripheral Nervous System.pdf
- Central Nervous System Histology and Development PDF
Full Transcript
Choroid Plexus The choroid plexus consists of highly vascular tissue, elaborately folded and projecting into the large ventricles of the brain. It is found in the roofs of the third and fourth ventricles and in parts of the two lateral ventricular walls, all regions in which the ependymal lining dir...
Choroid Plexus The choroid plexus consists of highly vascular tissue, elaborately folded and projecting into the large ventricles of the brain. It is found in the roofs of the third and fourth ventricles and in parts of the two lateral ventricular walls, all regions in which the ependymal lining directly contacts the pia mater. A decrease in the absorption of CSF or a blockage of outflow from the ventricles during fetal or postnatal development results in the condition Each villus of the choroid plexus contains a thin layer of well-vascularized pia mater known as hydrocephalus covered by cuboidal ependymal cells. (Gr. hydro, water + kephale, head), which promotes a progressive The function of the choroid plexus is to remove water from blood and release it as enlargement of the head followed by the CSF. CSF is clear, contains Na+, K+, and Cl– ions but very little protein, and its mental impairment. only cells are normally very sparse lymphocytes. It is produced continuously and it completely fills the ventricles, the central canal of the spinal cord, the subarachnoid and perivascular spaces. It provides the ions required for CNS neuronal activity and in the arachnoid serves to help absorb mechanical shocks. Arachnoid villi provide the main path- way for absorption of CSF back into the venous circulation. There are very few lymphatic vessels in CNS tissue. PERIPHERAL NERVOUS SYSTEM The main components of the peripheral nervous system (PNS) are the nerves, ganglia, and nerve endings. Peripheral nerves are bundles of nerve fibers (axons) individually surrounded by Schwann cells and connective tissue. Nerve Fibers Nerves are analogous to tracts in the CNS, containing axons enclosed within sheaths of glial cells specialized to facilitate axonal function. In peripheral nerves, axons are sheathed by Schwann cells or neurolemmocytes. The sheath may or may not form myelin around the axons, depending on their diameter. Myelinated Fibers As axons of large diameter grow in the PNS, they are engulfed along their length by a series of differentiating neurolemmocytes and become myelinated nerve fibers. The plasma membrane of each covering Schwann cell fuses with itself at an area termed the mesaxon and a wide, flattened process of the cell continues to extend itself, moving circumferentially around the axon many times. The multiple layers of Schwann cell membrane unite as a thick myelin sheath. Composed mainly of lipid bilayers and membrane proteins, myelin is a large lipoprotein complex that, like cell membranes, is partly removed by standard histologic procedure. Unlike oligodendrocytes of the CNS, a Schwann cell forms myelin around only a portion of one axon. With high-magnification TEM, the myelin sheath appears as a thick electron-dense axonal covering in which the concentric membrane layers may be visible. The prominent electron-dense layers visible ultrastructurally in the sheath, the major dense lines, represent the fused, protein-rich cytoplasmic surfaces of the Schwann cell membrane. Along the myelin sheath, these surfaces periodically separate slightly to allow transient movement of cyto- plasm for membrane maintenance; at these myelin clefts (or Schmidt-Lanterman clefts), the major dense lines temporarily disappear. Faintly seen ultrastructurally in the light staining layers are the intraperiod lines that represent the apposed outer bilayers of the Schwann cell membrane. Membranes of Schwann cells have a higher proportion of lipids than do other cell membranes, and the myelin sheath serves to insulate axons and maintain a constant ionic micro- environment most suitable for action potentials. Between adjacent Schwann cells on an axon, the myelin sheath shows small nodes of Ranvier (or nodal gaps), where the axon is only partially covered by interdigitating Schwann cell processes. At these nodes, the axolemma is exposed to ions in the interstitial fluid and has a much higher concentration of voltage-gated Na+ channels, which renew the action potential and produce saltatory conduction (L. saltare, to jump) of nerve impulses, their rapid movement from node to node. The length of axon ensheathed by one Schwann cell, the internodal segment, varies directly with axonal diameter and ranges from 300 to 1500 µm. Unmyelinated Fibers Unlike the CNS where many short axons are not myelinated at all but course among other neuronal and astrocytic processes, the smallest diameter axons of peripheral nerves are still enveloped within simple folds of Schwann cells. These very small unmyelinated fibers do not however undergo multiple wrapping to form a myelin sheath. In unmyelinated nerves, each Schwann cell can enclose portions of many axons with small diameters. Without the thick myelin sheath, nodes of Ranvier are not seen along unmyelinated nerve fibers. Moreover, these small-diameter axons have evenly distributed voltage-gated ion channels; their impulse conduction is not saltatory and is much slower than that of myelinated axons. portion of myelin in which the major dense lines of individual membrane layers can be distinguished, as well as the neurofilaments (NF) and microtubules (MT) in the axoplasm (A). At the center of the photo is a Schwann cell showing its active nucleus (SN) and Golgi-rich cytoplasm (SC). At the right is an axon around which myelin is still forming Unmyelinated axons (UM) are much smaller in diameter, and many such fibers may be engulfed by a single Schwann cell (SC). The glial cell does not form myelin wrappings around such small axons but simply encloses them. Whether it forms myelin or not, each Schwann cell is surrounded, as shown, by an external lamina containing type IV collagen and laminin like the basal laminae which underlie epithelia. longitudinally oriented nerve shows one node of Ranvier (N) with the axon visible. Col- lagen of the sparse endoneurium (En), blue in this trichrome stain, surrounds the Schwann cells and a capillary (C). At least one Schwann cell nucleus (S) is also clearly seen. (X400; Mallory trichome) The axon is enveloped by the myelin sheath, which, in addition to membrane, contains some Schwann cell cytoplasm in spaces called Schmidt-Lanterman or myelin clefts between the major dense lines of membranes. The upper diagram shows one set of such clefts ultrastructurally. The clefts contain Schwann cell cytoplasm that was not displaced to the cell body during myelin formation. This cytoplasm moves slowly along the myelin sheath, opening temporary spaces (the clefts) that allow renewal of some membrane components as needed for maintenance of the sheath. The lower diagram depicts the ultrastructure of a single node of Ranvier or nodal gap. Interdigitating processes extending from the outer layers of the Schwann cells (SC) partly cover and contact the axolemma at the nodal gap. This contact acts as a partial barrier to the movement of materials in and out of the periaxonal space between the axolemma and the Schwann sheath. The basal or external lamina around Schwann cells is continuous over the nodal gap. The axolemma at nodal gaps has abundant voltage-gated Na+ channels important for impulse conductance in these axons. Nerve Organization In the PNS, nerve fibers are grouped into bundles to form nerves. Except for very thin nerves containing only unmyelinated fibers, nerves have a whitish, glistening appearance because of their myelin and collagen content. Axons and Schwann cells are enclosed within layers of connective tissue. Immediately around the external lamina of the Schwann cells is a thin layer called the endoneurium, consisting of reticular fibers, scattered fibroblasts, and capillaries. Groups of axons with Schwann cells and endoneurium are bundled together as fascicles by a sleeve of perineurium, containing flat fibrocytes with their edges sealed together by tight junctions. From two to six layers of these unique connective tissue cells regulate diffusion into the fascicle and make up the blood-nerve barrier that helps maintain the fibers’ microenvironment. Externally, peripheral nerves have a dense, irregular fibrous coat called the epineurium, which extends deeply to fill the space between fascicles. Very small nerves consist of one fascicle. Small nerves can be found in sections of many organs and often show a winding disposition in connective tissue. Myelin maintenance and nodes of Ranvier. Peripheral nerves establish communication between centers in the CNS and the sense organs and effectors (muscles, glands, etc). They generally contain both afferent and efferent fibers. Afferent fibers carry information from internal body regions and the environment to the CNS. Efferent fibers carry impulses from the CNS to effector organs commanded by these centers. Nerves possessing only sensory fibers are called sensory nerves; those composed only of fibers carrying impulses to the effectors are called motor nerves. Most nerves have both sensory and motor fibers and are called mixed nerves, usually also with both myelinated and unmyelinated axons. Ganglia Ganglia are typically ovoid structures containing neuronal cell bodies and their surrounding glial satellite cells supported by delicate connective tissue and surrounded by a denser capsule. Because they serve as relay stations to transmit nerve impulses, at least one nerve enters and another exits from each ganglion. The direction of the nerve impulse determines whether the ganglion will be a sensory or an autonomic ganglion. Sensory Ganglia Sensory ganglia receive afferent impulses that go to the CNS. Sensory ganglia are associated with both cranial nerves (cranial ganglia) and the dorsal roots of the spinal nerves (spinal ganglia). The large neuronal cell bodies of ganglia are associated with thin, sheetlike extensions of small glial satellite cells. Sensory ganglia are supported by a distinct connective tissue capsule and an internal framework continuous with the connective tissue layers of the nerves. The neurons of these ganglia are pseudounipolar and relay information from the ganglion’s nerve endings to the gray matter of the spinal cord via synapses with local neurons. Autonomic Ganglia Autonomic nerves affect the activity of smooth muscle, the secretion of some glands, heart rate, and many other involuntary activities by which the body maintains a constant internal environment (homeostasis). Autonomic ganglia are small bulbous dilations in autonomic nerves, usually with multipolar neurons. Some are located within certain organs, especially in the walls of the digestive tract, where they constitute the intramural ganglia. The capsules of these ganglia may be poorly defined the local connective tissue. A layer of satellite cells also envelops the neurons of autonomic ganglia although these may also be inconspicuous in intramural ganglia. Autonomic nerves use two neuron circuits. The first neuron of the chain, with the preganglionic fiber, is located in the CNS. Its axon forms a synapse with postganglionic fibers of the second multipolar neuron in the chain located in a peripheral ganglion system. The chemical mediator present in the synaptic vesicles of all preganglionic axons is acetylcholine. Neuronal cell bodies of preganglionic sympathetic nerves are located in the thoracic and lumbar segments of the spinal cord and those of the parasympathetic division are in the medulla and midbrain and in the sacral portion of the spinal cord. Sympathetic second neurons are located in small ganglia along the vertebral column, while second neurons of the parasympathetic series are found in very small ganglia always located near or within the effector organs, for example in the walls of the stomach and intestines. Parasympathetic ganglia may lack distinct capsules altogether, perikarya and associated satellite cells simply forming a loosely organized plexus within the surrounding connective tissue. Neural Plasticity & Regeneration Certain regions of the CNS, such as near the ependyma, retain rare neural stem and progenitor cells that allow some replacement of neurons throughout life; neural plasticity involving formation and remodeling of synaptic connections is also prevalent throughout life. The complexity and distances of the neuronal and glial interconnections with the CNS make regeneration and restoration of function within this tissue after major injury very difficult. The more simply organized peripheral nerves have better capacity for axonal regeneration, a process involving reactivation of the peri- karyon, Schwann cells, and macrophages. NEURAL PLASTICITY & REGENERATION Despite its general stability, the nervous system exhibits neuronal differentiation and formation of new synapses even in adults. Embryonic development of the nervous system produces an excess of differentiating neurons, and the cells which do not establish correct synapses with other neurons are eliminated by apoptosis. In adult mammals after an injury, the neuronal circuits may be reorganized by the growth of neuronal processes, forming new synapses to replace ones lost by injury. Thus, new communications are established with some degree of functional recovery. This neural plasticity and reforma- tion of processes are controlled by several growth factors pro- duced by both neurons and glial cells in a family of proteins called neurotrophins. Neuronal stem cells are present in the adult CNS, located in part among the cells of the ependyma, which can supply new neurons, astrocytes, and oligodendrocytes. Fully differentiated, interconnected CNS neurons cannot temporarily disengage these connections and divide to replace cells lost by injury or disease; the potential of neural stem cells to allow tissue regen- eration and functional recovery within the CNS components is a subject of intense investigation. Astrocytes do proliferate at injured sites and these growing cells can interfere with success- ful axonal regeneration in structures such as spinal cord tracts. In the histologically much simpler peripheral nerves, injured axons have a much greater potential for regeneration and return of function. If the cell bodies are intact, damaged or severed PNS axons can regenerate as shown in the sequence of diagrams in Figure 9–30. Distal portions of axons, isolated from their source of new proteins and organelles, degenerate; the surrounding Schwann cells dedifferentiate, shed the myelin sheaths, and proliferate within the surrounding layers of con- nective tissue. Cellular debris including shed myelin is removed by blood-derived macrophages, which also secrete neurotroph- ins to promote anabolic events of axon regeneration. The onset of regeneration is signaled by changes in the perikaryon that characterize the process of chromatolysis: the cell body swells slightly, Nissl substance is initially dimin- ished, and the nucleus migrates to a peripheral position within the perikaryon. The proximal segment of the axon close to the wound degenerates for a short distance, but begins to grow again distally as new Nissl substance appears and debris is removed. The new Schwann cells align to serve as guides for