Bacterial Gene Expression Regulation PDF
Document Details
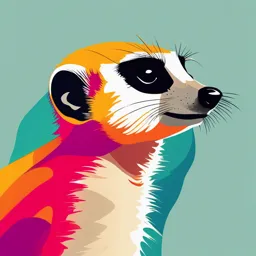
Uploaded by BrightestDune
Rutgers University
Tags
Related
Summary
This document provides an overview of the regulation of bacterial gene expression. It explores the concepts of constitutive genes, inducible genes, repressible genes, and catabolite repression. The document further discusses pre-transcriptional control including repression and induction and introduces the operon model, highlighting the roles of the promoter, operator, and structural genes. It also touches on epigenetic control mechanisms. Finally, there is a summary of mutations, mutagen agents, and repair mechanisms.
Full Transcript
The Regulation of Bacterial Gene Expression Constitutive genes are expressed at a fixed rate Other genes are expressed only as needed Inducible genes Repressible genes Catabolite repression Pre-Transcriptional Control Repression inhibits gene expression and decreases...
The Regulation of Bacterial Gene Expression Constitutive genes are expressed at a fixed rate Other genes are expressed only as needed Inducible genes Repressible genes Catabolite repression Pre-Transcriptional Control Repression inhibits gene expression and decreases enzyme synthesis – Mediated by repressors, proteins that block transcription – Default position of a repressible gene is on Induction turns on gene expression – Initiated by an inducer – Default position of an inducible gene is off The Operon Model of Gene Expression Promoter: segment of DNA where RNA polymerase initiates transcription of structural genes Operator: segment of DNA that controls transcription of structural genes Operon: set of operator and promoter sites and the structural genes they control Copyright © 2019 Pearson Education, Inc. All Rights Reserved The Operon Model of Gene Expression In an inducible operon, structural genes are not transcribed unless an inducer is present Example: the lac operon of E. coli – Three enzymes encoded by the lac operon are needed to metabolize lactose ▪ The structural genes for these enzymes (Z, Y, and A) are adjacent on the chromosome and their transcription is regulated together ▪ Control region includes: – Promoter: where transcription begins – Operator: stop or go signal Copyright © 2019 Pearson Education, Inc. All Rights Reserved The Operon Model of Gene Expression In the absence of lactose, the repressor (product of the I gene) binds to the operator, preventing transcription In the presence of lactose, a metabolite of lactose— allolactose (inducer)—binds to the repressor; the repressor cannot bind to the operator and transcription occurs Copyright © 2019 Pearson Education, Inc. All Rights Reserved Figure 8.12 An Inducible Operon (1 of 3) Operon Control region Structural genes I P O Z Y A DNA Regulatory Promoter Operator gene Structure of the operon. The operon consists of the promoter (P) and operator (O) sites and structural genes that code for the protein. The operon is regulated by the product of the regulatory gene (I) Copyright © 2019 Pearson Education, Inc. All Rights Reserved Figure 8.12 An Inducible Operon (2 of 3) RNA polymerase I P Z Y A Transcription Repressor Active mRNA repressor protein Translation Repressor active, operon off. The repressor protein binds with the operator, preventing transcription from the operon. Copyright © 2019 Pearson Education, Inc. All Rights Reserved Figure 8.12 An Inducible Operon (3 of 3) I P O Z Y A Transcription Operon mRNA Translation Allolactose (inducer) Inactive Transacetylase repressor Permease protein β-Galactosidase Repressor inactive, operon on. When the inducer allolactose binds to the repressor protein, the inactivated repressor can no longer block transcription. The structural genes are transcribed, ultimately resulting in the production of the enzymes needed for lactose catabolism. Copyright © 2019 Pearson Education, Inc. All Rights Reserved Positive Regulation Catabolite repression inhibits cells from using carbon sources other than glucose Cyclic AMP (cAMP) builds up in a cell when glucose is not available cAMP binds to the catabolic activator protein (CAP) that in turn binds the lac promoter, initiating transcription and allowing the cell to use lactose Copyright © 2019 Pearson Education, Inc. All Rights Reserved Bacteria growing Figure 8.14 The Growth on Rate of E. Coli on Glucose glucose as the sole and Lactose carbon Glucose source grow faster than on Lactose lactose. Bacteria growing All glucose in a consumed medium containing glucose and lactose first Glucose Lactose usedconsume used Lag the glucose and then, time after a short lag time, the lactose. During the lag time, intra- cellular cAMP increases, Copyright © 2019 the lac Pearson Education, Inc. All Rights Reserved Promoter Figure 8.15 Positive lacl lacZ Regulation of the Lac DNA Operon CAP-binding site RNA Operator polymerase can bind and transcribe cAMP Active CAP Inactive lac repressor Inactive CAP Lactose present, glucose scarce (cAMP level high). If glucose is scarce, the high level of cAMP activates CAP, and the lac operon produces large amounts of mRNA for lactose digestion. Promoter lacl lacZ DNA Operator RNA CAP-binding site polymerase can't bind Inactive lac Inactive repressor CAP Lactose present, glucose present (cAMP level low). When glucose is present, cAMP is scarce, and CAP is unable to stimulate transcription. Copyright © 2019 Pearson Education, Inc. All Rights Reserved The Operon Model of Gene Expression (3 of 3) In repressible operons, structural genes are transcribed until they are turned off – Excess tryptophan is a corepressor that binds and activates the repressor to bind to the operator, stopping tryptophan synthesis Copyright © 2019 Pearson Education, Inc. All Rights Reserved Figure 8.13 A Repressible Operon (1 of 3) Operon Control region Structural genes I P O E D C B A DNA Regulatory Promoter Operator gene Structure of the operon. The operon consists of the promoter (P) and operator (O) sites and structural genes that code for the protein. The operon is regulated by the product of the regulatory gene (I) Copyright © 2019 Pearson Education, Inc. All Rights Reserved Figure 8.13 A Repressible Operon (2 of 3) RNA polymerase I P O E D C B A Transcription Repressor mRNA Operon mRNA Translation Polypeptides Inactive comprising the repressor enzymes for protein tryptophan synthesis Repressor inactive, operon on. The repressor is inactive, and transcription and translation proceed, leading to the synthesis of tryptophan. Copyright © 2019 Pearson Education, Inc. All Rights Reserved Figure 8.13 A Repressible Operon (3 of 3) P I E D C B A Active repressor protein Tryptophan (corepressor) Repressor active, operon off. When the corepressor tryptophan binds to the repressor protein, the activated repressor binds with the operator, preventing transcription from the operon. Copyright © 2019 Pearson Education, Inc. All Rights Reserved Epigenetic Control Methylating nucleotides turn genes off Methylated (off) genes can be passed to offspring cells Not permanent Copyright © 2019 Pearson Education, Inc. All Rights Reserved Changes in Genetic Material Mutation: a permanent change in the base sequence of DNA Mutations may be neutral, beneficial, or harmful Mutagens: agents that cause mutations Spontaneous mutations: occur in the absence of a mutagen Copyright © 2019 Pearson Education, Inc. All Rights Reserved Parental DNA Figure 8.17 Base Substitutions Replication During DNA replication, a thymine is incorporated opposite guanine by Daughter DNA mistake. Daughter DNA Daughter DNA If not corrected, in the next round of replication, adenine pairs with Replication the new thymine, yielding an AT pair in place of the original GC pair. Granddaughter DNA When mRNA is transcribed from Transcription the DNA containing this substitution, a codon is produced that, during translation, encodes a different mRNA amino acid: tyrosine instead of cysteine. Translation Amino acids Cysteine Tyrosine Cysteine Cysteine Copyright © 2019 Pearson Education, Inc. All Rights Reserved Figure 8.18a-b Types of Mutations and Their Effects on the Amino Acid Sequences of Proteins DNA (template strand) Transcription mRNA Translation Amino acid sequence Met Lys Phe Gly Stop Normal DNA molecule DNA (template strand) mRNA Amino acid sequence Met Lys Phe Ser Stop Missense mutation Stop Copyright © 2019 Pearson Education, Inc. All Rights Reserved Figure 8-18a–c Types of Mutations and Their Effects on the Amino Acid Sequences of Proteins For Long description, see slide 183: Appendix 34 Copyright © 2019 Pearson Education, Inc. All Rights Reserved Types of Mutations Frameshift mutation – Insertion or deletion of one or more nucleotide pairs – Shifts the translational “reading frame” – Causes changes in many amino acids downstream from the site of the original mutation Copyright © 2019 Pearson Education, Inc. All Rights Reserved Figure 8.18a-d Types of Mutations and Their Effects on the Amino Acid Sequences of Proteins DNA (template strand) Transcription mRNA Translation Met Lys Leu Ala Amino acid sequence Met Lys Phe Gly Stop Normal DNA molecule Frameshift mutation Copyright © 2019 Pearson Education, Inc. All Rights Reserved Chemical Mutagens Nitrous acid: causes adenine to bind with cytosine instead of thymine Nucleoside analog: incorporates into DNA in place of a normal base; causes mistakes in base pairing Copyright © 2019 Pearson Education, Inc. All Rights Reserved Figure 8.19a Oxidation of Nucleotides Makes a Mutagen Copyright © 2019 Pearson Education, Inc. All Rights Reserved Figure 8.19b Oxidation of Nucleotides Makes a Mutagen Copyright © 2019 Pearson Education, Inc. All Rights Reserved Figure 8.20 Nucleoside Analogs and the Nitrogenous Bases They Replace Copyright © 2019 Pearson Education, Inc. All Rights Reserved Radiation Ionizing radiation (X-rays and gamma rays) causes the formation of ions that can oxidize nucleotides and break the deoxyribose-phosphate backbone UV radiation causes thymine dimers Copyright © 2019 Pearson Education, Inc. All Rights Reserved Radiation Photolyases separate thymine dimers Nucleotide excision repair: Enzymes cut out incorrect bases and fill in correct bases Copyright © 2019 Pearson Education, Inc. All Rights Reserved Ultraviolet light Figure 8.21 The Creation and Repair of a Thymine Dimer Caused by Exposure to ultraviolet light causes adjacent thymines to Ultraviolet Light become cross-linked, forming a thymine dimer and disrupting Thymine dimer their normal base pairing. An endonuclease cuts the DNA, and an exonuclease removes the damaged DNA. New DNA DNA polymerase fills the gap by synthesizing new DNA, using the intact strand as a template. DNA ligase seals the remaining gap by joining the old and new DNA. Copyright © 2019 Pearson Education, Inc. All Rights Reserved Identifying Mutants Positive (direct) selection detects mutant cells because they grow or appear different than unmutated cells Negative (indirect) selection detects mutant cells that cannot grow or perform a certain function Auxtotroph: mutant that has a nutritional requirement absent in the parent – Use of replica plating Copyright © 2019 Pearson Education, Inc. All Rights Reserved Figure 8.22 Replica Plating Handle Sterile velvet is pressed on the grown Velvet colonies on the surface master plate. (sterilized) Master plate with medium Cells from each colony containing are transferred from the histidine velvet to new plates. Petri plate with medium containing Petri plate with histidine medium lacking Plates are incubated. histidine Auxotrophic mutant Colony missing Growth on plates is compared. A colony that grows on the medium with histidine but could not grow on the medium without histidine is auxotrophic (histidine-requiring mutant). Copyright © 2019 Pearson Education, Inc. All Rights Reserved Identifying Chemical Carcinogens Many mutagens are carcinogens (cause cancer) Mutagens can be tested, using bacteria as carcinogen indicators The Ames test exposes mutant bacteria to mutagenic substances to measure the rate of reversal of the mutation – Uses a histidine (his-) auxotroph of Salmonella and measures its reversion to his+ after mutagen exposure – Rat liver extract is added to provide activation enzymes since animal enzymes may facilitate mutagenic activity – Reversion rate indicates degree to which a substance is mutagenic Copyright © 2019 Pearson Education, Inc. All Rights Reserved Figure 8.23 The Ames Reverse Gene Mutation Test Experimental sample Suspected mutagen Rat liver extract Experimental plate Incubation Cultures of Colonies of histidine-dependent Media lacking histidine revertant Salmonella bacteria Control Rat liver (no suspected extract mutagen added) Incubation Control plate Two cultures are pre- The suspected Each sample is poured onto The numbers of colonies on the experimental pared of Salmonella mutagen is added to a plate of medium lacking and control plates are compared. The control bacteria that have lost the experimental histidine. The plates are plate may show a few spontaneous the ability to synthesize sample only; rat liver then incubated at 37C for two histidine-synthesizing revertants. The test histidine (histidine- extract (an activator) days. Only bacteria whose plates will show an increase in the number of dependent). is added to both histidine-dependent histidine-synthesizing revertants if the test samples. phenotype has mutated back chemical is indeed a mutagen and potential (reverted) to histidine- carcinogen. The higher the concentration of synthesizing will grow into mutagen used, the more revertant colonies colonies. will result. Copyright © 2019 Pearson Education, Inc. All Rights Reserved