Chapter 20 Proteins PDF
Document Details
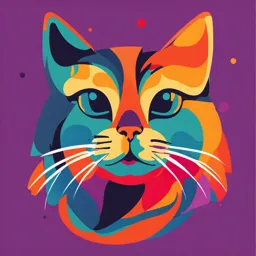
Uploaded by FerventSunstone7303
PHINMA Cagayan de Oro College
Tags
Summary
This chapter details the characteristics of proteins, including their building blocks, amino acids. It explains the different types of amino acids, their properties, and the role of side chains in determining protein properties. It also describes the structural characteristics of proteins.
Full Transcript
Proteins 20 CHAPT ER OU T LI N E...
Proteins 20 CHAPT ER OU T LI N E 20.1 Characteristics of Proteins 707 20.2 Amino Acids: The Building Blocks for Proteins 708 20.3 Essential Amino Acids 710 20.4 Chirality and Amino Acids 711 20.5 Acid–Base Properties of Amino Acids 711 20.6 Cysteine: A Chemically Unique Amino Acid 714 20.7 Peptides 714 20.8 Biochemically Important Small Peptides 718 20.9 General Structural © Jochem Wijnands/Alamy Characteristics of Proteins 719 20.10 Primary Structure of Proteins 720 20.11 Secondary Structure of Proteins 723 20.12 Tertiary Structure of Proteins 726 20.13 Quaternary Structure of The fibrous protein alpha-keratin is the major structural element present in Proteins 730 sheep’s wool. 20.14 Protein Hydrolysis 730 Chemistry at a Glance Protein Structure 731 20.15 Protein Denaturation 732 I n this chapter, the third of the bioorganic classes of molecules (Section 18.1) 20.16 Protein Classification Based is considered, the compounds called proteins. An extraordinary number of on Shape 733 20.17 Protein Classification Based different proteins, each with a different function, exist in the human body. on Function 737 A typical human cell contains about 9000 different kinds of proteins, and the 20.18 Glycoproteins 740 human body contains about 100,000 different proteins. Proteins are needed for 20.19 Lipoproteins 742 the synthesis of enzymes, certain hormones, and some blood components; for Chemical Connections the maintenance and repair of existing tissues; for the synthesis of new tissue; 20-A “Substitutes” for Human and sometimes for energy. Insulin 722 20-B Denaturation and Human Hair 734 20.1 Characteristics of Proteins 20-C Protein Structure and the Color of Meat 738 Next to water, proteins are the most abundant substances in nearly all 20-D Cyclosporine: An Antirejection Drug 743 cells—they account for about 15% of a cell’s overall mass (Section 18.1) and 20-E Colostrum: Immunoglobulins for almost half of a cell’s dry mass. All proteins contain the elements carbon, and Much More 745 hydrogen, oxygen, and nitrogen; most also contain sulfur. The presence of nitro- 20-F Lipoproteins and Heart gen in proteins sets them apart from carbohydrates and lipids, which most often Disease Risk 746 do not contain nitrogen. The average nitrogen content of proteins is 15.4% by mass. Other elements, such as phosphorus and iron, are essential constituents of certain specialized proteins. Casein, the main protein of milk, contains phos- Sign in to OWL at www.cengage.com/owl to view tutorials and simulations, develop phorus, an element very important in the diet of infants and children. Hemoglo- problem-solving skills, and complete online bin, the oxygen-transporting protein of blood, contains iron. homework assigned by your professor. 707 708 Chapter 20 Proteins The word protein comes from the A protein is a naturally occurring, unbranched polymer in which the monomer Greek proteios, which means “of units are amino acids. Thus the starting point for a discussion of proteins is an first importance.” This reflects the key role that proteins play in life understanding of the structures and chemical properties of amino acids. processes. 20.2 Amino Acids: The Building Blocks for Proteins An amino acid is an organic compound that contains both an amino (!NH2) group and a carboxyl (!COOH) group. The amino acids found in proteins are always a-amino acids. An a-amino acid is an amino acid in which the amino group and the carboxyl group are attached to the a-carbon atom. The general structural formula for an a-amino acid is In an a-amino acid, the carboxyl group and the amino group are -Carbon R Side chain attached to the same carbon atom. atom A Carboxyl group The nature of the side chain Amino H2NOCO COOH (R group) distinguishes a-amino group A acids from each other, both H physically and chemically. The R group present in an a-amino acid is called the amino acid side chain. The nature of this side chain distinguishes a-amino acids from each other. Side chains vary in size, shape, charge, acidity, functional groups present, hydrogen- bonding ability, and chemical reactivity. More than 700 different naturally occurring amino acids are known, but only 20 of them, called standard amino acids, are normally present in proteins. A standard amino acid is one of the 20 a-amino acids normally found in proteins. The structures of the 20 standard amino acids are given in Table 20.1. Within Table 20.1, amino acids are grouped according to side-chain polarity. In this system, there are four categories: (1) nonpolar amino acids (2) polar neutral amino acids (3) polar acidic amino acids and (4) polar basic amino acids. This classification system gives insights into how various types of amino acid side chains help determine the proper- ties of proteins (Section 20.12). The nonpolar amino acid proline has A nonpolar amino acid is an amino acid that contains one amino group, one a structural feature not found in any carboxyl group, and a nonpolar side chain. When incorporated into a protein, such other standard amino acid. Its side amino acids are hydrophobic (“water-fearing”); that is, they are not attracted to chain, a propyl group, is bonded to both the a-carbon atom and the water molecules. They are generally found in the interior of proteins, where there is amino nitrogen atom, giving a cyclic limited contact with water. There are nine nonpolar amino acids. Tryptophan is a side chain. borderline member of this group because water can weakly interact through hydro- gen bonding with the NH ring location on tryptophan’s side-chain ring structure. CH2 Thus, some textbooks list tryptophan as a polar neutral amino acid. H2C CH2 The three types of polar amino acids have varying degrees of affinity for water. HN C COOH Within a protein, such amino acids are said to be hydrophilic (“water-loving”). H Hydrophilic amino acids are often found on the surfaces of proteins. Proline A polar neutral amino acid is an amino acid that contains one amino group, one carboxyl group, and a side chain that is polar but neutral. In solution at physiologi- cal pH, the side chain of a polar neutral amino acid is neither acidic nor basic. There are six polar neutral amino acids. These amino acids are more soluble in water than the nonpolar amino acids as, in each case, the R group present can A variety of functional groups are hydrogen bond to water. present in the side chains of the A polar acidic amino acid is an amino acid that contains one amino group and two 20 standard amino acids: six have alkyl groups (Section 12.8), three carboxyl groups, the second carboxyl group being part of the side chain. In solution have aromatic groups (Section 13.11), at physiological pH, the side chain of a polar acidic amino acid bears a negative two have sulfur-containing groups charge; the side-chain carboxyl group has lost its acidic hydrogen atom. There are (Section 14.20), two have hydroxyl two polar acidic amino acids: aspartic acid and glutamic acid. (alcohol) groups (Section 14.2), three A polar basic amino acid is an amino acid that contains two amino groups have amino groups (Section 17.2), two have carboxyl groups (Section and one carboxyl group, the second amino group being part of the side chain. In 16.1), and two have amide groups solution at physiological pH, the side chain of a polar basic amino acid bears (Section 17.12). a positive charge; the nitrogen atom of the amino group has accepted a proton 20.2 Amino Acids: The Building Blocks for Proteins 709 Table 20.1 The 20 Standard Amino Acids, Grouped According to Side-Chain Polarity Below each amino acid’s structure are its name (with pronunciation), its three-letter abbreviation, and its one-letter abbreviation. Nonpolar Amino Acids CH3 CH3 CH3 CH3 CH CH2 H CH3 CH3 CH CH2 CH3 CH H2 N C COOH H2N C COOH H2N C COOH H2N C COOH H2 N C COOH H H H H H Glycine (Gly, G) Alanine (Ala, A) Valine (Val, V) Leucine (Leu, L) Isoleucine (Ile, I) GLY-seen AL-ah-neen VAY-leen LOO-seen eye-so-LOO-seen CH3 NH S CH2 CH2 H2C CH2 CH2 CH2 CH2 HN C COOH H2N C COOH H2N C COOH H2 N C COOH H H H H Proline (Pro, P) Phenylalanine (Phe, F) Methionine (Met, M) Tryptophan (Trp, W) PRO-leen fen-il-AL-ah-neen me-THIGH-oh-neen TRIP-toe-fane Polar Neutral Amino Acids OH SH CH3 CH2 CH2 CH OH H2N C COOH H2N C COOH H2N C COOH H H H Serine (Ser, S) Cysteine (Cys, C) Threonine (Thr, T) SEER-een SIS-teh-een THREE-oh-neen OH O NH2 C O NH2 C CH2 CH2 CH2 CH2 H 2N C COOH H2N C COOH H2 N C COOH H H H Asparagine (Asn, N) Glutamine (Gln, Q) Tyrosine (Tyr, Y) ah-SPAR-ah-geen GLU-tah-meen (TIE-roe-seen) Polar Acidic Amino Acids Polar Basic Amino Acids NH2 NH2 C NH CH2 NH O OH C HN CH2 CH2 O OH N C CH2 CH2 CH2 CH2 CH2 CH2 CH2 CH2 H2N C COOH H2 N C COOH H2N C COOH H 2N C COOH H2N C COOH H H H H H Aspartic acid (Asp, D) Glutamic acid (Glu, E) Histidine (His, H) Lysine (Lys, K) Arginine (Arg, R) ah-SPAR-tic acid glu-TAM-ic acid HISS-tuh-deen LYE-seen ARG-ih-neen 710 Chapter 20 Proteins (basic behavior; Section 17.6). There are three polar basic amino acids: lysine, arginine, and histidine. The names of the standard amino acids are often abbreviated using three- letter codes. Except in four cases, these abbreviations are the first three letters of the amino acid’s name. Also, a one-letter code for amino acid names exists that is particularly useful in computer applications that involve proteins. Both sets of abbreviations are used in specifying the amino acid make-up of protein. Both sets of abbreviations are given in Table 20.1. All of the standard amino acids in Table 20.1 are necessary constituents of human proteins. Adequate amounts of 11 of the 20 standard amino acids can be synthesized from carbohydrates and lipids in the body if a source of nitrogen is also available. However, the adult human body cannot produce adequate amounts of the other nine standard amino acids. These amino acids, which are called essential amino acids, must be obtained from dietary protein. 20.3 Essential Amino Acids Table 20.2 The Essential An essential amino acid is a standard amino acid needed for protein synthesis that Amino Acids must be obtained from dietary sources because the human body cannot synthesize it arginine* methionine in adequate amounts from other substances. There are nine essential amino acids for adults, and a tenth one is needed for growth in children. Table 20.2 lists the histidine phenylalanine essential amino acids. isoleucine threonine The human body can synthesize small amounts of some of the essential amino leucine tryptophan acids, but not enough to meet its needs, especially in the case of growing children. lysine valine A complete dietary protein is a protein that contains all of the essential amino * Arginine is required for growth acids in the same relative amounts in which the body needs them. A complete dietary in children but is not an essential protein may or may not contain all of the nonessential amino acids. Conversely, an amino acid for adults. incomplete dietary protein is a protein that does not contain adequate amounts, rela- tive to the body’s needs, of one or more of the essential amino acids. Associated with the term incomplete dietary protein is the term limiting amino acid. A limiting amino acid is an essential amino acid that is missing, or present in inadequate amounts, in an incomplete dietary protein. Protein from animal sources is usually complete dietary protein. Casein from milk and proteins found in meat, fish, and eggs are complete dietary proteins. There is one common incomplete dietary protein that comes from animal sources. It is gelatin, a protein in which tryptophan is the limiting amino acid. Protein from plant sources tends to be incomplete dietary protein. With plant proteins, three amino acids are often limiting: lysine (wheat, rice, oats, and corn), methionine (beans and peas), and tryptophan (corn and beans). Note that both corn and beans have two limiting amino acids. Soy is the only common plant protein that is a complete dietary protein. Complementary dietary proteins are two or more incomplete dietary proteins that, when combined, provide an adequate amount of all essential amino acids rela- tive to the body’s needs. A mix of plant proteins generally provides high-quality © iStockphoto.com/whitewish (complete) protein. Rice by itself is an incomplete dietary protein, as is beans. A serving of rice and beans provides all of the essential amino acids by protein complementation (Figure 20.1). Prior to the development of genetic engineering procedures (Section 22.14), the quality of a given plant’s protein was something that could not be changed. Genetic modification techniques can improve a plant’s protein by causing it to pro- duce increased amounts of amino acids that it normally has in short supply. Such Figure 20.1 A serving of rice and genetic modification, if fully implemented in the future, would be especially impor- beans, a common meal in many tant in areas of the world that rely heavily on one incomplete protein food source countries, involves complementary (beans or corn or rice); a much higher quality protein would be available to the dietary proteins. people for consumption. 20.5 Acid–Base Properties of Amino Acids 711 20.4 Chirality and Amino Acids Four different groups are attached to the a-carbon atom in all of the standard Glycine, the simplest of the amino acids except glycine, where the R group is a hydrogen atom. standard amino acids, is achiral. All of the other standard amino R acids are chiral. A H2NO CO COOH A H This means that the structures of 19 of the 20 standard amino acids possess a chiral center (Section 18.4) at this location, so enantiomeric forms (left- and right- handed forms; Section 18.5) exist for each of these amino acids. With few exceptions (in some bacteria), the amino acids found in nature and Because only L amino acids are in proteins are L isomers. Thus, as is the case with monosaccharides (Section 18.8), constituents of proteins, the enantiomer designation of L or D nature favors one mirror-image form over the other. Interestingly, for amino acids will be omitted in subsequent amino the L isomer is the preferred form, whereas for monosaccharides the D isomer is acid and protein discussions. It is preferred. understood that it is the L isomer The rules for drawing Fischer projection formulas (Section 18.6) for amino that is always present. acid structures follow. 1. The !COOH group is put at the top of the projection formula, the R group at the bottom. This positions the carbon chain vertically. 2. The !NH2 group is in a horizontal position. Positioning it on the left denotes the L isomer, and positioning it on the right denotes the D isomer. Figure 20.2 shows molecular models that illustrate the use of these rules. Fischer projection formulas for both enantiomers of the amino acids alanine and serine are: COOH COOH COOH COOH Two of the 19 chiral standard amino acids, isoleucine and threonine, H2N H H NH2 H2N H H NH2 possess two chiral centers (see Table 20.1). With two chiral centers CH3 CH3 CH2 CH2 present, four stereoisomers are A A possible for these amino acids. OH OH However, only one of the L isomers L-Alanine D-Alanine L -Serine D-Serine is found in proteins. 20.5 Acid–Base Properties of Amino Acids In pure form, amino acids are white crystalline solids with relatively high decompo- sition points. (Most amino acids decompose before they melt.) Also, most amino acids are not very soluble in water because of strong intermolecular forces within their crystal structures. Such properties are those often exhibited by compounds in which charged species are present. Studies of amino acids confirm that they are charged species both in the solid state and in solution. Why is this so? Mirror Figure 20.2 Designation of handedness in standard amino acid COOH COOH structures involves aligning the carbon chain vertically and looking at the position of the horizontally NH2 H H NH2 aligned !NH2 group. The L form has the !NH2 group on the left, and the D form has the !NH2 group on the right. R R L-Amino acid D-Amino acid 712 Chapter 20 Proteins Both an acidic group (9COOH) and a basic group (9NH2) are present on the same carbon in an a-amino acid. In drawing amino acid structures, H where handedness designation is A Acidic not required, the placement of the Basic H2NOCO COOH group four groups about the a-carbon group A atom is arbitrary. From this point on R in the text, we will draw amino acid structures such that the 9COOH Section 16.8 indicated that in neutral solution, carboxyl groups have a tendency to group is on the right, the 9NH2 lose protons (H1), producing a negatively charged species: group is on the left, the R group points down, and the H atom points !COOH h !COO2 1 H1 up. Drawing amino acids in this “arrangement” makes it easier to Section 17.6 indicated that in neutral solution, amino groups have a tendency to draw structures where amino acids accept protons (H1), producing a positively charged species: are linked together to form longer 1 amino acid chains. O NH2 1 H1 O NH3 Consistent with the behavior of these groups, in neutral solution, the 9COOH group of an amino acid donates a proton to the 9NH2 of the same amino acid. We can characterize this behavior as an internal acid–base reaction. The net result is that in neutral solution, amino acid molecules have the structure H 1 A H3NOCO COO2 A R Strong intermolecular forces Such a molecule is known as a zwitterion, from the German term meaning “double between the positive and negative ion.” A zwitterion is a molecule that has a positive charge on one atom and a negative centers of zwitterions are the charge on another atom, but which has no net charge. Note that the net charge on a cause of the high melting points of amino acids. zwitterion is zero even though parts of the molecule carry charges. In solution and also in the solid state, a-amino acids exist as zwitterions. Zwitterion structure changes when the pH of a solution containing an amino acid is changed from neutral either to acidic (low pH) by adding an acid such as HCl or to basic (high pH) by adding a base such as NaOH. In an acidic solution, the zwitterion accepts a proton (H1) to form a positively charged ion. From this point on in the text, the H H structures of amino acids will be A 1 A1 drawn in their zwitterion form H3NOCO COO2 1 H3O1 H3NOCO COOH 1 H2O unless information given about A A the pH of the solution indicates R R Zwitterion (no net charge) Positively charged ion otherwise. 1 In basic solution, the !NH3 of the zwitterion loses a proton, and a negatively charged species is formed. H H A 1 A 2 H3NOCO COO2 1 OH2 H2NOCO COO 1 H2O A A R R Zwitterion Negatively charged ion The ability of amino acids to react (no net charge) with both H3O1 and OH2 ions means that amino acid solutions can Thus, in solution, three different amino acid forms can exist (zwitterion, nega- function as buffers (Section 10.12). tive ion, and positive ion). The three species are actually in equilibrium with each The same is true for proteins, other, and the equilibrium shifts with pH change. The overall equilibrium process which are amino acid polymers can be represented as follows: (Section 20.1). The buffering action of proteins present in blood is a H H H major function of such proteins. 1A OH2 1 A OH2 A H3NOCO COOH H3NOCO COO2 H2NOCO COO2 A H3O1 A H3O1 A R R R Acidic solution Neutral solution Basic solution (low pH) (pH 5 7.0) (high pH) 20.5 Acid–Base Properties of Amino Acids 713 In acidic solution, the positively charged species on the left predominates; nearly neutral solutions have the middle species (the zwitterion) as the dominant species; in basic solution, the negatively charged species on the right predominates. EX A M PLE 20. 1 Determining Amino Acid Form in Solutions of Various pH Draw the structural form of the amino acid alanine that predominates in solution at each of the following pH values. Guidelines for amino acid form as a function of solution pH follow. a. pH 5 1.0 b. pH 5 7.0 c. pH 5 11.0 Low pH: All acid groups are Solution protonated (!COOH). At low pH, both amino and carboxyl groups are protonated. At high pH, both groups All amino groups + have lost their protons. At neutral pH, the zwitterion is present. are protonated (!NH3). a. H b. H c. H High pH: All acid groups are A 1 1 A A deprotonated H3NOCO COOH H3NOC O COO2 H2NOC O COO2 (!COO2). All amino A A A groups are deproton- CH3 CH3 CH3 ated (!NH2). pH 5 1.0 pH 5 7.0 pH 5 11.0 (net charge of 11) (no net charge) (net charge of 21) Neutral pH: All acid groups are deprotonated (!COO2). All amino Practice Exercise 20.1 groups are protonated + Draw the structural form of the amino acid valine that predominates in solution at (!NH3). each of the following pH values. a. pH 5 7.0 b. pH 5 12.0 c. pH 5 2.0 Answers: a. H b. H c. H A 1 A 1 A H3NOCO COO2 H2NOC O COO2 H3NOC O COOH A A A CH O CH3 CH O CH3 CH O CH3 A A A CH3 CH3 CH3 The previous discussion assumed that the side chain (R group) of an amino acid remains unchanged in solution as the pH is varied. This is the case for neutral amino acids but not for acidic or basic ones. For these latter compounds, the side The term protonated denotes gain chain can also acquire a charge because it contains an amino or a carboxyl group of a H1 ion, and the term deproton- that can, respectively, gain or lose a proton. ated denotes loss of a H1 ion. Because of the extra site that can be protonated or deprotonated, acidic and basic amino acids have four charged forms in solution. These four forms for aspar- tic acid, one of the acidic amino acids, are H H H H A1 OH2 A 1 OH 2 1 A OH2 A H3NOCO COOH H3NOCOCOO2 H3NOCOCOO2 H2NOCOCOO2 A H3O1 A H3O1 A H3O1 A CH2 CH2 CH2 CH2 A A A A COOH COOH COO2 COO2 Low-pH form Moderately-low-pH form Intermediate-pH form High-pH form (11 charge) (no net charge) (21 net charge) (22 net charge) (zwitterion) The existence of two low-pH forms for aspartic acid results from the two carboxyl Side-chain carboxyl groups are groups being deprotonated at different pH values. For basic amino acids, two high- weaker acids than a-carbon carboxyl groups. pH forms exist because deprotonation of the amino groups does not occur simul- taneously. The side-chain amino group deprotonates before the a-amino group for histidine, but the opposite is true for lysine and arginine. 714 Chapter 20 Proteins Table 20.3 Isoelectric Isoelectric Points Points for the 20 Amino Acids An important pH value, relative to the various forms an amino acid can have in Commonly Found in Proteins solution, is the pH at which it exists primarily in its zwitterion form, that is, its Isoelectric neutral form (no net charge). This pH value is known as the isoelectric point for the Name Point amino acid. An isoelectric point is the pH at which an amino acid exists primarily in its zwitterion form. At the isoelectric point, almost all amino acid molecules in a alanine 6.01 solution (more than 99%) are present in their zwitterion form. arginine 10.76 Every amino acid has a different isoelectric point. Fifteen of the 20 amino asparagine 5.41 acids, those with nonpolar or polar neutral side chains (Table 20.1), have isoelectric aspartic acid 2.77 points in the range of 4.8–6.3. The three basic amino acids have higher isoelec- cysteine 5.07 tric points, and the two acidic amino acids have lower ones. Table 20.3 lists the isoelectric points for the 20 standard amino acids. glutamic acid 3.22 glutamine 5.65 glycine 5.97 histidine 7.59 20.6 Cysteine: A Chemically Unique Amino Acid isoleucine 6.02 Cysteine is the only standard amino acid (Table 20.1) that has a side chain that leucine 5.98 contains a sulfhydryl group (9SH group; Section 14.20). The presence of this sulf- hydryl group imparts to cysteine a chemical property that is unique among the lysine 9.74 standard amino acids. Cysteine, in the presence of mild oxidizing agents, readily methionine 5.74 dimerizes, that is, reacts with another cysteine molecule to form a cystine molecule. phenylalanine 5.48 (A dimer is a molecule that is made up of two like subunits.) In cystine, the two proline 6.48 cysteine residues are linked via a covalent disulfide bond. serine 5.68 1 1 Disulfide threonine 5.87 H3N CH COO2 1 H3N CH COO2 COO2 bond COO2 tryptophan 5.88 CH2 CH2 C CH2 S S CH2 C 1 1 tyrosine 5.66 SH SH NH3 NH3 valine 5.97 Cysteine Cysteine Cystine The covalent disulfide bond of cystine is readily broken, using reducing agents, Cystine contains two cysteine to regenerate two cysteine molecules. This oxidation–reduction behavior involving residues linked by a disulfide bond. sulfhydryl groups and disulfide bonds was previously encountered in Section 14.20 when the reactions of thiols were considered. Oxidation SH 1 HS S S 1 2H Reduction As will be shown in Section 20.11, the formation of disulfide bonds between cysteine residues present in protein molecules has important consequences relative to protein structure and protein shape. 20.7 Peptides Under proper conditions, amino acids can bond together to produce an unbranched chain of amino acids. The length of the amino acid chain can vary from a few amino acids to many amino acids. Representative of such chains is the following five-amino-acid chain. Amino acid ! Amino acid ! Amino acid ! Amino acid ! Amino acid Such a chain of covalently linked amino acids is called a peptide. A peptide is an unbranched chain of amino acids. Peptides are further classified by the number of amino acids present in the chain. A compound containing two amino acids is spe- cifically called a dipeptide; three amino acids joined together in a chain constitute a tripeptide; and so on. The name oligopeptide is loosely used to refer to peptides with 10 to 20 amino acid residues, and the name polypeptide is used to refer to longer peptides. A polypeptide is a long unbranched chain of amino acids. 20.7 Peptides 715 Nature of the Peptide Bond The bonds that link amino acids together in a peptide chain are called peptide bonds. There are four peptide bonds present in a pentapeptide. Amino acid — Amino acid — Amino acid — Amino acid — Amino acid h h h h Peptide Peptide Peptide Peptide bond bond bond bond The nature of the peptide bond becomes apparent by reconsidering a chemical reaction previously encountered. In Section 17.16, the reaction between a carbox- ylic acid and an amine to produce an amide was considered. The general equation for this reaction was O H O H B A B A RO CO OH 1 HO NOR RO C O NO R 1 H2O Acid Amine Amide Two amino acids can combine in a similar way—the carboxyl group of one amino acid interacts with the amino group of the other amino acid. The products are a molecule of water and a molecule containing the two amino acids linked by an amide bond. H H H O H H 1 A 1 A 1 A B A A H3NO CO COO 1 H3NO COCOO2 2 H3NO COCONO CO COO2 1 H2O Amide bond formation is an A A A A example of a condensation reaction. R1 R2 R1 R2 Amide bond Removal of the elements of water from the reacting carboxyl and amino groups and the ensuing formation of the amide bond are better visualized when expanded structural formulas for the reacting groups are used. O H O H B A B A O CO O 1 H ONO O C ON O 1 H2O A H Carboxyl Amino Amide group group bond 1 (O COO2) (H3NO) In amino acid chemistry, amide bonds that link amino acids together are given A peptide chain has directionality the specific name of peptide bond. A peptide bond is a covalent bond between the because its two ends are different. There is an N-terminal end and a carboxyl group of one amino acid and the amino group of another amino acid. C-terminal end. By convention, the In all peptides, long or short, the amino acid at one end of the amino acid direction of the peptide chain is 1 sequence has a free H3N group, and the amino acid at the other end of the sequence always 1 has a free COO2 group. The end with the free H3N group is called the N-terminal N-terminal end S C-terminal end end, and the end with the free COO2 group is called the C-terminal end. By con- The N-terminal end is always on vention, the sequence of amino acids in a peptide is written with the N-terminal the left, and the C-terminal end is end amino acid on the left. The individual amino acids within a peptide chain are always on the right. called amino acid residues. An amino acid residue is the portion of an amino acid structure that remains, after the release of H2O, when an amino acid participates in peptide bond formation as it becomes part of a peptide chain. The structural formula for a peptide may be written out in full, or the sequence of amino acids present may be indicated by using the standard three-letter amino acid abbreviations. The abbreviated formula for the tripeptide H O H H O H H A B A A B A A 1 N-terminal H3NOCO CONO COCONO CO COO2 C-terminal end A A A end H CH3 CH2 A OH Glycine Alanine Serine 716 Chapter 20 Proteins which contains the amino acids glycine, alanine, and serine, is Gly–Ala–Ser. When we use this abbreviated notation, by convention, the amino acid at the N-terminal end of the peptide is always written on the left. The repeating sequence of peptide bonds and a-carbon 9CH groups in a peptide is referred to as the backbone of the peptide. O O O CH C NH CH C NH CH C NH CH R1 R2 R3 R4 Backbone of peptide (in color) The R group side chains are considered substituents on the backbone rather than part of the backbone. Thus, structurally, a peptide has a regularly repeating part (the backbone) and a variable part (the sequence of R groups). It is the variable R group sequence that distinguishes one peptide from another. E XAM P L E 20. 2 Converting an Abbreviated Peptide Formula to a Structural Peptide Formula Draw the structural formula for the tripeptide Ala–Gly–Val. Solution Step 1: The N-terminal end of the peptide involves alanine. Its structure is written first. H 1 A H3NO C OCOO2 A CH3 Step 2: The structure of glycine is written to the right of the alanine structure, and a peptide bond is formed between the two amino acids by removing the ele- ments of H2O and bonding the N of glycine to the carboxyl C of alanine. H H 1 A 1 A H3NO CO COO 1 H3NO CO COO2 2 A A CH3 H H O H H 1A B A A H3NOC OC ONOC O COO2 1 H2O A A CH3 H Step 3: To the right of the just-formed dipeptide, draw the structure of valine. Then repeat Step 2 to form the desired tripeptide. H O H H H 1 A B A A 1 A H3NO COC ONO CO COO 1 H3NO CO COO2 2 A A A CH3 H CH O CH3 A CH3 H O H H O H H A B A A B A A 1 H3NOC OCO NOC OC O NO CO COO2 1 H2O A A A CH3 H CH O CH3 A CH3 20.7 Peptides 717 Practice Exercise 20.2 Draw the structural formula for the tripeptide Cys–Ala–Gly. Answer: H O H H O H H 1 A B A A B A A H3NOC OCO NOC OC O NOCO COO2 A A A CH2 CH3 H A SH Peptide Nomenclature Small peptides are named as derivatives of the C-terminal amino acid that is present. The IUPAC rules for doing this are: Rule 1: The C-terminal amino acid residue (located at the far right of the structure) keeps its full amino acid name. Rule 2: All of the other amino acid residues have names that end in -yl. The -yl suffix replaces the -ine or -ic acid ending of the amino acid name, except for tryptophan (tryptophyl), cysteine (cysteinyl), glutamine (glutaminyl), and asparagine (asparaginyl). Rule 3: The amino acid naming sequence begins at the N-terminal amino acid residue. EX AM PLE 20. 3 Determining IUPAC Names for Small Peptides Assign IUPAC names to each of the following small peptides. a. Glu–Ser–Ala b. Gly–Tyr–Leu–Val Solution a. The three amino acids present are glutamic acid, serine, and alanine. Alanine, the C-terminal residue (on the far right), keeps its full name. The other amino acid residues in the peptide receive “shortened” names that end in -yl. The -yl replaces the -ine or -ic acid ending of the amino acid name. Thus glutamic acid becomes glutamyl serine becomes seryl alanine remains alanine The IUPAC name, which lists the amino acids in the sequence from N-terminal residue to C-terminal residue, becomes glutamylserylalanine. b. The four amino acids present are glycine, tyrosine, leucine, and valine. Proceeding as in part a, we note that glycine becomes glycyl tyrosine becomes tyrosyl leucine becomes leucyl valine remains valine Combining these individual names gives the IUPAC name glycyltyrosylleucylvaline. The complete name for a small peptide should actually include a handedness designation (L-designation; Section 20.4) before the name of each residue. For example, the dipeptide serylglycine (Ser–Gly) should actually be L-seryl–L-glycine (L-Ser–L-Gly). The L-handedness designation is, however, usually not included because it is understood that all amino acids present in a peptide, unless otherwise noted, are L enantiomers. Practice Exercise 20.3 Assign IUPAC names to each of the following small peptides. a. Gly–Ala–Leu b. Gly–Tyr–Ser–Ser Answers: a. Glycylalanylleucine; b. Glycyltyrosylserylserine 718 Chapter 20 Proteins Isomeric Peptides Peptides that contain the same amino acids but in different order are different mol- ecules (constitutional isomers) with different properties. For example, two different dipeptides can be formed from one molecule of alanine and one molecule of glycine. Amino acid sequence in a pep- H O H H H O H H tide has biochemical importance. 1 A B A A 1 A B A A Isomeric peptides give different H3NOCO CONO CO COO2 H3NOCO CONOCO COO2 A A A A biochemical responses; that is, CH3 H H CH3 they have different biochemical specificities. Ala] Gly Gly ] Ala In the first dipeptide, the alanine is the N-terminal residue, and in the second mol- ecule, it is the C-terminal residue. These two compounds are isomers with different chemical and physical properties. The number of isomeric peptides possible increases rapidly as the length of For a peptide containing one each the peptide chain increases. Let us consider the tripeptide Ala–Ser–Cys as another of n different kinds of amino acids, the number of constitutional iso- example. In addition to this sequence, five other arrangements of these three com- mers is given by n! (n factorial). ponents are possible, each representing another isomeric tripeptide: Ala–Cys–Ser, 5! 5 5 3 4 3 3 3 2 3 1 5 120 Ser–Ala–Cys, Ser–Cys–Ala, Cys–Ala–Ser, and Cys–Ser–Ala. For a pentapeptide containing five different amino acids, 120 isomers are possible. 20.8 Biochemically Important Small Peptides Many relatively small peptides have been shown to be biochemically active. Func- tions for them include hormonal action, neurotransmission, and antioxidant activity. Small Peptide Hormones Oxytocin plays a role in stimulating The two best-known peptide hormones, both produced by the pituitary gland, are the flow of milk in a nursing mother. oxytocin and vasopressin. Each hormone is a nonapeptide (nine amino acid resi- The baby’s suckling action sends dues) with six of the residues held in the form of a loop by a disulfide bond formed nerve signals to the mother’s brain, triggering the release of oxytocin, from the interaction of two cysteine residues (Section 20.6). Structurally, these via the blood, to the mammary nonapeptides differ in the amino acid present in positions 3 and 8 of the peptide glands. The oxytocin causes muscle chain. In both structures, an amine group replaces the C-terminal single-bonded contraction in the mammary gland, oxygen atom. forcing out milk. As suckling contin- O ues, more oxytocin is released and 1 6 7 8 9 more milk is available for the baby. Cys S S Cys Pro Leu Gly C NH2 Arg 2 5 Tyr Asn Oxytocin Ile Gln 3 Phe 4 Vasopressin Oxytocin regulates uterine contractions and lactation. Vasopressin regulates the excretion of water by the kidneys; it also affects blood pressure. Another name for vasopressin is antidiuretic hormone (ADH). This name relates to vasopressin’s function in the kidneys, which is to decrease urine output in order to decrease water elimination from the body. Such action is necessary when the body becomes dehydrated. Small Peptide Neurotransmitters Enkephalins are pentapeptide neurotransmitters produced by the brain itself that bind at receptor sites in the brain to reduce pain. The two best-known enkepha- lins are Met-enkephalin and Leu-enkephalin, whose structures differ only in the 20.9 General Structural Characteristics of Proteins 719 amino acid residue present at the C-terminal end (Section 20.6) of the peptide; this amino acid difference is incorporated into their names. Met-enkephalin: Tyr–Gly–Gly–Phe–Met Leu-enkephalin: Tyr–Gly–Gly–Phe–Leu The pain-reducing effects of enkephalin action play a role in the “high” The action of another important reported by long-distance runners, in the competitive athlete’s managing to finish peptide hormone, the octapeptide angiotensin, is considered in the the game despite being injured, and in the pain-relieving effects of acupuncture. next chapter (Section 21.10). The action of the prescription painkillers morphine and codeine is based on their binding at the same receptor sites in the brain as the naturally occurring en- kephalins. Enkephalin pain relief is short-term, whereas morphine-codeine pain relief lasts much longer. Enzymes present in the brain readily hydrolyze the peptide linkages in enkephalins; morphine and codeine do not have such linkages and are unaffected by the hydrolysis enzymes. Small Peptide Antioxidants The tripeptide glutathione (Glu–Cys–Gly) is present in significant concentrations Other antioxidants previously in most cells and is of considerable physiological importance as a regulator of considered are BHA and BHT oxidation–reduction reactions. Specifically, glutathione functions as an antioxidant (Section 14.13) and b-carotene (Section 13.7). (Section 14.13), protecting cellular contents from oxidizing agents such as perox- ides and superoxides (highly reactive forms of oxygen often generated within the cell in response to bacterial invasion) (Section 23.11). The tripeptide structure of glutathione has an unusual feature. The amino acid Glu, an acidic amino acid, is bonded to Cys through the side-chain carboxyl group rather than through its a-carbon carboxyl group. O O 1 H3N CH CH2 CH2 C NH CH C NH CH COO2 COO2 CH2 H SH Glu Cys Gly The side chains are circled in the above structure. 20.9 General Structural Characteristics of Proteins In Section 20.1, a protein was defined simply as a naturally occurring, unbranched Proteins are the second type of polymer in which the monomer units are amino acids. A more specific protein defi- biochemical polymer encountered in this text; the other was polysac- nition is now in order. A protein is a peptide in which at least 40 amino acid residues charides (Section 18.14). Protein are present. The defining line governing the use of the term protein— 40 amino acid monomers are amino acids, whereas residues—is an arbitrary line. The terms polypeptide and protein are often used polysaccharide monomers are interchangeably; a protein is a relatively long polypeptide. The key point is that the monosaccharides. term protein is reserved for peptides with a large number of amino acids; it is not correct to call a tripeptide a protein. More than 10,000 amino acid residues are present in several proteins; 400–500 amino acid residues are common in proteins; small proteins contain 40–100 amino acid residues. More than one peptide chain may be present in a protein. On this basis, pro- teins are classified as monomeric or multimeric. A monomeric protein is a protein in which only one peptide chain is present. Large proteins, those with many amino acid residues, usually are multimeric. A multimeric protein is a protein in which more than one peptide chain is present. The peptide chains present in multimeric proteins are 720 Chapter 20 Proteins Table 20.4 Types of Conjugated Proteins Class Prosthetic Group Specific Example Function of Example hemoproteins heme unit hemoglobin carrier of O2 in blood myoglobin oxygen binder in muscles lipoproteins lipid low-density lipoprotein (LDL) lipid carrier high-density lipoprotein (HDL) lipid carrier glycoproteins carbohydrate gamma globulin antibody mucin lubricant in mucous secretions interferon antiviral protection phosphoproteins phosphate group glycogen phosphorylase enzyme in glycogen phosphorylation nucleoproteins nucleic acid ribosomes site for protein synthesis in cells viruses self-replicating, infectious complex metalloproteins metal ion iron–ferritin storage complex for iron zinc–alcohol dehydrogenase enzyme in alcohol oxidation called protein subunits. The protein subunits within a multimeric protein may all be identical to each other or different kinds of subunits may be present. Proteins with up to 12 subunits are known. The small protein insulin, which functions as a hor- mone in the human body, is a multimeric protein with two protein subunits; one subunit contains 21 amino acid residues and the other 30 amino acid residues. The structure of insulin is considered in more detail in Section 20.12. Proteins, on the basis of chemical composition, are classified as simple or complex. A simple protein is a protein in which only amino acid residues are present. More than one protein subunit may be present in a simple protein, but all subunits contain only amino acids. A conjugated protein is a protein that has one or more non-amino acid entities present in its structure in addition to one or more peptide chains. These non-amino acid components, which may be organic or inorganic, are called prosthetic groups. A prosthetic group is a non-amino acid group present in a conjugated protein. Conjugated proteins may be further classified according to the nature of the prosthetic group(s) present. Lipoproteins contain lipid prosthetic groups, glycopro- teins contain carbohydrate groups, metalloproteins contain a specific metal, and so on (Table 20.4). Some proteins contain more than one type of prosthetic group. In general, prosthetic groups have important roles in the biochemical functions for conjugated proteins. Several examples of glycoproteins and lipoproteins are discussed in Sections 20.18 and 20.19, respectively. In general, the three-dimensional structures of proteins, even those with just a single peptide chain, are more complex than those of carbohydrates and lipids— the biomolecules discussed in the two previous chapters. Describing and under- standing this complexity in protein structure involves considering four levels of protein structure. These four protein structural levels, listed in order of increas- ing complexity, are primary structure, secondary structure, tertiary structure, and quaternary structure. They are the subject matter for the next four sections of this chapter. 20.10 Primary Structure of Proteins The primary structure of a protein Primary protein structure is the order in which amino acids are linked together in a is the sequence of amino acids in protein. Every protein has its own unique amino acid sequence. Primary protein a protein chain—that is, the order structure always involves more than just the numbers and kinds of amino acids in which the amino acids are con- nected to each other. present; it also involves the order of attachment of the amino acids to each other through peptide bonds. 20.10 Primary Structure of Proteins 721 Insulin, the hormone that regulates blood-glucose levels, was the first protein for which primary structure was determined; the “sequencing” of its 51 amino acids was completed in 1953, after eight years of work by the British biochem- ist Frederick Sanger (Figure 20.3). Today, primary structures are known for many thousands of proteins, and the sequencing procedures involve automated meth- ods that require relatively short periods of time (days). Figure 20.4 shows the pri- mary structure of myoglobin, a protein involved in oxygen transport in muscles; it contains 153 amino acids assembled in the particular, definite order shown in this Hulton Archives/Getty Images diagram. The primary structure of a specific protein is always the same regardless of where the protein is found within an organism. The structures of certain proteins are even similar among different species of animals. For example, the primary structures of insulin in cows, pigs, sheep, and horses are very similar both to each other and to human insulin. Until recently, this similarity was particularly impor- tant for diabetics who required supplemental injections of insulin. The focus on relevancy feature Chemical Connections 20-A on the next page considers several Figure 20.3 The British biochemist old and new aspects of insulin chemistry. Frederick Sanger (1918– ) determined the primary structure of the protein hormone insulin in + Lys Gln Leu 1953. His work is a landmark in NH3 Asp Phe Ala Ser Glu Phe biochemistry because it showed for the first time that a protein has Gly Phe Lys Leu His Leu Arg a precisely defined amino acid Leu Lys His Pro Ala Ala Lys sequence. Sanger was awarded the Nobel Prize in chemistry in 1958 Ser Glu Leu Lys Thr Lys Asp for this work. Later, in 1980, he was Asp Leu Lys Ile Lys Asp Met awarded a second Nobel Prize in chemistry, this time for work that Gly Thr Ser Glu His Met Ala involved the sequencing of units in Glu Glu Glu Ala Lys Ala Ser nucleic acids (Chapter 22). Trp Pro Asp Glu Ile Gly Asn Gln His Glu His Pro Gln Tyr Leu Gly Met His Val Ala Lys Val Lys Lys Gly Lys Asp Glu Leu Phe Ala Lys Tyr Ala Leu Asn Leu Ser Lys Leu Gly Gly Val Arg Glu Lys Glu Phe Phe Trp Ile Asp Leu Phe Asp Gln Gly Leu Leu Ile Ile Gly Gly Lys Val Lys Gly Ser Pro COO– Val Glu Lys Gly Glu His Glu Gln His Leu Cys Lys Ala Gly Gly Ala Ile Ser Asp His Ala Thr Ile Gln Ile Gly Thr Leu Gln Leu Pro Val Val Figure 20.4 The primary structure of human myoglobin. This diagram gives only the sequence of the amino acids present and conveys no information about the actual three- dimensional shape of the protein. The “wavy” pattern for the 153 amino acid sequence was chosen to minimize the space used to present the needed information. The actual shape of the protein is determined by secondary and tertiary levels of protein structure, levels yet to be discussed. 722 Chapter 20 Proteins C HE MIC AL CONNECTIONS 20-A “Substitutes” for Human Insulin Insulin is a 51-amino-acid-containing protein hormone Porcine and human insulin match at 50 of the 51 amino whose structure is given later in the chapter (Section 20.12). acid positions, and bovine and human insulin match at 48 of Its function within the human body primarily involves regu- the 51 amino acid positions (see the table that follows and lation of blood-glucose levels. It assists the entry of blood Figure 20.10). glucose into cells by interacting with receptors on cell mem- branes (Section 19.10). It also helps facilitate the conversion Chain A Chain B of glucose to the storage polysaccharide glycogen (Section Species #8 #9 #10 #30 18.15) when blood-glucose levels become too high and facili- tates the reverse process (conversion of glycogen back to glu- human Thr Ser Ile Thr cose) when blood-glucose levels become too low. pig (porcine) Thr Ser Ile Ala Insufficient insulin production or an inability to use in- cow (bovine) Ala Ser Val Ala sulin produced (insulin resistance) result in the condition diabetes mellitus. Treatment of this condition often involves The dependency of diabetics on animal insulin is now giving a person insulin via subcutaneous injection (see ac- on the decline because of the availability of human insulin companying photo). produced by genetically engineered bacteria (Section 22.14). These bacteria carry a gene that directs the synthesis of human insulin. Such bacteria-produced insulin is fully func- tional. All diabetics now have the choice of using human insulin or using animal insulin. Many still continue to use the animal insulin because it is cheaper. Biosynthetic “human” insulin has now gone beyond the “bacteria stage.” Researchers have successfully introduced the gene for human insulin into plants, specifically the saf- © Svanblar/Shutterstock.com flower plant, which then produce the insulin. It is anticipated that this advance will significantly reduce biosynthetic insulin production costs. Several of the “new” insulins researchers have obtained are called insulin analogs because they are not exact copies of human insulin but, rather, slightly modified copies of human insulin. The modifications are planned modifications. These insulin analogs affect glucose levels in the same manner as Currently, diabetics who require insulin must obtain it “regular” insulin but often have better absorption rates and through injection rather than orally. longer periods of bioactivity. The onset of action for insu- lin analogs can be as short as 15 minutes compared to usual For many years, because of the limited availability of hu- rates of beyond an hour. Some insulin analogs remain active man insulin, most insulin used by diabetics was obtained for a period of 18 to 24 hours; in essence, they are “extended from the pancreases of slaughter-house animals. Such ani- release” insulins. mal insulin, obtained primarily from cows and pigs, was Another active area of research is the development of used by most diabetics without serious side effects because it insulin forms that can be taken orally. Currently, insulins is structurally very similar to human insulin. Immunological cannot be taken orally because they, like other proteins, reactions gradually do increase over time, however, because lose their activity when they encounter the stomach’s di- the animal insulin is foreign to the human body. gestive enzymes. Oral insulin research involves developing In terms of primary structure, human insulin, porcine methods for protecting insulin formulations from digestive (pig) insulin, and bovine (cow) insulin are very similar. enzymes. An analogy is often drawn between the primary structure of proteins and words. Words, which convey information, are formed when the 26 letters of the English alphabet are properly sequenced. Proteins are formed from proper sequences of the 20 standard amino acids. Just as the proper sequence of letters in a word is necessary for it to make sense, the proper sequence of amino acids is necessary to make biochemically active protein. Furthermore, the letters that form a word are written from left to right, as are amino acids in protein formulas. As any dictionary of the English language will document, a tremendous variety of words can be formed by different letter sequences. Imagine the number of amino acid 20.11 Secondary Structure of Proteins 723 sequences possible for a large protein. There are 1.55 3 1066 sequences possible for the 51 amino acids found in insulin! From these possibilities, the body reliably pro- duces only one, illustrating the remarkable precision of life processes. From the sim- plest bacterium to the human brain cell, only those amino acid sequences needed by the cell are produced. The fascinating process of protein biosynthesis and the way in which genes in DNA direct this process will be discussed in Chapter 22. The various amino acids present in a protein, whose order is the primary struc- ture of the protein, are linked to each other by peptide linkages. The peptide bonds are part of the “backbone” of the protein. The structural characteristics of a pro- tein backbone are the same as those of a peptide backbone (Section 20.7); relative to backbone structure, a protein is simply an “extra long” peptide. A representative segment of a protein backbone is as follows: O O O B B B Segment of a OCHOCONHOCHOCONHOCHOCONHOCHO protein backbone A A A A R1 R2 R3 R4 Attached to the backbone, at the CH locations, are the various amino acid R groups. The carbon and nitrogen atoms of a protein backbone are arranged in a “zigzag” manner. This zigzag pattern arises from the geometric characteristics of peptide bonds. Important points concerning peptide bond geometry are: 1. The peptide linkages are essentially planar. This means that for two amino acids linked through a peptide linkage, six atoms lie in the same plane: the a-carbon atom and the C"O group from the first amino acid and the N!H group and the a-carbon atom from the second amino acid. O R H C Ca Ca N Plane of peptide bond H R H 2. The planar