Lehninger Principles of Biochemistry Fatty Acid Catabolism PDF
Document Details
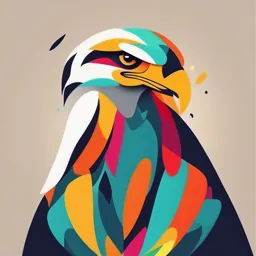
Uploaded by TrustworthyAgate6970
2021
David L. Nelson • Michael M. Cox
Tags
Summary
This document provides lecture notes on fatty acid catabolism, encompassing principles, mechanisms, and regulatory aspects. It outlines processes such as beta-oxidation and the role of various enzymes in these pathways. The document includes activities and questions to test knowledge of the studied concepts.
Full Transcript
17 Fatty Acid Catabolism © 2021 Macmillan Learning The Oxidation of Long-Chain Fatty Acids to Acetyl-CoA serves as a central energy-yielding pathway in many organisms and tissues – provides as much as 80% of the energetic needs in mammalian heart and liver – provides >4...
17 Fatty Acid Catabolism © 2021 Macmillan Learning The Oxidation of Long-Chain Fatty Acids to Acetyl-CoA serves as a central energy-yielding pathway in many organisms and tissues – provides as much as 80% of the energetic needs in mammalian heart and liver – provides >40% of the daily energy requirement electrons removed from fatty acids during oxidation pass through the respiratory chain, driving ATP synthesis acetyl-CoA produced from the fatty acids may be completely oxidized to CO2 in the citric acid cycle β Oxidation β oxidation = oxidation of the fatty acyl group at the C-3, or β, position—hence the name – occurs after the carboxyl group at C-1 is activated by attachment to coenzyme A Principle 1 (1 of 5) Metabolites of diverse origin funnel into a few central pathways. Fatty acid catabolism and glycolysis convert quite different starting materials into the same product (acetyl-CoA). The electrons from the oxidative reactions of these pathways and of the citric acid cycle are carried by common cofactors (NAD and FAD) to the mitochondrial respiratory chain leading to oxygen, providing the energy for ATP synthesis by oxidative phosphorylation. Principle 2 (1 of 5) Evolution selects for chemical mechanisms that make useful reactions more energetically favorable, and those same mechanisms are used in different pathways. In the breakdown of fatty acids, we see the activation of a carboxylic acid by its conversion to a thioester, as we saw with acetyl-CoA in the citric acid cycle. To break the C–C bonds in the long chain of relatively inert –CH2–CH2– groups in fatty acids, a carbonyl group is created adjacent to the – CH2– group, as we saw in the reactions of the citric acid cycle. Principle 3 (1 of 4) Allosteric mechanisms and posttranslational regulation (protein phosphorylation) coordinate metabolic processes within a cell. Hormones and growth factors coordinate metabolic activities among tissues and organs. Reciprocal regulation of catabolic and anabolic pathways prevents the inefficiency of futile cycling. Principle 4 (1 of 6) When a process lacks a critical component—an enzyme, a cofactor, or a regulatory agent—the resulting loss of homeostasis may cause disease across a spectrum of severity. Defects in fatty acid breakdown are no exception. Principle 5 (1 of 4) The liver plays a unique role in whole-body metabolism. When glucose is unavailable, the liver makes glucose by gluconeogenesis and releases it to the blood for distribution to other tissues, including the brain. During starvation, the liver processes fatty acids into ketone bodies, which, unlike fatty acids, can cross the blood brain barrier and fuel the brain. 17.1 Digestion, Mobilization, and Transport of Fats Sources of Fatty Acid Fuels cells can obtain fatty acid fuels from four sources: – fats consumed in the diet – fats stored in cells as lipid droplets – fats synthesized in one organ for export to another – fats obtained by autophagy Clicker Question 1 Which answer choice is NOT a major source of fatty acid fuels in vertebrates? A. conversion in the liver of excess dietary amino acids to fats B. conversion in the liver of excess dietary carbohydrates to fats C. fats stored in adipocytes D. fatty acids in the diet Clicker Question 1, Response Which answer choice is NOT a major source of fatty acid fuels in vertebrates? A. conversion in the liver of excess dietary amino acids to fats Although vertebrates do have pathways to convert amino acids to fats, this is not a significant source of fatty acid fuels. Dietary Fats Are Absorbed in the Small Intestine Chylomicron Formation apolipoproteins = proteins in their lipid-free form that bind lipids to form lipoproteins – target triacylglycerols, phospholipids, cholesterol, and cholesteryl esters for transport between organs chylomicrons = particles consisting of triacylglycerols, cholesterol, and apolipoproteins Lipoprotein Particles lipoprotein particles = spherical aggregates of apolipoproteins and lipids – arranged with hydrophobic lipids at the core and hydrophilic protein side chains and lipid head groups at the surface – various in densities depending on combinations of lipid and protein range from chylomicrons and very-low-density lipoproteins (VLDL) to very-high-density lipoproteins (VHDL) Apolipoprotein B-48 (apoB-48) and Apolipoprotein C-II (apoC-II) apolipoprotein B-48 (apoB-48) = primary protein component of chylomicrons apolipoprotein C-II (apoC-II) = protein picked up in the blood by chylomicrons from high-density lipoprotein (HDL) particles Lipoprotein Lipase lipoprotein lipase = extracellular enzyme in the capillaries of muscle and adipose tissue that hydrolyzes triacylglycerols to free fatty acids and monoacylglycerols – activated by apoC-II Clicker Question 2 What does apolipoprotein C-II do? A. It activates LCAT on HDL. B. It is a structural component of LDL. C. It is a ligand for the LDL receptor. D. It activates lipoprotein lipase activity on chylomicrons. E. It inhibits lipoprotein lipase activity on chylomicrons. Clicker Question 2, Response What does apolipoprotein C-II do? D. It activates lipoprotein lipase activity on chylomicrons. In the blood, chylomicrons pick up apolipoprotein C-II (apoC-II) from high-density lipoprotein (HDL) particles and are carried to muscle and adipose tissue. In the capillaries of these tissues, the extracellular enzyme lipoprotein lipase, activated by apoC-II, hydrolyzes triacylglycerols to free fatty acids and monoacylglycerols. Clicker Question 3 Which statement is false regarding the processing of dietary lipids in vertebrates? A. Dietary lipids are emulsified by bile salts in the intestine. B. Triacylglycerols in mixed micelles in the intestine diffuse into cells of the intestinal mucosa. C. Ultimately, dietary lipids are oxidized as fuel by muscle or stored as triacylglycerols in adipose tissue. D. Dietary lipids are packaged in lipoprotein aggregates known as chylomicrons, which are then exported to the lymph system. Clicker Question 3, Response Which statement is false regarding the processing of dietary lipids in vertebrates? D. Triacylglycerols in mixed micelles in the intestine diffuse into cells of the intestinal mucosa. Water-soluble lipases in the intestine convert triacylglycerols to monoacylglycerols, diacylglycerols, and free fatty acids. These products of lipase action diffuse or are transported into the epithelial cells lining the intestinal surface (the intestinal mucosa), where they are reconverted to triacylglycerols. Principle 3 (2 of 4) Allosteric mechanisms and posttranslational regulation (protein phosphorylation) coordinate metabolic processes within a cell. Hormones and growth factors coordinate metabolic activities among tissues and organs. Reciprocal regulation of catabolic and anabolic pathways prevents the inefficiency of futile cycling. Storage of Excess Fatty Acids fatty acids are converted to triacylglycerols in the liver triacylglycerols are packaged with specific apolipoproteins into VLDLs VLDLs are secreted and transported in the blood to adipose tissue triacylglycerols are removed and stored in lipid droplets within adipocytes in the adipose tissue Hormones Trigger Mobilization of Stored Triacylglycerols lipid droplets = organelles stored in adipocytes and steroid- synthesizing cells that contain neutral lipids – contain a core of triacylglycerols and sterol esters surrounded by a monolayer of phospholipids perilipins = family of proteins that coats the surface of lipid droplets to restrict access to lipid droplets – prevent untimely lipid mobilization Clicker Question 4 What are perilipins? A. major proteins of periwinkles B. apolipoproteins of chylomicrons C. coat proteins of lipid droplets D. cytosolic lipidated proteins E. enzymes with cholesteryl esterase activity Clicker Question 4, Response What are perilipins? C. coat proteins of lipid droplets Phosphorylated perilipins expose the lipids stored in a lipid droplet to the hormone-sensitive lipase (HSL). Principle 1 (2 of 5) Metabolites of diverse origin funnel into a few central pathways. Fatty acid catabolism and glycolysis convert quite different starting materials into the same product (acetyl-CoA). The electrons from the oxidative reactions of these pathways and of the citric acid cycle are carried by common cofactors (NAD and FAD) to the mitochondrial respiratory chain leading to oxygen, providing the energy for ATP synthesis by oxidative phosphorylation. Mobilization of Triacylglycerols Stored in Adipose Tissue mobilization occurs when hormones (glucagon and epinepherine) signal the need for metabolic energy PKA triggers changes that open the lipid droplet to the action of three cytosolic lipases Clicker Question 5 Which factor would stimulate movement of fatty acids to muscle and the liver when blood glucose levels fall? A. insulin B. glucagon C. an increase in protein kinase C in activity D. an increase in phospholipase C activity E. an increase in citric acid cycle activity in adipose Clicker Question 5, Response Which factor would stimulate movement of fatty acids to muscle and the liver when blood glucose levels fall? B. glucagon Low levels of glucose in the blood trigger the release of glucagon. Binding of this hormone to a G protein–coupled receptor on the adipocyte plasma membrane triggers the mobilization of stored triacylglycerol. Human Serum Albumin free fatty acids, FFAs = fatty acids released by lipases serum albumin = blood protein that noncovalently binds and transports FFAs to target tissues – makes up ~½ of the total serum protein Entry of Glycerol into the Glycolytic Pathway most of the biologically available energy of triacylglycerols resides in their three long-chain fatty acids glycerol kinase = phosphorylates glycerol to form glycerol 3- phosphate glyceraldehyde 3-phosphate can enter glycolysis Clicker Question 6 Which molecule can be produced rapidly from glycerol in only three steps, allowing an interaction between carbohydrate and lipid metabolism? A. acetyl-CoA B. glucose C. pyruvate D. glyceraldehyde 3-phosphate E. phosphoenolpyruvate Clicker Question 6, Response Which molecule can be produced rapidly from glycerol in only three steps, allowing an interaction between carbohydrate and lipid metabolism? D. glyceraldehyde 3-phosphate The glycerol released by lipase action is phosphorylated by glycerol kinase, and the resulting glycerol 3-phosphate is oxidized to dihydroxyacetone phosphate. The glycolytic enzyme triose phosphate isomerase converts this compound to glyceraldehyde 3-phosphate, which is oxidized via glycolysis. Fatty Acids Are Activated and Transported into Mitochondria small (< 12 carbons) fatty acids diffuse freely across mitochondrial membranes carnitine shuttle = transports long-chain fatty acids (containing 14+ carbons) through the mitochondrial membrane – requires activation to a fatty acyl-CoA and attachment to carnitine Clicker Question 7 Where does β oxidation occur? A. in the cytosol B. in the mitochondrial matrix C. in the ER lumen D. on the Golgi apparatus membrane E. at the plasma membrane Clicker Question 7, Response Where does β oxidation occur? B. in the mitochondrial matrix The enzymes of fatty acid oxidation in animal cells are located in the mitochondrial matrix. Clicker Question 8 Why does β oxidation occur in the mitochondrial matrix? A. to allow coordinated regulation with fatty acid synthesis B. to coordinate production of acetyl-CoA with the introduction into the citric acid cycle C. to compartmentalize D. because necessary oxidative enzymes are present E. All of the answers are correct. Clicker Question 8, Response Why does β oxidation occur in the mitochondrial matrix? E. All of the answers are correct. The mitochondrial matrix contains all the enzymes necessary for the β-oxidation pathway and the citric acid cycle. If β oxidation occurred in the cytosol, the simultaneous synthesis and degradation of fatty acids could occur, resulting in a wasteful and futile cycle. Fatty Acyl-CoA Synthetase fatty acyl-CoA synthetase = isozymes present in the outer mitochondrial membrane that activate the fatty acid by conversion to fatty acyl-CoA thioesters fatty acid + CoA + ATP ⇄ fatty acyl-CoA + AMP + PPi Principle 2 (2 of 5) Evolution selects for chemical mechanisms that make useful reactions more energetically favorable, and those same mechanisms are used in different pathways. In the breakdown of fatty acids, we see the activation of a carboxylic acid by its conversion to a thioester, as we saw with acetyl-CoA in the citric acid cycle. To break the C–C bonds in the long chain of relatively inert –CH2–CH2– groups in fatty acids, a carbonyl group is created adjacent to the – CH2– group, as we saw in the reactions of the citric acid cycle. Formation of a Fatty Acyl-CoA fatty acyl-CoA = contains a thioester linkage between the fatty acid carboxyl group and the thiol group of coenzyme A – high energy compound – formation is made more favorable by the hydrolysis of two ATP bonds The Overall Reaction for Fatty Acyl- CoA Formation involves three steps: – the two-step formation of the fatty acyl-CoA derivative – the hydrolysis of the pyrophosphate created the overall reaction is: fatty acid + CoA + ATP ⇌ fatty acyl-CoA + AMP + 2Pi (17-1) ∆G′° = −34 kJ/mol Clicker Question 9 Which reaction mechanism has sufficient total energy yield to add coenzyme A to fatty acids but requires two enzymes? A. phosphorylation B. oxidative decarboxylation C. dehydrogenation D. substrate-level phosphorylation E. adenylylation Clicker Question 9, Response Which reaction mechanism has sufficient total energy yield to add coenzyme A to fatty acids but requires two enzymes? E. adenylylation The reaction catalyzed by a fatty acyl–CoA synthetase involves a fatty acyl–adenylate intermediate. The formation of a fatty acyl–CoA is made more favorable by the hydrolysis of two high-energy bonds in ATP; the pyrophosphate formed in the activation reaction is immediately hydrolyzed by inorganic pyrophosphatase, which pulls the preceding activation reaction in the direction of fatty acyl–CoA formation. Carnitine carnitine = compound that transports fatty acyl-CoAs destined for mitochondrial oxidation across the inner mitochondrial membrane Attachment of Carnitine to the Fatty Acyl-CoA carnitine acyl-transferase 1, CAT1 (carnitine palmitoyltransferase 1, CPT1) = catalyzes a transesterification reaction to transiently attach a fatty acyl- CoA to the hydroxyl group of carnitine to form fatty acyl- carnitine The Acyl-Carnitine/Carnitine Cotransporter acyl-carnitine/carnitine cotransporter = allows the passive transport of the fatty acyl-carnitine ester – moves one carnitine into the intermembrane space as one fatty acyl-carnitine moves into the matrix Carnitine Acyltransferase 2 (CAT2) carnitine acyltransferase 2 (CAT2, CPT2) = transfers the fatty acyl group from carnitine back to coenzyme A to regenerate fatty acyl-CoA and free carnitine – located on the inner face of the inner mitochondrial membrane Clicker Question 10 How do fatty acids get into the mitochondrial matrix? A. spontaneously B. via the malate shuttle C. via carnitine palmitoyltransferase D. via palmitoyl-CoA transferase E. via the citrate shuttle Clicker Question 10, Response How do fatty acids get into the mitochondrial matrix? C. via carnitine palmitoyltransferase In a transesterification catalyzed by carnitine acyltransferase 1, CAT1 (also called carnitine palmitoyltransferase 1, CPT1) in the outer mitochondrial membrane, the fatty acyl–CoA is transiently attached to the hydroxyl group of carnitine to form fatty acyl– carnitine. The fatty acyl–carnitine ester then diffuses across the intermembrane space and enters the matrix by passive transport through the acyl-carnitine/carnitine cotransporter of the inner mitochondrial membrane. The Two Pools of Coenzyme A one pool is in the cytosol and the other is in mitochondria coenzyme A in the mitochondrial matrix is largely used in oxidative degradation of pyruvate, fatty acids, and some amino acids coenzyme A in the cytosol is used in the biosynthesis of fatty acids The Two Pools of Fatty Acyl-CoA one pool is in the cytosol and the other is in mitochondria fatty acyl-CoA in the mitochondrial matrix can be used for oxidation and ATP production – conversion to the carnitine ester commits it to oxidation fatty acyl–CoA in the cytosolic pool can be used for membrane lipid synthesis The Carnitine Shuttle Is a Major Control Point carnitine-mediated entry is the rate-limiting step for oxidation of fatty acids in mitochondria carnitine acyltransferase 1 is inhibited by malonyl-CoA, the first intermediate in fatty acid synthesis – prevents the simultaneous synthesis and degradation of fatty acids Clicker Question 11 The regulation of β oxidation: A. is not glucagon dependent in any cells in humans. B. is not directly linked to intermediates in fatty acid biosynthesis. C. occurs both by transcriptional regulation as well as by regulating transport of fatty acids into mitochondria. D. does not involve direct regulation of any of the four enzymes of β oxidation. Clicker Question 11, Response The regulation of β oxidation: C. occurs both by transcriptional regulation as well as by regulating transport of fatty acids into mitochondria. The first intermediate in fatty acid synthesis inhibits carnitine acyltransferase 1 (CAT1), assuring that fatty acid oxidation and fatty acid synthesis do not occur simultaneously. Two of the enzymes of oxidation are also regulated by metabolites that signal energy sufficiency. Additionally, transcriptional regulation can change the number of molecules of the enzymes of fatty acid oxidation on a longer time scale — minutes to hours. Clicker Question 12 The simultaneous activation of β oxidation and fatty acid synthesis in the same cells is a futile cycle. No biological work is accomplished. Which molecule prevents this futile cycle by preventing fatty acid transport through a membrane? A. acetyl-CoA B. malonyl-CoA C. palmitoyl-CoA D. ADP E. methylmalonyl-CoA Clicker Question 12, Response The simultaneous activation of β oxidation and fatty acid synthesis in the same cells is a futile cycle. No biological work is accomplished. Which molecule prevents this futile cycle by preventing fatty acid transport through a membrane? B. malonyl-CoA Malonyl-CoA, the first intermediate in fatty acid synthesis, inhibits carnitine acyltransferase 1 (CAT1), assuring that fatty acid oxidation and fatty acid synthesis do not occur simultaneously. 17.2 Oxidation of Fatty Acids Stage 1 of Fatty Acid Oxidation Stage 1: β oxidation – fatty acids undergo oxidative removal of successive two- carbon units in the form of acetyl-CoA Stage 2 of Fatty Acid Oxidation Stage 2: oxidation of acetyl-CoA groups to CO2 in the citric acid cycle – occurs in the mitochondrial matrix – generates NADH, FADH2, and one GTP Stage 3 of Fatty Acid Oxidation Stage 3: electron transfer chain and oxidative phosphorylation – generates ATP from NADH and FADH2 Clicker Question 13 An important theme in Biochemistry is interaction among metabolic pathways. Which pathway would obviously be MOST affected by increased β oxidation of fatty acids? A. glycolysis B. the citric acid cycle C. the glyoxylate pathway D. the pentose phosphate pathway E. gluconeogenesis Clicker Question 13, Response An important theme in Biochemistry is interaction among metabolic pathways. Which pathway would obviously be MOST affected by increased β oxidation of fatty acids? B. the citric acid cycle In the second stage of fatty acid oxidation, the acetyl groups of acetyl-CoA are oxidized to CO2 in the citric acid cycle, which also takes place in the mitochondrial matrix. Activity: Sources of Acetyl-CoA Observe the structure of acetyl-CoA in Mol3D viewer in your Achieve course. Activity Instructions (1 of 3) On your own, think of answers to the questions: – Which atoms of acetyl-CoA enter the citric acid cycle via the citrate synthase reaction? – What pathways produce acetyl-CoA? – What carbon sources can serve as starting materials for acetyl-CoA production? (2 minutes) Pair up to discuss your ideas. (2 minutes) Share your ideas with the class in group discussion. (3 minutes) Activity Solution (1 of 4) – Which atoms of acetyl-CoA enter the citric acid cycle via the citrate synthase reaction? As depicted, the two-carbon acetyl group on the right side of the molecule condenses with oxaloacetate during the citrate synthase reaction. – What pathways produce acetyl-CoA? fatty acid β oxidation, ketolysis, glycolysis (via the pyruvate dehydrogenase complex), amino acid breakdown Activity Solution (2 of 4) – What carbon sources can serve as starting materials for acetyl-CoA production? fatty acids, ketone bodies, amino acids*, carbohydrates (via pyruvate) *Note that the breakdown of ketogenic amino acids directly forms acetyl-CoA, whereas the breakdown of glucogenic amino acids indirectly forms acetyl‐CoA via intermediates such as pyruvate. The β Oxidation of Saturated Fatty Acids Has Four Basic Steps acyl-CoA dehydrogenase = flavoprotein with tightly bound FAD that catalyzes the dehydrogenation of fatty acyl-CoA to yield a trans-∆2- enoyl-CoA Acyl-CoA Dehydrogenase Isozymes isozymes are specific for fatty-acyl chain lengths: VLCAD (inner mitochondrial matrix): 12-18 carbons MCAD (matrix): 4-14 carbons SCAD (matrix): 4-8 carbons Electrons from Fatty Acyl-CoA Enter the Mitochondrial Respiratory Chain electron transfer flavoprotein (ETF) = electron carrier that accepts electrons from FADH2 ETF:ubiquinone oxidoreductase = flavoprotein that accepts electrons from ETF – passes electrons through ubiquinone into the mitochondrial respiratory chain Clicker Question 14 Which sequence of electron carriers transfers electrons from a fatty acyl–CoA to the mitochondrial respiratory chain? A. ETF → ubiquinone → ETF:ubiquinone oxidoreductase → FADH2 B. FADH2 → ETF → ETF:ubiquinone oxidoreductase → ubiquinone C. ETF → FADH2 → ETF:ubiquinone oxidoreductase → ubiquinone D. FADH2 → ETF:ubiquinone oxidoreductase → ubiquinone → ETF Clicker Question 14, Response Which sequence of electron carriers transfers electrons from a fatty acyl–CoA to the mitochondrial respiratory chain? B. FADH2 → ETF → ETF:ubiquinone oxidoreductase → ubiquinone Each molecule of FADH2 formed during oxidation of the fatty acyl–CoA donates a pair of electrons to the electron transfer flavoprotein (ETF). Electrons move from ETF to a second flavoprotein, ETF:ubiquinone oxidoreductase, and through ubiquinone into the mitochondrial respiratory chain. Principle 2 (3 of 5) Evolution selects for chemical mechanisms that make useful reactions more energetically favorable, and those same mechanisms are used in different pathways. In the breakdown of fatty acids, we see the activation of a carboxylic acid by its conversion to a thioester, as we saw with acetyl-CoA in the citric acid cycle. To break the C–C bonds in the long chain of relatively inert –CH2–CH2– groups in fatty acids, a carbonyl group is created adjacent to the – CH2– group, as we saw in the reactions of the citric acid cycle. The Oxidation Catalyzed by Acyl‐CoA Dehydrogenase Is Analogous to Succinate Dehydrogenation in both reactions: – the enzyme is bound to the inner membrane – a double bond is introduced into a carboxylic acid between the α and β carbons – FAD is the electron acceptor – electrons from the reaction ultimately enter the respiratory chain and pass to O2 Hydration of the Trans-∆2-Enoyl-CoA enoyl-CoA hydratase = catalyzes the addition of water to the double bond of the trans-∆2-enoyl- CoA to form L-β- hydroxyacyl-CoA (3- hydroxyacyl-CoA) – formally analogous to the fumarase reaction in the citric acid cycle Principle 2 (4 of 5) Evolution selects for chemical mechanisms that make useful reactions more energetically favorable, and those same mechanisms are used in different pathways. In the breakdown of fatty acids, we see the activation of a carboxylic acid by its conversion to a thioester, as we saw with acetyl-CoA in the citric acid cycle. To break the C–C bonds in the long chain of relatively inert –CH2–CH2– groups in fatty acids, a carbonyl group is created adjacent to the – CH2– group, as we saw in the reactions of the citric acid cycle. Dehydration of L-β-Hydroxyacyl-CoA β-hydroxyacyl-CoA dehydrogenase = catalyzes the dehydrogenation of L-β- hydroxyacyl-CoA to form β-ketoacyl-CoA – enzyme is specific for the L stereoisomer – closely analogous to the malate dehydrogenase reaction of the citric acid cycle Electrons from NADH Enter the Mitochondrial Respiratory Chain NADH dehydrogenase (Complex I) = electron carrier of the respiratory chain – accepts electrons from the NADH formed in the β- hydroxyacyl-CoA dehydrogenase reaction Cleavage of the β-Ketoacyl-CoA acyl-CoA acetyl- transferase (thiolase) = catalyzes the reaction of β-ketoacyl-CoA with free coenzyme A to yield acetyl CoA and a fatty acyl-CoA shortened by two carbons – reverse Claisen condensation Clicker Question 15 The reactions of mitochondrial β oxidation do NOT include: A. a hydratase B. a thiolase C. a dehydrogenase D. an oxidase Clicker Question 15, Response The reactions of mitochondrial β oxidation do NOT include: D. an oxidase The four enzymes of mitochondrial β oxidation are: acyl-CoA dehydrogenase enoyl-CoA hydratase β-hydroxyacyl-CoA dehydrogenase acyl-CoA acetyltransferase (thiolase) Trifunctional Protein (TFP) trifunctional protein (TFP) = a multienzyme complex associated with the inner mitochondrial membrane that catalyzes steps 2-4 of the β-oxidation pathway for fatty acyl chains of 12+ carbons – allows efficient substrate channeling TFP is a heterooctamer of α4β4 subunits: – α subunits contain enoyl-CoA hydratase and β- hydroxyacyl-CoA dehydrogenase activity – β subunits contain thiolase activity Principle 2 (5 of 5) Evolution selects for chemical mechanisms that make useful reactions more energetically favorable, and those same mechanisms are used in different pathways. In the breakdown of fatty acids, we see the activation of a carboxylic acid by its conversion to a thioester, as we saw with acetyl-CoA in the citric acid cycle. To break the C–C bonds in the long chain of relatively inert –CH2–CH2– groups in fatty acids, a carbonyl group is created adjacent to the – CH2– group, as we saw in the reactions of the citric acid cycle. The Chemical Logic of the β- Oxidation Sequence the first three reactions convert the stable single bond between methylene groups to a much less stable C–C bond the ketone function on the β carbon (C-3) makes it a good target for nucleophilic attack the terminal –CH2–CO–S-CoA is a good leaving group, facilitating breakage of the α–β bond A Conserved Reaction Sequence The Four β-Oxidation Steps Are Repeated to Yield Acetyl-CoA and ATP the shortened fatty acyl–CoA reenters the β-oxidation sequence for removal of another, and then another, acetyl-CoA The Overall Reaction for One Pass Through Stage 1 of β Oxidation each pass removes: – one molecule of acetyl-CoA – two pairs of electrons – four protons (H+) the overall reaction (beginning with palmitoyl-CoA) is: palmitoyl-CoA + CoA + FAD + NAD+ + H2O ⟶ myristoyl-CoA + acetyl-CoA + FADH2 + NADH + H+ (17-2) The Overall Reaction for Stage 1 of β Oxidation the overall reaction (beginning with palmitoyl-CoA) is: palmitoyl-CoA + 7CoA + 7FAD + 7NAD+ + 7H2O ⟶ 8 acetyl-CoA + 7FADH2 + 7NADH + 7H+ (17-3) Clicker Question 16 The overall reaction to convert palmitoyl-CoA to 8 acetyl-CoA: A. consumes 8 H2O. B. produces either 7 NADH or 7 FADH2, depending on the organelle. C. requires 8 rounds of β oxidation. D. produces 7 NADH and 7 FADH2. Clicker Question 16, Response The overall reaction to convert palmitoyl-CoA to 8 acetyl-CoA: D. produces 7 NADH and 7 FADH2. Palmitoyl-CoA, the coenzyme A derivative of palmitate (16:0), undergoes 7 rounds of β oxidation, with each round producing 1 NADH via the acyl-CoA dehydrogenase reaction and 1 FADH2 via the β-hydroxyacyl-CoA dehydrogenase reaction. Principle 1 (3 of 5) Metabolites of diverse origin funnel into a few central pathways. Fatty acid catabolism and glycolysis convert quite different starting materials into the same product (acetyl-CoA). The electrons from the oxidative reactions of these pathways and of the citric acid cycle are carried by common cofactors (NAD and FAD) to the mitochondrial respiratory chain leading to oxygen, providing the energy for ATP synthesis by oxidative phosphorylation. FADH2 and NADH Donate Electrons to the Respiratory Chain each FADH2 donates a pair of electrons to ETF – generates 1.5 molecules of ATP each NADH donates a pair of electrons to the mitochondrial NADH dehydrogenase – generates 2.5 molecules of ATP in total, 4 ATP are formed for each pass through β oxidation “Metabolic Water” each pair of electrons transferred from NADH or FADH2 to O2 yields one H2O (“metabolic water”) reduction of O2 by NADH also consumes one H+ per NADH molecule: NADH + H+ + ½O2 → NAD+ + H2O important in hibernating animals and camels Clicker Question 17 Which statement is false about fat oxidation in hibernating bears? A. The energy of fat oxidation allows bears to maintain a body temperature close to normal. B. Amino groups released during fat oxidation can be used to make amino acids. C. Fat oxidation releases water, which replenishes water lost in breathing. D. Degradation of triacylglycerols provides a substrate for gluconeogenesis. Clicker Question 17, Response Which statement is false about fat metabolism in hibernating bears? B. Amino groups released during fat oxidation can be used to make amino acids. Fat metabolism provides bears with energy, water, and glucose precursors, but not amino groups. Urea formed during breakdown of amino acids is reabsorbed in the kidneys and recycled, with the amino groups reused to make new amino acids for maintaining body proteins. The Overall Reaction for Stage 1 of β Oxidation, Including Electron Transfers and Oxidative Phosphorylations the overall reaction is: palmitoyl-CoA + 7CoA + 7O2 + 28Pi + 28ADP ⟶ 8 acetyl-CoA + 28ATP + 7H2O (17-4) Principle 1 (4 of 5) Metabolites of diverse origin funnel into a few central pathways. Fatty acid catabolism and glycolysis convert quite different starting materials into the same product (acetyl-CoA). The electrons from the oxidative reactions of these pathways and of the citric acid cycle are carried by common cofactors (NAD and FAD) to the mitochondrial respiratory chain leading to oxygen, providing the energy for ATP synthesis by oxidative phosphorylation. Acetyl-CoA Can Be Further Oxidized in the Citric Acid Cycle acetyl-CoA produced from the oxidation of fatty acids can be oxidized to CO2 and H2O by the citric acid cycle the overall reaction for the second and third stages of fatty acid oxidation (in the oxidation of palmitoyl-CoA) is: 8 acetyl-CoA + 16O2 + 80Pi + 80ADP ⟶ (17-5) 8CoA + 80ATP + 16CO2 + 16H2O The Overall Reaction for the Complete Oxidation of Palmitoyl-CoA to CO2 and H2O the overall reaction is: palmitoyl-CoA + 23O2 + 108Pi + 108ADP ⟶ CoA + 108ATP + 16CO2 + 23H2O (17-6) ATP Yield During Complete Oxidation of Palmitoyl-CoA Table 17-1 Yield of ATP during Oxidation of One Molecule of Palmitoyl-CoA to CO2 and H2O Enzyme catalyzing the oxidation step Number of NADH Number of ATP or FADH2 formed ultimately formed β Oxidation Acyl-CoA dehydrogenase 7 FADH2 10.5 β-Hydroxyacyl-CoA dehydrogenase 7 NADH 17.5 Citric acid cycle Isocitrate dehydrogenase 8 NADH 20 α-Ketoglutarate dehydrogenase 8 NADH 20 Succinyl-CoA synthetase 8 Succinate dehydrogenase 8 FADH2 12 Malate dehydrogenase 8 NADH 20 Total 108 Clicker Question 18 What is the potential ATP yield from complete oxidation of the coenzyme A derivative of stearic acid (18:0)? A. 54 B. 96 C. 108 D. 122 E. 244 Clicker Question 18, Response What is the potential ATP yield from complete oxidation of the coenzyme A derivative of stearic acid (18:0)? Enzyme catalyzing the oxidation No. of NADH or No. of ATP step FADH2 formed ultimately D. 122 formed β-Oxidation Acyl-CoA dehydrogenase 8 FADH2 12 β-Hydroxyacyl-CoA dehydrogenase 8 NADH 20 Citric acid cycle Isocitrate dehydrogenase 9 NADH 22.5 α-Ketoglutarate dehydrogenase 9 NADH 22.5 Succinyl-CoA synthetase 9 Succinate dehydrogenase 9 FADH2 13.5 Malate dehydrogenase 9 NADH 22.5 Total 122 Clicker Question 19 Why is the net production of ATP from palmitate (16:0) oxidation 106 ATP and not 108 ATP? A. Conversion of palmitate to palmitoyl Co-A consumes the equivalent of 2 ATP. B. The overall reaction for conversion of palmitoyl‐CoA to 16 CO2 has 106 ATP on the products side of the reaction. C. Each NADH produces about 2.5 ATP, and each FADH2 produces about 1.5 ATP. D. The production of H+ by β oxidation inside a mitochondrion results in the loss of 2 ATP. Clicker Question 19, Response Why is the net production of ATP from palmitate oxidation 106 ATP and not 108 ATP? A. Conversion of palmitate to palmitoyl Co-A consumes the equivalent of 2 ATP. The formation of a fatty acyl–CoA is made more favorable by the hydrolysis of two high-energy bonds in ATP. Fatty acid + CoA + ATP ⇌ fatty acyl–CoA + AMP + 2Pi Principle 1 (5 of 5) Metabolites of diverse origin funnel into a few central pathways. Fatty acid catabolism and glycolysis convert quite different starting materials into the same product (acetyl-CoA). The electrons from the oxidative reactions of these pathways and of the citric acid cycle are carried by common cofactors (NAD and FAD) to the mitochondrial respiratory chain leading to oxygen, providing the energy for ATP synthesis by oxidative phosphorylation. Oxidation of Unsaturated Fatty Acids Requires Two Additional Reactions enoyl-CoA hydratase cannot catalyze the addition of H2O to a cis double bond oxidation of unsaturated fatty acids requires two additional enzymes: – enoyl-CoA isomerase (converts cis double bonds to trans) – 2,4-dienoyl-CoA reductase (reduces cis double bonds) Oxidation of a Monounsaturated Fatty Acid oxidation of a monounsaturated fatty acid requires an enoyl-CoA isomerase ∆3,∆2- enoyl-CoA isomerase = isomerizes the cis-∆3-enoyl-CoA to the trans-∆2-enoyl-CoA Oxidation of a Polyunsaturated Fatty Acid requires both enoyl-CoA isomerase and 2,4-dienoyl- CoA reductase to transform the cis-∆3,cis-∆6 intermediate into one that can enter the β-oxidation pathway Oxidation of Linoleoyl-CoA (∆9,12) Oxidation of Linoleoyl-CoA (∆9,12), Continued Clicker Question 20 The β oxidation of polyunsaturated fatty acids: A. uses the same enzymes as β oxidation of saturated fatty acids, with the addition of an isomerase and a reductase. B. requires an entirely different pathway than that used for saturated fatty acids. C. requires propionyl-CoA carboxylase. D. occurs in the cytosol in vertebrates. Clicker Question 20, Response The β oxidation of polyunsaturated fatty acids: A. uses the same enzymes as β oxidation of saturated fatty acids, with the addition of an isomerase and a reductase. Two auxiliary enzymes — an isomerase and a reductase — must act on the common unsaturated fatty acids to transform them into substrates for the β-oxidation pathway. Clicker Question 21 Which additional reactant is required for oxidation of polyunsaturated fatty acids compared with saturated fatty acids? A. biotin B. O2 C. NADPH D. ATP E. FAD Clicker Question 21, Response Which additional reactant is required for oxidation of polyunsaturated fatty acids compared with saturated fatty acids? C. NADPH The enzyme 2,4-dienoyl-CoA reductase is NADPH dependent. Complete Oxidation of Odd-Number Fatty Acids Requires Three Extra Reactions propionate (CH3–CH2–COO−) = three-carbon compounds formed by cattle and other ruminant animals during carbohydrate fermentation odd-number fatty acids are oxidized by the β-oxidation pathway to yield acetyl-CoA and a molecule of propionyl- CoA Oxidation of Propionyl-CoA – Step 1 propionyl-CoA carboxylase = catalyzes the carboxylation of propionyl-CoA to form D- methylmalonyl- CoA – requires the cofactor biotin Oxidation of Propionyl-CoA – Step 2 methylmalonyl-CoA epimerase = catalyzes the epimerization of D- methylmalonyl-CoA to its L stereoisomer Oxidation of Propionyl-CoA – Step 3 methylmalonyl-CoA mutase = catalyzes the intramolecular rearrangement of L-methylmalonyl-CoA to form succinyl-CoA (which can enter the citric acid cycle) – requires 5′-deoxyadenosylcobalamin, or coenzyme B12, as its coenzyme Clicker Question 22 Which factor is NOT associated with β oxidation of fatty acids with an odd number of carbons? A. malonyl-CoA as in intermediate B. a rare example in humans of an enzyme that requires a vitamin B12-derived cofactor C. repeated cycles of β oxidation until a three-carbon fragment remains D. a citric acid cycle intermediate as the end product Clicker Question 22, Response Which factor is not associated with β oxidation of fatty acids with an odd number of carbons? A. malonyl-CoA as in intermediate The intermediates in the β oxidation of odd-number fatty acids are D- and L-methylmalonyl-CoA, not malonyl-CoA. Clicker Question 23 Which product from oxidation of fatty acids CANNOT feed into the citric acid cycle? A. acetyl-CoA B. succinyl-CoA C. succinate D. NADP+ E. FAD Clicker Question 23, Response Which product from oxidation of fatty acids CANNOT feed into the citric acid cycle? D. NADP+ The acetyl-CoA resulting from all the β-oxidation pathways, the succinyl Co-A formed by the oxidation of odd-number fatty acids, and the succinate resulting from the oxidation of polyunsaturated fatty acids can all feed into the citric acid cycle. However, the NADP+ resulting from the reductase step in the oxidation of polyunsaturated fatty acids cannot enter the citric acid cycle. Principle 4 (2 of 6) When a process lacks a critical component—an enzyme, a cofactor, or a regulatory agent—the resulting loss of homeostasis may cause disease across a spectrum of severity. Defects in fatty acid breakdown are no exception. Deficiencies in Propionyl-CoA Carboxylase occurs in ~ 1 in 100,000 babies propionic acidemia = severe acidification of the blood and urine resulting from accumulated propionyl-CoA in the mitochondria being released to the blood as propionate – uses the carnitine shuttle Principle 3 (3 of 4) Allosteric mechanisms and posttranslational regulation (protein phosphorylation) coordinate metabolic processes within a cell. Hormones and growth factors coordinate metabolic activities among tissues and organs. Reciprocal regulation of catabolic and anabolic pathways prevents the inefficiency of futile cycling. Fatty Acid Oxidation Is Tightly Regulated malonyl-CoA = the first intermediate of cytosolic fatty acid synthesis – blocks entry of fatty acids into mitochondria to prevent futile cycling Principle 3 (4 of 4) Allosteric mechanisms and posttranslational regulation (protein phosphorylation) coordinate metabolic processes within a cell. Hormones and growth factors coordinate metabolic activities among tissues and organs. Reciprocal regulation of catabolic and anabolic pathways prevents the inefficiency of futile cycling. Transcription Factors Turn on the Synthesis of Proteins for Lipid Catabolism PPAR family of nuclear receptors = transcription factors that affect many metabolic processes in response to a variety of fatty acid–like ligands PPARα stimulates the synthesis of enzymes required in β oxidation when there is an increased demand for energy from fat catabolism Principle 4 (3 of 6) When a process lacks a critical component—an enzyme, a cofactor, or a regulatory agent—the resulting loss of homeostasis may cause disease across a spectrum of severity. Defects in fatty acid breakdown are no exception. Genetic Defects in Fatty Acyl-CoA Dehydrogenases Cause Serious Disease medium-chain acyl-CoA dehydrogenase (MCAD) = acyl- CoA dehydrogenase isozyme that acts on fatty acids of 4-14 carbons individuals with two mutant MCAD alleles cannot oxidize fatty acids of 6-12 carbons – symptoms include fatty liver, high blood levels of octanoic acid (8:0), coma, and death Principle 4 (4 of 6) When a process lacks a critical component—an enzyme, a cofactor, or a regulatory agent—the resulting loss of homeostasis may cause disease across a spectrum of severity. Defects in fatty acid breakdown are no exception. Other Genetic Defects in Fatty Acid Transport or Oxidation loss of the long-chain β-hydroxyacyl-CoA dehydrogenase activity of the trifunctional protein, TFP defects in the α or β subunits that affect all three activities of TFP Peroxisomes Also Carry Out β Oxidation peroxisomes = organelles found in plants and animals β oxidation in peroxisomes has four steps: – dehydrogenation – addition of water to the resulting double bond – oxidation of the β- hydroxyacyl-CoA to a ketone – thiolytic cleavage by coenzyme A Differences Between the Peroxisomal and Mitochondrial Pathways in peroxisomes, the flavoprotein acyl-CoA oxidase that introduces the double bond passes electrons directly to O2, producing H2O2 – the enzyme catalase cleaves H2O2 to H2O and O2 the peroxisomal system is much more active on very-long- chain fatty acids and branched-chain fatty acids Clicker Question 24 Which statement regarding peroxisomal β oxidation is false? A. Peroxisomal β oxidation occurs in the liver of mammals. B. The acetyl-CoA produced is largely consumed by the peroxisomal citric acid cycle. C. It produces hydrogen peroxide. D. The reactions are similar to those of mitochondrial β oxidation. Clicker Question 24, Response Which statement regarding peroxisomal β oxidation is false? B. The acetyl-CoA produced is largely consumed by the peroxisomal citric acid cycle. Peroxisomes, which lack the enzymes of the citric acid cycle, export the acetyl-CoA that they produce. Principle 4 (5 of 6) When a process lacks a critical component—an enzyme, a cofactor, or a regulatory agent—the resulting loss of homeostasis may cause disease across a spectrum of severity. Defects in fatty acid breakdown are no exception. Genetic Defects in Peroxisomal Oxidation Zellwger syndrome = characterized by an inability to make peroxisomes – individuals lack all metabolism related to peroxisomes X-linked adrenoleukodystrophy (XALD) = characterized by the inability of peroxisomes to oxidize very-long-chain fatty acids – due to the lack of a functional transporter in the peroxisomal membrane Phytanic Acid Undergoes α Oxidation in Peroxisomes phytanic acid = a long-chain fatty acid with methyl branches that is derived from the phytol side chain of chlorophyll – the methyl group on the β carbon makes β oxidation impossible α Oxidation of Phytanic Acid α oxidation = removes a single carbon from the carboxyl end of the fatty acid – converts branched fatty acids to products that can undergo β oxidation to yield acetyl-CoA and propionyl-CoA Refsum disease = results from a genetic defect in phytanoyl-CoA hydroxylase 17.3 Ketone Bodies Principle 5 (2 of 4) The liver plays a unique role in whole-body metabolism. When glucose is unavailable, the liver makes glucose by gluconeogenesis and releases it to the blood for distribution to other tissues, including the brain. During starvation, the liver processes fatty acids into ketone bodies, which, unlike fatty acids, can cross the blood brain barrier and fuel the brain. The “Ketone Bodies” “ketone bodies” = acetone, acetoacetate, and D-β- hydroxybutyrate – formed from acetyl-CoA in the liver acetone is exhaled acetoacetate and D-β- hydroxybutyrate are transported to extrahepatic tissues and converted to acetyl-CoA to be oxidized in the citric acid cycle Clicker Question 25 Acetone resulting from ketone body production is: A. removed from the body by exhalation. B. used as fuel in tissues other than the liver. C. not a ketone body. D. produced by nonenzymatically and enzymatically by decarboxylation of D‐β‐hydroxybutyrate. Clicker Question 25, Response Acetone resulting from ketone body production is: A. removed from the body by exhalation. Acetone, a volatile compound produced in smaller quantities than the other ketone bodies, is exhaled. The exhaled acetone imparts a characteristic odor to the breath. Ketone Bodies, Formed in the Liver, Are Exported to Other Organs as Fuel thiolase = catalyzes the enzymatic condensation of two acetyl-CoA molecules to form acetoacetyl- CoA – reversal of the last step of β oxidation The HMG-CoA Synthase and HMG- CoA Lyase Reactions HMG-CoA synthase = catalyzes the condensation of acetoacetyl-CoA with acetyl-CoA to form β- hydroxy-β-methylglutaryl- CoA (HMG-CoA) HMG-CoA lyase = catalyzes the cleavage of HMG-CoA to free acetoacetate and acetyl-CoA The Decarboxylation and Reduction of Acetoacetate acetoacetate decarboxylase = catalyzes the decarboxylation of acetoacetate to acetone D-β-hydroxybutyrate dehydrogenase = catalyzes the reversible reduction of acetoacetate to D-β-hydroxybutyrate D-β-Hydroxybutyrate as Fuel D-β-hydroxybutyrate dehydrogenase = catalyzes the oxidation of D-β- hydroxybutyrate to acetoacetate in extrahepatic tissue β-ketoacyl-CoA transferase = catalyzes the activation of acetoacetate acetyl-CoA enters the citric acid cycle Clicker Question 26 Which statement is true regarding the reactions of ketone body metabolism? A. The enzymes that catalyze biosynthesis of ketone bodies are found in the cytosol of hepatocytes. B. NADH is produced by catabolism of D-β-hydroxybutyrate. C. Conversion of 2 acetyl-CoA to acetoacetyl-CoA is accompanied by hydrolysis of ATP to AMP and PPi. D. The liver lacks thiolase and therefore cannot use ketone bodies as fuel. Clicker Question 26, Response Which statement is true regarding the reactions of ketone body metabolism? B. NADH is produced by catabolism of D‐β‐hydroxybutyrate. The oxidation of D‐β‐hydroxybutyrate to acetoacetate by D‐β‐hydroxybutyrate dehydrogenase reduces NAD+ to NADH. Principle 5 (3 of 4) The liver plays a unique role in whole-body metabolism. When glucose is unavailable, the liver makes glucose by gluconeogenesis and releases it to the blood for distribution to other tissues, including the brain. During starvation, the liver processes fatty acids into ketone bodies, which, unlike fatty acids, can cross the blood brain barrier and fuel the brain. Ketone Bodies Are Used as Fuels in all Tissues Except Liver the liver lacks β-ketoacyl-CoA transferase the liver is a producer of ketone bodies, not a consumer Principle 4 (6 of 6) When a process lacks a critical component—an enzyme, a cofactor, or a regulatory agent—the resulting loss of homeostasis may cause disease across a spectrum of severity. Defects in fatty acid breakdown are no exception. Principle 5 (4 of 4) The liver plays a unique role in whole-body metabolism. When glucose is unavailable, the liver makes glucose by gluconeogenesis and releases it to the blood for distribution to other tissues, including the brain. During starvation, the liver processes fatty acids into ketone bodies, which, unlike fatty acids, can cross the blood brain barrier and fuel the brain. Ketone Bodies Are Overproduced in Diabetes and during Starvation the accumulation of acetyl-CoA accelerates formation of ketone bodies – extrahepatic tissues do not have the capacity to oxidize them all Clicker Question 27 Which molecule is produced in high concentration as a result of both enhanced gluconeogenesis during starvation and in untreated diabetes, which has the same fate under those two conditions? A. acetyl-CoA B. glyceraldehyde 3-phosphate C. succinate D. NADH E. FADH2 Clicker Question 27, Response Which molecule is produced in high concentration as a result of both enhanced gluconeogenesis during starvation and in untreated diabetes, which has the same fate under those two conditions? A. acetyl-CoA The citric acid cycle is severely slowed under both conditions, and acetyl-CoA concentrations increase, leading to acetoacetate formation (i.e., ketone bodies). Acidosis, Ketosis, and Ketoacidosis acidosis = lowered blood pH – can be caused by increased levels of acetoacetate, and D-β-hydroxybutyrate ketosis = high levels of ketone bodies in the blood and urine ketoacidosis = condition when ketosis and acidosis are combined Individuals with Untreated Diabetes Have High Acetone Levels acetoacetate decarboxylase = catalyzes the decarboxylation of acetoacetate to acetone individuals with untreated diabetes produce large quantities of acetoacetate acetone formed from the decarboxylation of acetoacetate is volatile – imparts a characteristic odor to the breath Clicker Question 28 Starvation and uncontrolled diabetes mellitus can both result in: A. ketosis, implying that the body of a person with uncontrolled diabetes mellitus is acting metabolically as though it is starving. B. ketosis, implying that ketoacidosis is a result of uncontrolled diabetes mellitus but not starvation. C. ketosis, implying that high levels of ketone bodies in the blood must be well-tolerated. D. ketosis, though neither case is due to overproduction of ketone bodies by the liver, but rather because cells are impaired in uptake of ketone bodies. Clicker Question 28, Response Starvation and uncontrolled diabetes mellitus can both result in: A. ketosis, implying that the body of a person with uncontrolled diabetes mellitus is acting metabolically as though it is starving. When the insulin level is insufficient, extrahepatic tissues cannot take up glucose efficiently from the blood. Fatty acid oxidation increases, but the resulting acetyl-CoA cannot pass through the citric acid cycle because cycle intermediates have been drawn off for use as substrates in gluconeogenesis. The accumulation of acetyl-CoA accelerates the formation of ketone bodies.