Global Energy Trend Overview PDF
Document Details
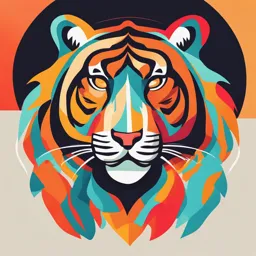
Uploaded by ConsistentObsidian4053
Politecnico di Milano
Tags
Summary
This document provides an overview of global energy trends, focusing on exploration's role in energy transition. It details pre-drill phases, risk assessments, and project maturation, highlighting the importance of exploration in a world of growing energy needs and the transition away from fossil fuels. Furthermore, it contains information about geological concepts and principles.
Full Transcript
Contents: GLOBAL ENERGY TREND OVERVIEW o How Exploration contributes to energy transition EXPLORATION IN O&G BUSINESS o Pre-drill phases o Risk assessment and economics o Post-drill and development project opportunity...
Contents: GLOBAL ENERGY TREND OVERVIEW o How Exploration contributes to energy transition EXPLORATION IN O&G BUSINESS o Pre-drill phases o Risk assessment and economics o Post-drill and development project opportunity maturation [5-13] How exploration contributes to energy transition Energy is required for every single daily activity. Furthermore, world is growing so energy requirements are increasing. Oil and gas contributes to more than 50% of the energy mix of the world @ 2022. Energy transition is a fundamental to transition away from fossil and adopt renewable sources. Anyway energy transition needs time and needs to be sustainable in a scenario of growing people. Without exploration it is difficult to sustain a scenario in which oil and gas are predominant, even though production is declining and will further decline in the next 10 years (in particular concerning oil). It needs time to replace 80% of fossil fuels (including coal), so exploration is still important. Resilient exploration will deliver advantaged resources that can be on production within five years from the discovery (short track time), have a low break even price (economically sustainable) and be producible with net zero upstream emission (low carbon risk) Hydrocarbon production will shrink in the next years, gas will be the bridge fuel and carbon capture utilization and storage will be a key factor. [9-10] [13-21] Exploration objectives and pre-drill phases Exploration is searching for undrilled and undiscovered hydrocarbon resources in the ground through geological and geophysical studies. Fundamental parameters are: Original hydrocarbon in place (MBOE, Mega Barrel of Oil Equivalent) -> volume POS % (possibility to find HC) -> probability of success Exploration Contingent Resources are discovered volumes not yet under production. Exploration wells (New Field Wildcat NFW or Appraisal) have to be drilled to declare our discovery After exploration dedicated engineering studies need to be developed (development project). The hydrocarbon amount that can be produced is called Reserve (MBOE) the recovery factor (%) is the ratio between them reserve and original hydrocarbon in place. The typical gas recovery factor is between 60-85%, while for oil it is lower between 10-45%. The typical workflow consists in three plus one phases: see 240617 (m.1) - Upstream project development (4.4) Exploration. Exploration can be divided in three phases: o New venture and contract ( 0 ) or Monitor underground storage of CO2. The final goal is to decrease the risk when making reservoir development and production decisions. Contents: What is geology Sedimentary basins Geological time concept Principles of stratigraphy Petroleum system [2-19] What is geology Geology is the study of the Earth's form and composition, and the changes that has undergones Earth structure is composed by Crust 0-80 km. It is divided between continental and oceanic crust (under the ocean). The crust is composed of plates. Continental drift causes continuous change in continent location and shape. Plate boundaries can be: o Divergent: material moving up in between, upwelling magma. Ridge forms in the ocean (mid-ocean ridge), rift zone on the land. o Convergent: one is subducting. Earthquakes happen. Himalayas are convergent setting, originated from India collapsing to Eurasian plate. o Transform: shear one to other. Strike slip setting. Mantle 2900 km thick: characterized by convective motions of the endogenic material and causing endogenic forces Outer core 2250 km thick Inner core 1200 km thick [20-30] Sedimentary basins Sedimentary basins are regions of the Earth where long-term subsidence creates accomodation space for accumulation of sediments. Rocks are made of minerals. They are classified in : Igneous (granite, gabbro, pumice, obsidian). They are non-poreous rocks Sedimentary (sandstone, limestone, shale, conglomerate, gypsum). Poreous rock. Reservoir if underwater (?) Sedimentary rocks can form in diverging or convering zones. Metamorphic (marble, slate, quartzite, gneiss). They are non-poreous rocks. They crystalized with heat and pressure. [31-33] Geological time concept From ancient to more recent: Cenozoic Mesozoic Paleozoic Precambrian (quite unknown) [34-38] Principles of Stratigraphy 1. Principle of Original Horizontality. Sediments are deposited in flat, horizontal layers. 2. Principle of superposition. The oldest layers lower than the younger layers, reflecting the mechanical deposition of sediments. All new layers cover the previous and older ones. 3. Principle of original lateral continuity. The sediments being deposited on the seafloor will be a layer that extends for a considerable distance in all direction: layers are laterally continuous 4. Principle of actualism. Uniformitarianism is the assumption that the natural processes operating in the past are the same as those that can be observed in the present [39-48] Petroleum system See 240611 (m.1) - Intro to Exploration (4.2): Play and prospect Additional infos from 240612 (m) - Reservoir (4.3) Contents Energy transition New exploration for Energy Transition CCS: a G&G perspective [3-7] Energy transition and Eni's approach Energy transition for a sustainable development: include following the 17 SDGs included in the 2030 agenda for sustainable development adopted by United Nations (2015) Energy trilemma: see 240625 (p.2) - NG techs (4.6) - Gas advocacy Secure & reliable - energy security Sustainable & clean - energy sustainability Accessible & affordable - energy economics The goal is carbon neutrality by 2050 Full decarbonization process involves different leverages: biorefineries, circular processes, blue and green hydrogen, improved efficiency and digital solutions, renewables, gas as transition fuel and natural and artificial carbon capture. [7-] New exploration for Energy Transition Exploration is still needed (see 240611 (m.1) - Intro to Exploration (4.2)) : not only to extract gas, but also to provide critical elements that are used in renewables technology (litium, cobalt, nichel, Rare Earth Elements) with connected emissions [10-11], And to exploit the potential of geothermics ("extract" heat and steam). These new challenges can be superated with advanced technologies and computational power (HPC6, 600 PFlops). [15-] CCS: a G&G (Geological & Geophysical) perspective CCS is the process of capturing waste carbon dioxide, transporting it to a storage site and depositing it where it will not enter the atmosphere, normally an underground geological formation. Global annual CO2 emissions is 37 billion tons (37 Gton/y) @ 2021. To reach NZE by 2050, 6 billions tons per year (6 Gton/y) are required from CCS. Carbon capture is vital to reduce emissions to net zero and achieve global climate change targets : to meet climate targets more than 100 Gton CO2 should be stored, versus the 12 Gton maximum theoretical potential of current CCS project pipeline (??) The sectors of source of CO2 to be reinjected are: hard-to-abate industry (cement, iron, steel), waste to energy, power generation, hydrogen/chemical and fertilizers/ethanol production, natural gas processing. [21-24] Storage: basic concepts Geological Reservoir can be used as permanent storage: saline acquifers, depleted fields (and others). The requirements for geological storage are: sufficient storage capacity, the presence of a seal to avoid escape, adequate transport and injectivity facilities, possibility to monitor levels. CO2 deep reservoirs are at supercritical state. To avoid phase changes reservoir should be deeper than 800 meters. Supercritical state is advantages because the density is liquid but the viscosity is gas, so it takes the best from both states. CO2 is lighter than water, so it goes up like hydrocarbons, and it is not inert (it can react). It can be trapped by: mineralization or mineral trapping (occurs when dissolved CO2 reacts with minerals in the surrounding rock to form stable, solid carbonate minerals, such as calcite CaCO₃; requires 1.000 to 10.000 years), dissolution (CO2 diffuses and dissolves into the surrounding water, forming carbonic acid H₂CO₃), residual trapping (CO2 gets physically trapped in the pore spaces of the rock due to capillary forces) or free gas accumulation in structural trapping. At first injection, most of CO2 is free gas that accumulates in structural & stratigraphic trapping (below source rocks). With years passing by, the other mechanism occur, increasing storage security. [22-23] CO2 might affect the subsurface generating stress, strain and deformation, and in some cases causing unwanted mechanical changes [25-30] 1. Find a storage site, characterize it Site screening is the process used to find CO2 storage sites. Screening is based on Identification Characterization (petrophysical properties) and Volumetrics preliminary estimates Formulas are similar to that seen in 240611 (m.1) - Intro to Exploration (4.2) o For depleted reservoirs the assumption is that the pore space, once filled by hydrocarbons, can now be filled by CO2. o In saline aquifer no hydrocarbon production has depressurized the reservoir, but the assumption is that CO2 replaces water in the pore spaces. The capacity coefficient is site-specific and is a small fraction of the available pore (0.5-5%) Beside geological factors, other factors influence the necessity for CCS The presence of CO2 emitter and the distance Government policy and regulation, driving investment: ETS, carbon taxes, … [31-40] 2. Model the plume (and pressure…) The 3D Geological Model must consider seismic derived geomechanical parameters, sedimentological model and structural model. Geological model must consider (and analyse) Fault stability: avoid fault initiation and spread Thermally induced fracture (TIF) Cap rock integrity Preliminary works include: evaluating the migration of CO2 on basin scale evaluating the pore pressure and pre-injection stress field It is important that the pore pressure does not exceed failure, otherwise the stress field inside the basin can lead to mechanical alteration in the subsurface. Another special study from Eni is CO2 reactive modelling [41-] 3. Operate and monitor the site Measurement, monitoring and verification (MMV) is an important step of a project, required by worldwide legislations. Monitoring can be divided in: Site storage monitoring: plume evolution, leakage prevention, storage complex characterization & injection monitoring (through gravimetric monitoring based on density monitoring vs water: not always feasible and applicable). The European directive on carbon capture and storage stress the importance of analyzing bigger area then the couplet reservoir-caprock: storage complex = storage site (defined volume) + surrounding geological domain Main monitoring activities include: ground deformation monitoring, geomechanical response monitoring: microseismic monitoring (detection of small cracks) and fiber optic borehole seismic monitoring, and 4D seismic monitoring. Environmental monitoring: leakage detection and remediation The detection of CO2 leaks is usually difficult due to high natural "noise". Gas bubbles and pH variations in the sea could be an indicator. [] CCS: Pros and Cons Pros: With CCS we can reach negative emissions Only solution for hard-to-abate Use of O&G skills and know-how Cons: Strong CAPEX, also related to CO2 transport Clear regulatory framework is required [] Eni strategy and Business cases Eni is planning 40 Mton/y of CO2 stored by CCS post-2030 (vs 6000 Mton required!) Solution are studied for hard to abate and upstream sectors. Business cases: Hynet North West (for hard-to-abate industries) Bacton Thames Ravenna CCS Ex-Neptune [1-18] Fast track approach Upstream activity cycle: see 240617 (m.1) - Upstream project development (4.4) Time to market is the time from discovery to the start of production. It includes the appraisal well and the development plan. The fast track or phased approach is an approach to fast up the time to market to less than five years and used to de-risk the full field exploitation. It involves strong integration between discipline and parallel (non sequential!) approach. It consists in developing while appraising: once I discover a field, I start production and while producing I appraise another well, and so on. These approach: Improve Rate of Returns (break-even point happens earlier in time) Reduce medium-long term price scenarios uncertainties, and Reduce the financial exposure (minimum NPV), since revenue finance other phases [19-29] Reservoir Role in HC Exploitation A simplified reservoir scheme is based on layers of rock saturated with different phases, along the vertical: gas is the lighter phase on the top, then oil and then water on the bottom. See 240611 (m.1) - Intro to Exploration (4.2) for: The concepts of volume (how much do I have: OHIP or Exploration Contingent Resources), reserves (how much can I get: percentage of discovered HC that is producible and sustainable), production (shape of production rate over time) Calculation of Original Hydrocarbon in Place (OHIP) [30-] Reservoir models Moka is a model of a reservoir: gas is pushing from top to down on oil, that is helped in going up to the upper chamber. To reconstruct a reservoir, few data are available. We have seismic surveys on the larger scale (10^3 to 10^6 m), on a smaller scale we have well test, logs, … All these data helps in constructing a model of the reservoir based on complex numerical model (structured grid and properties). The models helps in calculating the volume of hydrocarbon in place and to generate the production profiles. Echelon is a commercial reservoir simulator built for scratch for GPU to exploit high performance computational speed. Echelon is used to preserve natural geology, to deal with complex physics and to manage uncertainty. [33-34] Results on the fields confirms Echelon performance. Echelon allows for real time operation: update the model while drilling the well - geosteering (technique used in the drilling of oil and gas wells, particularly in horizontal and directional drilling, to precisely navigate and control the wellbore's path in real-time to target specific subsurface formations or reservoirs. The goal of geosteering is to optimize the placement of the well within a desired geological formation). Reservoir activities are also working with Artificial Intellicence for robust optimization issues: preserve geological consistency, monitor well and reservoir performance, optimize well locations, production and injection strategies. [54-] Reservoir Role in Energy transition The same tools and workflows for reservoir characterization can be used in support of other projects: CO2 storage (see integration in 240611 (p.2) - CCS (4.2)) H2 storage (Underground Hydrogen Storage UHS) (see 240613 (p) - Tech innovation (4.3)) Blue H2 production can be planned, so H2 storage depends on production volume and usage. Otherwise, green H2 comes from RES so it cannot be planned: H2 storage is needed, and subsurface may play a major role H2 storage becomes competitives for long cycles: for short charge/discharge cycles, batteries are more efficient. Storage sites are the same as CO2 and NG: o Salt caverns: from a structural and functional point of view they are the best: impervious walls, easy charge and discharge, flexible and modular. The main disadvantages are restricted volume, leakages and impurities that may trigger reactions. o Saline acquifers o Depleted reservoirs: large volume available. The main issues are related to the controllability: possible H2 losses, interaction with bacteria and chemical reactions -> it is difficult to get back pure H2 Geothermal energy: inject cold water, produce/extract hot water. "geothermal water" is used in a heat exchanger to heat up the technical water used for district heating. Contents: Introduction o D&C activities as part of the UPS process Well drilling and Completion o Technical overview of well construction process o Drilling rigs and equipment o Drilling control and well data o Well drilling problems o Well construction contracts Technology innovation in drilling o Role of tech innovation o Innovation general processes and agreements o Intellectual property o Drilling safety package [4-] D&C activities as part of the UPS process Well operations take all the phases of the business cycle, from exploration start to decommissioning D&C involves different environment Remote Desert Offshore and deepwater Arctic Well engineering involves both Exploration/Reservoir (geological and pressure prediction) and Development+Production (environmental and facilities constraints) Well construction and engineering are two-way continuous optimisation Well completion makes possible the HC production [9-36] Technical overview of well construction process A well is composed by Christmas tree: it allows to open and close the stream. Wellhead (below christmas tree). It is the connection part between the christmas tree and the pipe. Casing and cement (around the well pipe) to protect the structure from collapsing Completion: is the most costly part, connection between reservoir and christmas tree. Drilling is performed during the different phases for different purposes: Exploration or appraisal: to identify the presence of a reservoir Development: produce hydrocarbon Injection / disposal: to reinject produced water or gas, supporting the reservoir pressure Relief: to intercept an uncontrolled flowing formation and kill a well in blow out. Pore pressure is the pressure of fluids contained in the rocks cavities (pore). Pressure regimes can be: normal (hydrostatic: linearly increasing pressure with depth), overpressure or underpressure. Pore gradient is the derivative of pore pressure with depth. The formula can be extended: Pore gradient = pore pressure / (depth * 10) [ (kg/cm^2) / 10m] Normal pressure regime -> constant pore gradient Overpressure regime -> increasing pore gradient with depth Underpressure regime -> decreasing pore gradient with depth Casing setting depths, casing size, mud densities are defined together as they are strictly mutually dependent When drilling a development well, the target is achieve the maximum recovery of HC reserves in complete safety, shorter time, lowest investment Different wells trajectories can be adopted: the focus is reducing the environmental impact and soil consumption. Examples of directional profiles : Vertical Slant (inclined from a certain vertical depth and down) S-shaped (vertical-inclined-vertical again) Horizontal, through small curvature over hundred meters to km. Extended reach: put the well onshore to take oil and gas from the subsea surface. Well drilling process. Three conditions to maintain Weight on bit (WOB): axial force. The WOB can be computed by subtracting the hookload (with free bit or "off bottom") by the hookload (with bit on the location of drilling or "on bottom"). The instrument is Martin Decker Weight Indicator Rotation of the drill string Circulation of mud: mud injected from the axis, going out toward the outside at the bit height The drilling sequence [25-28] consists in adding new stand between drill string and top drive until the final depth is reached. Drilling fluid. The main function of the drilling fluid is to provide hydrostatic pressure to contain the formation pressure (pressure pushing toward the inside of the hole) and prevent influx into the well. Other function are prevent instability problems, transport the cuttings to the surface and lubricate drill string. At every depth, the mud pressure should be higher than the pore pressure but lower than fraction pressure. The same for the gradients. [31-32] If the mud pressure is too low, there is an underbalance so we have influx of formation fluids from the outside; while if the mud pressure is too high we have breaking of formation and mud losses. Cementing is performed combining: cement powder with water and additives. Cement should be the right density, easy to pump and with the right thickening time (should not solidify too early or too late). Well completion [34-35]. After the well is drilled, a production tubing of a selected material is run and set in the well in order to bring hydrocarbons from reservoir to surface in a safe manner. We can have: Open hole Cased hole (hole in the tubing) Well completions typologies are: Single: one flowing zone Dual: two independent flowing zones at the same time Intelligent: two or more communicating flowing zones for the same bore with remote flow control system. Valve can open or close some zones Multilateral: two or more communicating flowing zones (different bores) [37-49] Drilling rigs and equipment Main equipment of a drilling rig are : Hoisting and rotation [40-41] The hoisting system sustain the drill string, allowing to run in hole, pull out of hole and providing WOB during operations. It involves a drawwork (argano) The rotation system provide torque and rotation to the drilling string (top drive system) Surface well control BOP (Blow Out Preventer), Ram preventer and Annular preventer. Prevent occurrence of uncontrolled flow, close and seal on the drill pipe (ram preventer); close and seal on open holes. The function of Well Barriers, adopted in case of emergency , is to maintain full control of formation fluids at all times. At least two independent WB's shall be present at all times during the execution of well operations: Two Barriers Policy Mud circulation (mud storage, pumps, …) It supply the necessary pressure and flow rate for the circultaion of mud in the wellbore. It's a closed loop! Power generation system. One or more power generators that feed the mud pump, the drawworks and the top drive. There are battery packs to supply energy for x days. From 240611 (m.1) - Intro to Exploration (4.2) The drilling rig technology may involve different equipments depending on Max operating water depth: see Dispense introduttive 05 - well drilling & completion Landrig for on-shore Drilling barge for shallow water Bottom sea supported o Platform Rig up to 150/200 m depth o Jack-up (supporting legs, 3 legs that can be moved up and down) for up to 150 m Floaters: semi-sub for wavy sea, drilling ship when not so wavy o Semi-sub: usually anchored for depth up to 1000/1500 m, dynamic positioning for up to 3000m o Drilling ship (dynamic position, subsceptible to sea motions) for up to 3600 m Different power classes according to the max depth: Class I: up to 2500m, 700 hp Class II: up to 3500m, 1000 hp Class III: up to 4500m, 1500 hp Class IV: up to 5500m, 2000 hp Class V: more, 3000 hp [50-52] Drilling control and well data During the drilling operations all the drilling parameters (pressure, volume, temperature, density, flow rate, lithologies, formation fluids, …) are kept under control and monitored in real time by means of surface instrumentation and downhold instrumentation. Data transmission is performed via Telemetry systems allowing real-time control. [53-64] Well drilling problems Three are the main drilling problems, posing problems for safety, environment, loss of asset integrity, company reputation. They also constitute NPT, so it's a problem of lost time and so lost revenue. 1. Formation fluid influx (kick). A kick is an entry of water, gas, oil or other formation fluid into the wellbore during drilling. It happens when the mud pressure is not enough to contain the formation pressure. A blowout may occur. The solution is activating the BOP preventer. 2. Mud losses Losses can be partial or total. Common causes are mud pressure higher than frac pressure, or high porosity in the formations. Solutions can be circulate lower mud density, or seal of loss zone with cement. 3. Stuck pipe. A pipe can stuck due to differential pressure sticking (e.g. when mud weight is too high), or as borehole collapse or geometry. Recovery actions are mechanical actions with drill string tools as jar or bumper, and specific mud pills (lubricant effect, or acid to free some formations) Operations are divided in three time coding Planned operations (code P) Unplanned operations (code U) Non- productive time (NPT) Planned and unplanned operations are part of regular operations, while NPT is a downtime. No revenues on downtime! [65-71] Well Construction Contracts The two actors in a contract are the operator (company) and the contractors. The operator can drill the well (company) and owns the assets, the contractors provide specialised and ancillary services involved with operations. Contracts are awarded at the end of a Tendering Process. Once the well program is ready, the procurement department proceeds to purchase materials, and to award the contracts with the required contractors. Contracts can be Time based: costs are affected by time Price per unit (fixed costs) Talking of a well, e.g. 16% fixed costs and 84% time based. Intangibles are 43%. For exploration we are around 500K-1M$ per day. Onshore is less costly than offshore [73-] Technology innovation R&D in Well operations is divided in 4 section Operational efficiency Safety enhancement Energy transition o Carbon Capture and Storage (CCS) o Geothermal Energy o Undergroung Hydrogen Storage (UHS) o GHG Emission Monitoring Digital solutions Eni has developed multiple technologies to improve safety and performance in years Drilling safety package : casing valves, new wellhead safety valve, downhole isolation packer to prevent risks (process safety) E-WISE (Eni Well Incident Systematic Evaluation) : is an advanced risk mgmt model for blowout probability calculation for a specific well design. The probability is calculated based on three criterion: human error, mechanical failure and geological conditions. Contents Carbon capture and storage: for integration see 240611 (p.2) - CCS (4.2) o Introduction o R&D projects o CCS projects - innovation highlights Geothermal energy o Introduction o Incubation project o VALE project Hydrogen o Introduction o R&D project GHG monitoring Digital solutions - Digital Drilling Packaeg [8-] CCS - Introduction CCS: Capture is performed with amine-based liquids. CO2 is made available at high purity (95%). Injection happens in gas, liquid or supercritical state. The three main elements of a geological storage are: a reservoir rock for CO2 injection a cap rock above, and a trap system avoiding lateral movement of fluid in the reservoir. Two are the main types of sites: saline aquifers and depleted reservoirs. When developing CCS technology, main aspect and criticalities to address are: [12-15] Provide a rock-to-rock plug, meaning a cement plug in the tube that is not permeable to CO2 and isolate CO2 from channeling up. Barrier Solutions are: minimum 200 feet of good cement adiacent to the annular good cement of the tube casing, or dual cement barrier solution each 100 feet CO2 has low critical pressure (74 bar) and temperature (31°C). When CO2 is in supercritical state, its expansion causes a severe cooling effect that can lead to very low temperature, causing severe problems to the materials. When injecting CO2, temperature could drop to -78°C. Dry ice and hydrate forms around the expansion region CO2 can change significantly its density passing from gas (low density) to liquid or supercritical state (high density), causing erosion problems In presence of water, CO2 reacts generating acids. Acids can pose corrosion or cement carbonation problems Materials should be deigned for low T and corrosive environment. [16-25] CCS - R&D projects A CO2 injection well present the same fundamental elements as O&G well R&D projects focus on: Assessments of commercial CO2 resistant cements. Cement should not carbonate with CO2 and should resist re-pressurization cycles Tubing freaded (avvitate) connections should resist low T (-35°C to - 78°C), because JT effect can cause severe T drop. Mechanical stress tests and simulation are performed @ low T. [21-23] SC-SSV (Subsurface Safety Valve test) [26-31] CCS projects - innovation highlights Highlights Bismuth alloy for Rock-to-Rock plug are used in Hewett Field Specific projects ongoing to address innovative solutions for P&A in Ravenna district (old well field) [32-35] Geothermal - Intro Geothermal energy is the thermal energy generated and stored in the Earth from radioactive decay and continual heat loss. Geothermal energy contributes to global renewable capacity amounts to 16 GW. A geothermal system is made up of: a heat source, a reservoir (acquifer or hot rocks), production and injection wells, and conversion plants. Conventional geothermic resources are hydrothermal from volcanic or sedimentary reservoirs, while Unconventional geothermal system includes enhanced geothermal system (EGS) and advanced geothermal system (AGS) Geothermal Energy can be in form of: heat from low temperature reservoir or electricity from medium high temperature reservoir Three entalpy levels: vapour dominated streams, so vapour at high temperature above 240°C. It can be used for power generation in flash and dry steam geothermal power plants. Liquid dominated or flash streams, medium temperature between 110- 240°C. Suitable for power conversion via ORC or Power Production above 1 MW How water streams (70-110°C). Not suitable for power generation, use for direct use or district heating [37-43] Geothermal - Incubation project The objective of the project is addressing main technical needs and gaps in geothermal wells. Main issues to address in geothermal fields: The choice of drill beats suitable for application in volcanic high temperature wells. Formations are characterised by high strengths and abrasion properties Electronic components (Measurement While Drilling MWD, Logging While Drilling LWD), that are fundamental for drilling operation and monitoring withstand 175°C maximum. Directional Drilling DD limited by high T (150-180°C). A solution may involve cooling system for high temperature downhole (HTDH) Muds and cement are tested up to 250°C, but in literature there are field applications up to 300°C. With increasing temperature muds become thinner and more unstable; oil-based muds can withstand 180-200°C and mud coolers can be used to lower T about 30-40°C. At high T, cementing reaction become faster, reducing thickening time and making cementing more challenging Well completion is not always present. Bigger casings are commonly adopted to enhance hot fluid flow rate. Expansion spool to compensate for thermal elongation Material selection is important, to resist high temperature effects, fatigue degradation and corrosion. [44-47] Geothermal - VALE project The objective of the project is producing a proprietary workflow for techno- economic evaluation (screening) of high entropy geothermal fields (150-350°C): Finding areas with the greatest energy potential Evaluation of recoverable heat Well design Defining testing protocol for geothermal application [48-51] Hydrogen - Intro Hydrogen is important because it can store and supply large quantities of energy. Three types of hydrogen Grey hydrogen from steam reforming Blue hydrogen from steam reforming and carbon capture Green hydrogen from renewable energy H2 storage becomes competitives for long cycles: for short charge/discharge cycles, batteries are more efficient. The three main elements of a geological storage are: a reservoir rock for H2 injection a cap rock above, and a trap system avoiding lateral movement of fluid in the reservoir. Three are the main types of sites: Depleted reservoirs (from 100s metres to 2700 m depth, pressure from 10s to 285 bar) Take advantage of existing facilities, highest depth available. Large volume available. The main issues are related to the controllability: possible H2 losses, interaction with bacteria and chemical reactions -> it is difficult to get back pure H2 Salt dome (from 100s metres to 1800 m depth, pressure from 10s to 210 bar). Lowest depth, lowest pressure available. from a structural and functional point of view they are the best: impervious walls, easy charge and discharge, flexible and modular. The main disadvantages are restricted volume, leakages and impurities that may trigger reactions. Aquifer (from 100s metres to 2300 m depth, pressure from 10s to 315 bar). The aim is assessing H2 impact on well equipment: metals, elastomers, cement [52-59] Hydrogen - R&D projects Evaluating the effect of hydrogen on metals including: hydrogen embrittlement & stress cracking (hydrogen + applied stress), induced cracking (stress induced by hydrogen), chemical reactions (decarburization forming methane or hydrides) and hydrogen attack at high temperature (decarburization at high T) Long-term testing in high-pressure environment, electrochemical loading test (faster and easier to be performed) Metal tests on J55, L80, T95, P110 Effect of hydrogen on elastomers including: volume change, plasticization effect (hydrogen diffusion), explosive decompression failure (XDF) for rapid gas decompression (RGD) Elastomer tests on nitril rubber (NBR), hydrogenated nytril rubber (HNBR), Flourine Kautschuk Material (FKM) Evaluating effect of hydrogen on cement: potential reduction of Fe2O3 to Fe3O4 should not impact cement resistance and sealing capacity. No significant changes were recorded in cement performance after H2 exposure. Non-reactive behaviour was demonstrated. [60-70] GHG monitoring Scope 1, 2 and 3, ENI objectives (see 240618 (m.3) - Carbon neutrality in UPS (4.5)) GHG emission of rig fall into Scope 3, need to be quantified and monitored with dedicated tools API compendium identifies all upstream segments with emission sources for each. For D&C the main CO2 emission source is the fuel gas of power engine. Challenge is integrate CO2 accounting method inside performance monitoring tools. The digital workflow accounting cycle for greenhouse gas emissions consists in integrating the classical standard methodology for estimation, based on multiplying fuel consumption by emission factor (considering GWP index), inside the monitoring system in order to see emissions by activity and identify reduction solution. Advanced analytics provides insights and GHG allocation along well construction process to select the most suitable solutions for emission reduction. GHG emissions vs land rig (standard = 1,0) Jack-up: between 1 to 2.5 times more Semisub (anchored): 2 to 3 Semisub (DP): 4 to 8.5 Drilling ship: 5 to 8. The dynamic position rigs have more consumption because energy is required to keep them in place. [71-89] Digital solutions - Digital Drilling Package Objective is building a great data platform Digital Drilling Package based on three instruments Id3: Integrated drilling data discovery. It is a web-based software that allows to perform a detailed rig performance analysis through the calculation of standardised parameters KPI: identification of Invisible Lost Time, well planning, real-time operations monitoring, post-well analysis. Combines rig data (standard surface logging) with daily reports through logical rules to evaluate accurate performance index. Target values (benchmark) for performances monitoring are automatically generated. E-npt: NPT predictive tool. Predictive algorithm based on early detection of precursor events. eDrilling: well simulator, drilling digital twin. It allows to perform dynamic simulation for accurate web planning. Contents: LNG value chain and chain efficiency Main properties and definitions Plant and components Shipping and regassification Innovation on floaters and small scale LNG [6-13] LNG value chain and chain efficiency 4 main step in LNG value chain: Upstream Liquefaction (upstream and liquefaction are the 80% of total investment in the chain) Shipping, and Regassification Upstream involves high risk and margins, while liquefaction shipping and regassification have variable risks and lower margins The chain efficiency is defined as the ratio between the energy sold and the energy from the wells. Typical efficiency is between 85 and 90%. The losses of efficiency are related to fueling turbogas for liquefaction (nearly 10-11%) Losses during transport (0,16%/gg) losses during regassification (1-2%) [14-15] Main properties, composition Gas has to be taken to -161°C for liquefaction, that is the temperature for methane liquefaction and methane is the main component (>85%). It is stored and transported at atm pressure. The density as liquid is 1/600 vs gas, 1/2 vs water as liquid Units Metric tonnes for weight Scm or scft for volume MMBtu for calorific power Composition: Methane CH4 70-90 mol% Ethane C2 Propane, Butane (C3-4) or LPG C5+ as condensate Acid gases (CO2, H2S) as impurities Nitrogen as impurity LNG or grid includes C1 to C4 NGLs includes C2-C5+ [16-31] Plant and components A typical LNG plant involves 1. Upstream section. In the upstream section, the feed gas is supplied from the wells. The gas is received and metered, then sent to the slug catcher and MEG section for dehydration. The condensate and hydrate are stabilised and then used for reinjection, while the dehydrated gas is sent to the warm end pretreatment. 2. Warm end section (pretreatment). The warm end section includes the acid gas removal and following acid gas treatment. Once the gas has been purified from acid gas, it is sent to a new dehydration unit and then mercury removal. The gas is then ready for cold end section. 3. Cold end section (cooling & liquefaction, fractionation). First, the hydrocarbons are separated. LNG are sent to liquefaction. Fractionation helps separating heavy condensates (to reinjection or disposal) from LPG, that are recycled to liquefaction. Liquefaction can be performed at different pressure level. The majority of processes maintain high pressure value for LNG (up to critical pressure or above, 45 bars) as long as possible by reducing pressure only at the end. Refrigeration of LNG is performed in heat exchangers, using as coolant different types of compounds in a closed cycle (same as refrigerator: coolant itself heating up to cool down LNG, then expansion, cool down, compression and again). Steam or gasturbine are used to drive the compressor. Propane is tipically used for pre-cooling, then mixed refrigerant (MR, mix of HC as methane, ethane, propane) for intermediate cycle, then nitrogen cycle for final stages (only in some cases, AP-X). Lot of patents are available for liquefaction technology. The main processes are propane pre-cooled mixed refrigerant (C3MR) - APCI For small-medium trains, 1 to 6 mtpa ConocoPhilips Optimized Cascade Process (COPOC), Double Mixing Refrigerant (DMR) - Shell propane pre-cooled mixed refrigerant and nitrogen (AP-X) - APCI For bigger trains, up to 8 mtpa Multi fluid cascade process (MFC) - Linde APCI are the most used (84%), then COPOC (11%), then others. 4. Off-load section. After the gas has been liquefied, it is stored and then loaded to ships. [1-12] Shipping Capacity: 100 to 270.000 m3. Mainly 150.000-185.000 m3 (large LNG), approx 200-300M$ Size classification: o QMAX 270.000 m3 o QFLEX 210.000 m3 o Large LNG o RLNG (regasification vessel) o Midsize 30.000-90.000 m3 o Small scale