Chapter 19: d and f-Block Elements PDF
Document Details
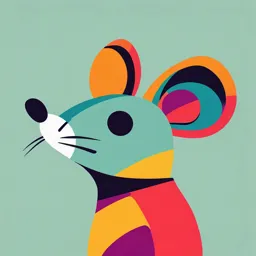
Uploaded by NourishingRoseQuartz
null
Tags
Summary
This chapter covers d and f-block elements, specifically their electronic configurations, oxidation states, and various physical properties like atomic radii, ionization energies and more. The discussion also includes a comparative analysis of elements in different transition series.
Full Transcript
833 60 d and f-Block Elements Chapter E3 19 d and f-Block Elements Cobalt Co 27 [Ar] 3d74s2 Nickel Ni 28 [Ar] 3d84s2 Copper Cu* 29 [Ar] 3d104s1 Zinc Zn 30 [Ar] 3d104s2 ID U A transition element may be defined as an element whose atom in the ground state or ion in common oxidation state has incomplet...
833 60 d and f-Block Elements Chapter E3 19 d and f-Block Elements Cobalt Co 27 [Ar] 3d74s2 Nickel Ni 28 [Ar] 3d84s2 Copper Cu* 29 [Ar] 3d104s1 Zinc Zn 30 [Ar] 3d104s2 ID U A transition element may be defined as an element whose atom in the ground state or ion in common oxidation state has incomplete sub-shell, has to 2. The definition of transition metal excludes Zn, Cd and Hg because they have complete d- orbital. Their common oxidation state is Zn , Cd , Hg. They also do U not show the characteristics of transition element. Element of group 3 (Sc, Y, La and Ac) and group 12 ( Zn, Cd , Hg ) are called non typical transition element. ST Table : 19.1 First transition or 3d series : Symbo l At. No. Scandium Sc 21 Titanium Ti 22 Vanadium V 23 * Chromiu m Cr Manganes e Mn Iron Fe 24 25 26 3d-orbitals are filled up Element Electronic configuration [Ar] 3d14s2 Element 5 Symbol 39 [Kr] 4d15s2 Zirconium Zr 40 [Kr] 4d25s2 Niobium Nb* 41 Molybdenum Mo* 42 Technetium Tc 43 Ruthenium Ru* 44 Rhodium Rh* 45 Palladium Pd* 46 Silver Ag* 47 [Kr] 4d105s1 Cadmium Cd 48 [Kr] 4d105s2 [Kr] 4d45s1 [Kr] 4d55s1 [Kr] 4d55s2 [Kr] 4d75s1 [Kr] 4d85s1 [Kr] 4d105s0 Table : 19.3 Third transition or 5d-series : Element [Ar] 3d 4s [Ar] 3d64s2 Electronic configuratio n Y 1 [Ar] 3d54s2 At. No. Yttrium [Ar] 3d24s2 [Ar] 3d34s2 Table : 19.2 Second transition or 4d-series : 4d-orbitals are filled up (n 1)1 to 10 ns 0 D YG electron 1 to 9. It is called transition element due to fact that it is lying between most electropositive (sblock) and most electronegative (p-block) elements and represent a transition from them. The general electronic configuration of these element is Symbo l At. No. Lanthanu m La 57 Hafnium Hf 72 Tantalum Ta 73 Electronic configuration 5d-orbitals are filled up d-Block elements [Xe] 5d16s2 [Xe] 4f145d26s2 [Xe] 4f145d36s2 834 d and f-Block Elements 74 [Xe] 4f145d46s2 Rhenium Re 75 [Xe] 4f145d56s2 Osmium Os 76 [Xe] 4f145d66s2 Iridium Ir 77 [Xe] 4f145d76s2 Platinum Pt* 78 [Xe] 4f145d106s0 Gold Au* 79 [Xe] 4f145d106s! Mercury Hg 80 [Xe] 4f145d106s2 Table : 19.4 Fourth transition or 6d-series : Symbo l Actinium Ac 89 [Rn] 6d17s2 Rutherfordiu m Rf 10 4 [Rn] 5f146d27s2 Hahnium Ha 10 5 [Rn] 5f146d37s2 Seaborgium Sg 10 6 Bohrium Bh 107 10 8 Meitnerium Mt 10 9 Ununnilium Uun 110 Unununium Unubium ID Hs [Rn] 5f146d47s2 [Rn] 5f146d57s2 14 6 2 [Rn] 5f 6d 7s [Rn] 5f146d77s2 [Rn] 5f146d87s2 D YG Hassium Electronic configuration Uuu 111 [Rn] 5f146d97s2 Uub 112 [Rn] 5f146d107s2 Elements marked with asterisk have anomalous configurations. These are attributed to factors like nuclear-electron and electron-electron forces and stability of half filled and full filled orbital. U All transition elements are d block elements but all d block elements are not transition elements. Physico-Chemical Properties of d-Block Elements (1) Atomic radii : The atomic, radii of 3d-series of elements are compared with those of the neighbouring s and p-block elements. ST Explanation : When we go in any transition series from left, to right, the nuclear charge increases gradually by one unit at each elements. The added electrons enter the same penultimate shell, (inner dshell). These added electrons shield the outermost electrons from the attraction of the nuclear charge. The increased nuclear charge tends to reduce the atomic radii, while the added electrons tend to increase the atomic radii. At the beginning of the series, due to smaller number of electrons in the d-orbitals, the effect of increased nuclear charge predominates, and the atomic radii decrease. Later in the series, when the number of d-electrons increases, the increased shielding effect and the increased repulsion between the electrons tend to increase the atomic radii. Somewhere in the middle of the series, therefore the atomic radii tend to have a minimum value as observed. (ii) The atomic radii increase while going down in each group. However, in the third transition series from hafnium (Hf) and onwards, the elements have atomic radii nearly equal to those of the second transition elements. U At. No. 6d-orbitals are filled up Element the atomic number. The decrease however, is not regular. The atomic radii tend to reach minimum near at the middle of the series, and increase slightly towards the end of the series. 60 W E3 Tungsten K Ca Sc Ti V Cr Mn 227 197 144 132 122 117 117 Fe Co Ni Cu Zn Ga Ge 117 116 115 117 125 135 122* * in pm units The atomic radii of transition elements show the following characteristics, (i) The atomic radii and atomic volumes of dblock elements in any series decrease with increase in Explanation : The atomic radii increase while going down the group. This is due to the introduction of an additional shell at each new element down the group. Nearly equal radii of second and third transition series elements is due to a special effect called lanthanide contraction. (2) Ionic radii : For ions having identical charges, the ionic radii decrease slowly with the increase in the atomic number across a given series of the transition elements. Elements (m): Ionic radius,(M2+)/pm: Pm:(M3+)/pm: Sc – 81 Ti 90 76 V 88 74 Cr 84 69 Mn 80 66 Fe 76 64 Co 74 63 Ni 72 – Cu 69 – Zn 74 – Explanation : The gradual decrease in the values of ionic radius across the series of transition elements is due to the increase in the effective nuclear charge. d and f-Block Elements I1 I2 I3 Sc 632 1245 2450 Ti 659 1320 2721 V 650 1376 2873 Cr 652 1635 2994 Mn 716 1513 3258 Fe 762 1563 2963 Co 758 1647 3237 Ni 736 1756 3400 Cu 744 1961 3560 906 1736 3838 Zn * in kJ mol –1 The following generalizations can be obtained from the ionisation energy values given above. ID (i) The ionisation energies of these elements are high and in the most cases lie between those of s- and p-block elements. This indicates that the transition elements are less electropositive than s-block elements. E3 Elements These elements occur in three types e.g., face- centered cubic (fcc), hexagonal close-packed (hcp) and bodycentered cubic (bcc), structures. The transition elements shows both covalent as well as metallic bonding amongst their atoms. Explanation : The ionisation energies of the transition elements are not very high. The outermost shell in their atoms have many vacant, partially filled orbitals. These characteristics make these elements metallic in character. The hardness of these metals, suggests the presence of covalent bonding in these metals. The presence of unfilled d-orbitals favour covalent bonding. Metallic bonding in these metals is indicated by the conducting nature of these metals. Therefore, it appears that there exists covalent and metallic bonding in transition elements. (5) Melting and boiling points : The melting and boiling points of transition elements except Cd and Hg, are very high as compared to the s-block and p-block elements. The melting and boiling points first increase, pass through maxima and then steadily decrease across any transition series. The maximum occurs around middle of the series. Explanation : Atoms of the transition elements are closely packed and held together by strong metallic bonds which have appreciable covalent character. This leads to high melting and boiling points of the transition elements. The strength of the metallic bonds depends upon the number of unpaired electrons in the outermost shell of the atom. Thus, greater is the number of unpaired electrons stronger is the metallic bonding. In any transition element series, the number of unpaired electrons first increases from 1 to 5 and then decreases back to the zero.The maximum five unpaired electrons occur at Cr (3d series). As a result, the melting and boiling points first increase and then decrease showing maxima around the middle of the series. The low melting points of Zn, Cd, and Hg may be due to the absence of unpaired d-electrons in their atoms. 60 (3) Ionisation energies : The ionisation energies of the elements of first transition series are given below: 835 D YG U Explanation : Transition metals have smaller atomic radii and higher nuclear charge as compared to the alkali metals. Both these factors tend to increase the ionisation energy, as observed. ST U (ii) The ionisation energy in any transition series increases in the nuclear with atomic number; the increase however is not smooth and as sharp as seen in the case of s and p-block elements. Explanation : The ionisation energy increases due to the increase in the nuclear charge with atomic number at the beginning of the series. Gradually, the shielding effect of the added electrons also increases. This shielding effect tends to decrease the attraction due to the nuclear charge. These two opposing factors lead to a rather gradual increase in the ionisation energies in any transition series. (iii) The first ionisation energies of 5d-series of elements are much higher than those of the 3d and 4d series elements. Explanation : In the 5d-series of transitions elements, after lanthanum (La), the added electrons go to the next inner 4f orbitals. The 4f electrons have poor shielding effect. As a result, the outermost electrons experience greater nuclear attraction. This leads to higher ionisation energies for the 5d- series of transition elements. (4) Metallic character : All the transition elements are metals. These are hard, and good conductor of heat and electricity. All these metals are malleable, ductile and form alloys with other metals. (6) Enthalpies of atomization : Transition metals exhibit high enthalpies of atomization. Explanation : This is because the atoms in these elements are closely packed and held together by strong metallic bonds. The metallic bond is formed as a result of the interaction of electrons in the outermost shell. Greater the number of valence electrons, stronger is the metallic bond. (7) Oxidation states : Most of the transition elements exhibit several oxidation states i.e., they show variable valency in their compounds. Some common oxidation states of the first transition series elements are given below in table, 836 d and f-Block Elements Outer Ele. Confi. and O. S. for 3d- elements 3d1 4s2 Oxidation states + 2, + 3 Ti 2 3d 4s 2 + 2, + 3, + 4 V 3 3d 4s 2 + 2,+ 3,+ 4,+ 5 Cr 3d5 4s1 + 1, + 2, + 3, + 4, + 5, + 6 Mn 5 3d 4s 2 + 2, + 3, + 4, + 5, + 6, + 7 6 2 + 2, + 3, + 4, + 5, + 6 Fe 3d 4s Co 3d74s2 + 2, + 3, + 4 8 + 2, + 3, + 4 2 Ni 3d 4s Cu 3d104s1 Zn 10 3d 4s 2 (v) Within a group, the maximum oxidation state increases with atomic number. For example, iron shown the common oxidation state of + 2 and + 3, but ruthenium and osmium in the same group form compounds in the + 4, + 6 and + 8 oxidation states. 60 Sc Outer electronic configuration (vi) Transition metals also form compounds in low oxidation states such as +1 and 0. For example, nickle in, nickel tetracarbonyl, Ni(CO)4 has zero oxidation state. Similarly Fe in (Fe(CO )5 has zero + 1,+ 2 +2 oxidation state. The bonding in the compounds of transition metals in low oxidation states is not always very simple. (vii) Ionisation energies and the stability of oxidation states :The values of the ionisation energies can be used in estimating the relative stability of various transition metal compounds (or ions). For example, Ni2+ compounds are found to be thermodynamically more stable than Pt2+, whereas Pt4+ compounds are more stable than Ni4+ compounds. The relative stabilities of Ni2+ relative to Pt2+ and that of Pt4+ relative to Ni4+ can be explained as follows, D YG U ID Explanation : The outermost electronic configuration of the transition elements is (n - 1)d110 ns2. Since, the energy levels of (n-1)d and ns-orbitals are quite close to each other, hence both the ns and (n1) d-electrons are available for bonding purposes. Therefore, the number of oxidation states show by these elements depends upon the number of delectrons it has. For example, Sc having a configuration 3d 1 4s 2 may show an oxidation state of + 2 (only s-electrons are lost) and + 3 (when delectron is also lost). The highest oxidation state which an elements of this group might show is given by the total number of ns and (n -1) delectrons. E3 Elements (iv) The transition elements in the + 2 and + 3 oxidation states mostly form ionic bonds. In compounds of the higher oxidation states (compound formed with fluorine or oxygen), the bonds are essentially covalent. For example, in permanganate ion MnO4–, all bonds formed between manganese and oxygen are covalent. U The relative stability of the different oxidation states depends upon the factors such as, electronic configuration, nature of bonding, stoichiometry, lattice energies and solvation energies. The highest oxidation states are found in fluorides and oxides because fluorine and oxygen are the most electronegative elements. The highest oxidation state shown by any transition metal is eight. The oxidation state of eight is shown by Ru and Os. ST An examination of the common oxidation states reveals the following conclusions. (i) The variable oxidation states shown by the transition elements are due to the participation of outer ns and inner (n–1)d-electrons in bonding. (ii) Except scandium, the most common oxidation state shown by the elements of first transition series is +2. This oxidation state arises from the loss of two 4s electrons. This means that after scandium, d-orbitals become more stable than the s-orbital. (iii) The highest oxidation states are observed in fluorides and oxides. The highest oxidation state shown by any transition elements (by Ru and Os) is 8. The first four ionisation energies of Ni and Pt Metal (IE1+IE2) kJmol-1, (IE3+IE4) -1 kJmol , Ni 2490 8800 Pt 2660 6700 Etotal, kJ mol-1 (= IE1 + IE2 + IE3 + IE4) 11290 9360 2+ Thus, the ionisation of Ni to Ni requires lesser energy (2490 kJ mol–1) as compared to the energy required for the production of Pt2+ (2660 kjmol–1). Therefore, Ni2+ compounds are thermodynamically more stable than Pt2+ compounds. On the other hand, formation of Pt4+ requires lesser energy (9360 kJ mol–1) as compared to that required for the formation of Ni4+(11290 kJ mol–1). Therefore, Pt4+ compounds are more stable than Ni 4+ compounds. This is supported by the fact that [PtCl6]2– complex ion is known, while the corresponding ion for nickel is not known. However, other factors which affect the stability of a compound are, (a) Enthalpy of sublimation of the metal. (b) Lattice and the solvation energies of the compound or ion. d and f-Block Elements (viii) Transition elements like Sc, Y, La and Ac do not show variable valency. (8) Electrode potentials (Eo) : Standard electrode potentials of some half–cells involving 3d-series of transition elements and their ions in aqueous solution are given in table, Standard electrode potentials for 3d-elements E°/ volt Sc Sc + 3e Sc – 2.10 Ti Ti2+ Ti2++ 2e– Ti – 1.60 V V2+ V2++ 2e– V – 1.20 3+ 3+ 3+ – – Cr Cr Mn Mn2+ Mn2++ 2e– Mn – 1.18 Fe Fe2+ Fe2+ + 2e– Fe – 0.44 Co 2+ Co + 2e Co – 0.28 Ni 2+ Ni Ni2+ + 2e– Ni – 0.24 Cu Cu2+ Cu2+ + 2e– Cu + 0.34 Zn 2+ + 2e Zn – 0.76 Co Zn 2+ Zn 2+ + 3e Cr Cr – – M (s) – 0.71 Thus, this reduction reaction will take place if the electrode potential for Mn+/M half- cell is positive. The reverse reaction, M(s) Mn+(aq) + ne– are favourable in the forward direction. In actual practice however, most of these metals react with dilute acids very slowly. Some of these metals get coated with a thin protective layer of oxide. Such an oxide layer prevents the metal to react further. U (ii) These metals should act as good reducing agents. There is no regular trend in the E° values. This is due to irregular variation in the ionisation and sublimation energies across the series. Relative stabilities of transition metal ions in different oxidation states in aqueous medium can be ST data. To ; H1 Enthalpy of sublimation, Hsub M (g) M (g) e ; H 2 Ionisation energy , IE M (g) M (aq) ; H 3 Enthalpy of hydration, H hyd Adding these equations one gets, M (s) M (aq) e Involving the formation of Mn+(aq) will occur if the electrode potential is negative, i.e., the tendency for the formation of Mn+(aq) from the metal M will be more if the corresponding E° value is more negative. In other words, the oxidation state for which E° value is more negative (or less positive) will be more stable in the solution. U D YG M + 2H+ M2+ + H2 (g); 2M + 6H+ 2M3+ + 3H2(g) M(s) M(g) favourable only if H is negative. More negative is the value is of H , more favourable will be the formation of that cation from the metal. Thus, the oxidation state for which H value is more negative will be stable in Electrode potential for a Mn+/M half-cell is a measure of the tendency for the reaction, Mn+(aq) + ne– (i) These metals should liberate hydrogen from predicted from the electrode potential illustrate this, let us consider the following, M+(aq) +e–, will be the solution. The negative values of E° for the first series of transition elements (except for Cu2+/ Cu ) indicate that, dilute acids i.e., the reactions, 60 Electrode reaction Sc 3+ The reaction, M(s) E3 Ion The H represents the enthalpy change required to bring the solid metal M to the monovalent ion in aqueous medium, M+(aq). ID Elements 837 When an elements exists in more than one oxidation states, the standard electrode potential (E°) values can be used in the predicting the relative stabilities of different oxidation states in aqueous solutions. The following rule is found useful. The oxidation state of a cation for which H (H sub lE H hyd ) or E° is more negative (for less positive) will be more stable. (9) Formation of coloured ions : Most of the compound of the transition elements are coloured in the solid state and /or in the solution phase. The compounds of transition metals are coloured due to the presence of unpaired electrons in their d-orbitals. Explanation : In an isolated atom or ion of a transition elements, all the five d-orbitals are of the same energy (they are said to be regenerate). Under the influence of the combining anion (s), or electronrich molecules, the five d-orbitals split into two (or sometimes more than two) levels of different energies. The difference between the two energy levels depends upon the nature of the combining ions, but corresponds to the energy associated with the radiations in the 760 nm ). Typical splitting for visible region, 2 2( 380 2 dx -y dz dxyare dyzshown dzx octahedral and tetrahedral geometries in fig. H H1 H 2 H 3 H sub IE H hyd dorbitals dz2 dxy dyz dzx Octahexial field No field dx2-y2 Tetrahedral field The splitting of d-orbital energy levels in (a) an octahedral, (b) a tetrahedral, geometry. This 838 d and f-Block Elements 60 E The transition metal ions which have completely filled d-orbitals are colourless, as there are no vacant d-orbitals to permit promotion of the electrons. Therefore, Zn2+ (3d10), Cd2 + (4d10) and Hg2+(5d10) Sc 3+, Ti4+, Cu+ ions and Zn, Cd, Hg are colourless and diamagnetic. The transition metal ions which have completely empty d-orbitals are also colourless, Thus, Sc3+ and Ti4+.ions are colourless, unless a coloured anion is present in the compound. h E= h E3 Energy However, if radiations of all the wavelengths (or colours) except one are absorbed, then the colour of the substance will be the colour of the transmitted radiation. For example, if a substance absorbs all colours except green, then it would appear green to the eyes. Colours and the outer- electronic configurations of the some important ions of the first transition series elements are given bellow, Splitted dElectron gets orbitals promoted to the Promotion of d-electron to a higher higher level level by Ion Outer configurati on ID The transition metals in elements form or in the ionic form have one or more unpaired electrons. When visible light falls on the sample, the electrons from the lower energy level get promoted to a higher energy level due to the absorption of light of a characteristic wavelength (or colour). This wavelength (or colour) of the absorbed light depends upon the energy difference of the two levels. Rest of the light gets transmitted. The transmitted light has a colour complementary to the absorbed colour. Therefore, the compound or the solution appears to be of the complementary colour. U appear blue-green (blue-green is complementary colour to red). Hydrated Co2+ ions absorb radiation in the blue-green region, and therefore, appear red in sunlight. Relationship between the colour of the absorbed radiation and that of the transmitted light is given in table Relationship between the colours of the absorbed and transmitted light: the complementary colours. ST Colour of the Absorbed light Colour of the Transmitt ed light Absorbed light Transmitt ed light IR White Blue-green Red Red Blue-green Blue Orange Orange Blue Indigo Yellow Yellow Indigo Violet Yellowgreen Yellowgreen Violet UV White Green Purple of Colour of the ion Sc3+ 3d0 0 Colourles s Ti3+ 3d1 1 Purple 4+ Ti 0 3d 0 Colourles s V3+ 3d2 2 Green 3 3 Violet 5 3d 5 Light pink 3d4 4 Violet 6 3d 4 Green Fe3+ 3d5 5 Yellow Co3+ 3d7 3 Pink 2+ 8 2 Green U D YG For example, Cu (H 2 O)62 ions absorb red radiation, and Number unpaired electrons 3+ Cr Mn 2+ Mn3+ Fe 2+ Ni 3d 9 3d 1 Blue Cu+ 3d10 0 Colourles s Zn2+ 3d10 0 Colourles s Cu 2+ 3d (10) Magnetic properties : Most of the transition elements and their compounds show paramagnetism. The paramagnetism first increases in any transition element series, and then decreases. The maximum paramagnetism is seen around the middle of the series. The paramagnetism is described in Bohr Magneton (BM) units. The paramagnetic moments of some common ions of first transition series are given below in Table Explanation : A substance which is attracted by magnetic filed is called paramagnetic substance. The substances which are repelled by magnetic filed are, d and f-Block Elements s 4 S S 1 BM nn 2 BM where, S is the total spin (n s ) : n is the number of unpaired electrons and s is equal to ½ (representing the spin of an unpaired electron). From the equation given above, the magnetic moment ( s ) increases with an increase in the number of unpaired electrons. Magnetic moments of some ions of the 3d-series elements Outer configuratio n No. of unpaired electrons Magnetic moment (BM) Calculated observe d 3d0 0 0 0 3+ Ti 1 3d 1 1.73 1.75 Ti2+ 3d2 2 2.84 2.86 V2+ 3d3 3 3.87 3.86 Cr2+ 3d4 4 4.90 Mn2+ 4.80 3d5 5 5.92 2+ Fe 3d6 4 4.90 Co2+ 3d7 3 3.87 4.4-5.2 Ni2+ 3d8 2 2.84 2.9-3.4 2+ 9 1 1.73 1.4-2.2 0 0 0 Zn2+ 3d 3d10 5.95 5.0-5.5 D YG Cu (ii) These compounds show essentially the same chemical properties as the parent metals, but differ in physical properties such as density and hardness. Steel and cast iron are hard due to the formation of interstitial compound with carbon. Some nonstoichimetric compounds are, VSe0.98 (Vanadium selenide), Fe0.94O and titanium nitride. Explanation : Interstital compounds are hared and dense. This is because, the smaller atoms of lighter elements occupy the interstices in the lattice, leading to a more closely packed structure. Due to greater electronic interactions, the strength of the metallic bonds also increases. U Sc3+ (i) These are non-stoichiometric compounds and cannot be given definite formulae. ID Ion (12) Formation of interstitial compounds : Transition elements form a few interstitial compounds with elements having small atomic radii, such as hydrogen, boron, carbon and nitrogen. The small atoms of these elements get entrapped in between the void spaces (called interstices) of the metal lattice. Some characteristics of the interstitial compound are, 60 The magnetic moment of any transition element or its compound/ion is given by (assuming no contribution from the orbital magnetic moment). (ii) The availability to vacant inner d-orbitals of suitable energy. E3 called diamagnetic substances. Paramagnetism is due to the presence of unpaired electrons in atoms, ions or molecules. 839 U In d-obitals belonging to a particular energy level, there can be at the maximum five unpaired electrons in d5 cases. Therefore, paramagnetism in any transition series first increases, reaches a maximum value for d5 cases and then decreases thereafter. ST (11) Formation of complex ions : Transition metals and their ions show strong tendency for complex formation. The cations of transition elements (d-block elements) form complex ions with certain molecules containing one or more lone-pairs of electrons, viz., CO, NO, NH3 etc., or with anions such as, F–, Cl–, CN– etc. A few typical complex ions are, [Fe(CN )6 ] 4 , [Cu ( NH 3 ) 4 ] 2 , [Y (H 2 O)6 ] 2 , [ Ni(CO ) 4 ], [Co ( NH 3 )6 ] 3 [FeF6 ] 3 Explanation : This complex formation tendency is due to, (i) Small size and high nuclear charge of the transition metal cations. (13) Catalytic properties : Most of the transition metals and their compounds particularly oxides have good catalytic properties. Platinum, iron, vanadium pentoxide, nickel, etc., are important catalysts. Platinum is a general catalyst. Nickel powder is a good catalyst for hydrogenation of unsaturated organic compound such as, hydrogenation of oils some typical industrial catalysts are, (i) Vanadium pentoxide (V2O5) is used in the Contact process for the manufacture of sulphuric acid, (ii) Finely divided iron is used in the Haber’s process for the synthesis of ammonia. Explanation : Most transition elements act as good catalyst because of, (i) The presence of vacant d-orbitals. (ii) The tendency to exhibit variable oxidation states. (iii) The tendency to form reaction intermediates with reactants. (iv) The presence of defects in their crystal lattices. (14) Alloy formation : Transition metals form alloys among themselves. The alloys of transition metals are hard and high metals are high melting as compared to the host metal. Various steels are alloys of iron with metals such as chromium, vanadium, molybdenum, tungsten, manganese etc. 840 d and f-Block Elements (15) Chemical reactivity : The d-block elements (transition elements) have lesser tendency to react, i.e., these are less reactive as compared to s-block elements. Low reactivity of transition (b) Conversion of chromate into dichromate : Sodium chromate solution obtained in step (a) is treated with concentrated sulphuric acid when it is converted into sodium dichromate. 2 Na 2 CrO4 H 2 SO 4 Na 2 Cr2 O7 Na 2 SO 4 H 2 O sodium chromate sodium dichromate On concentration, the less soluble sodium sulphate, Na2SO4.10H2O crystallizes out. This is filtered hot and allowed to cool when sodium dichromate, Na2Cr2O7.2H2O, separates out on standing. (c) Concentration of sodium dichromate to potassium dichromate : Hot concentrated solution of sodium dichromate is treated with a calculated amount of potassium chloride. When potassium dichromate being less soluble crystallizes out on cooling. (i) Their high ionisation energies. (ii) Low heats of hydration of their ions. (iii) Their high heats of sublimation. Chromium containing compounds E3 Explanation : elements is due to, 4 FeCr2O4 16 NaOH 7O2 8 Na2CrO4 2Fe 2O3 8 H 2O 60 Explanation : The atomic radii of the transition elements in any series are not much different from each other. As a result, they can very easily replace each other in the lattice and form solid solutions over an appreciable composition range. Such solid solutions are called alloys. Na 2 Cr2 O7 2 KCl K 2 Cr2 O7 2 NaCl sod.dichromate Potassium dichromate, (K2Cr2O7) Physical properties (i) Potassium coloured crystals. ID Potassium dichromate is one of the most important compound of chromium, and also among dichromates. In this compound Cr is in the hexavalent (+6) state. dichromate forms orange-red (ii) It melts at 699 K. (iii) It is very stable in air (near room temperature) and is generally, used as a primary standard in the volumetric analysis. U Preparation : It can be prepared by any of the following methods, pot.dichromate 2 K 2 CrO 4 potassium chromate yellow D YG (i) From potassium chromate : Potassium dichromate can be obtained by adding a calculated amount of sulphuric acid to a saturated solution of potassium chromate. H 2 SO 4 K 2 Cr2 O 7 K 2 SO 4 H 2 O potassium dichromate orange K2Cr2O7 Crystals can be obtained by concentrating the solution and crystallisation. U (ii) Manufacture from chromite ore : K2Cr2O7 is generally manufactured from chromite ore (FeCr2O4). The process involves the following steps. ST (a) Preparation of sodium chromate : Finely powdered chromite ore is mixed with soda ash and quicklime. The mixture is then roasted in a reverberatory furnace in the presence of air. Yellow mass due to the formation of sodium chromate is obtained. 4 FeCr2O4 O2 2 Fe 2O3 4 Cr2O3 4 Cr2O3 8 Na2CO3 6O2 8 Na2CrO4 8 CO2 (g) 4 FeCr2 O4 8 Na 2 CO 3 7 O2 2 Fe 2 O3 8 CO 2 g 8 Na 2 CrO4 sodium chromate The yellow mass is extracted with water, and filtered. The filtrate contains sodium chromate. The reaction may also be carried out by using NaOH instead of Na2CO3. The reaction in that case is, (iv) It is soluble in water though the solubility is limited. Chemical properties (i) Action of heat : Potassium dichromate when heated strongly. Decomposes to give oxygen. 4 K 2 Cr2 O7 s 4 K 2 CrO4 (s) 2Cr2 O3 (s) 3 O 2 (ii) Action of acids (a) In cold, with concentrated H2SO4, red crystals of chromium trioxide separate out. K2Cr2O7 (aq) conc.H 2 SO 4 KHSO 4 (aq) 2CrO3 s H 2O On heating a dichromate-sulphuric acid mixture, oxygen gas is given out. 2K2Cr2O7 8 H 2 SO 4 2K2 SO 4 2Cr2 (SO 4 )3 8 H 2O 3O2 (b) With HCl, on heating chromic chloride is formed and Cl2 is liberated. K 2 Cr2 O7 aq 14 HCl aq 2CrCl3 aq 2 KCl aq 7 H 2 O 3Cl 2 g (iii) Action of alkalies : With alkalies, it gives chromates. For example, with KOH, K 2 Cr2 O4 2 KOH 2 K 2 CrO4 H 2 O orange yellow On acidifying, the colour again changes to orangered owing to the formation of dichromate. d and f-Block Elements 841 2 K2CrO4 H 2 SO 4 K2Cr2O7 K2 SO 4 H 2O K2Cr2O7 H 2SO4 K2SO4 Cr2 SO4 3 4 H 2O 3O Actually, in dichromate solution, the Cr2 O72 ions 2 KI H 2 O O 2 KOH I2 ] 3 2 KOH H 2 SO 4 K 2 SO 4 2 H 2 O] 3 ions. K2 Cr2 O7 7 H 2 SO 4 6 KI 4 K2 SO 4 Cr2 SO 4 3 3 I2 7 H 2 O Cr2 O72 H 2 O ⇌ 2CrO42 2 H (iv) Oxidising nature : In neutral or in acidic solution, potassium dichromate acts as an excellent oxidising agent, and Cr2 O72 gets reduced to Cr3+. The of the reducing agents such as, Fe2+. It oxidises, Ionic equation 6 Fe sulphates H 2 S O H 2O S ] 3 K2Cr2O7 4 H 2 SO 4 3 H 2 S K2 SO 4 Cr2 SO 4 3 7 H 2O 3S 3 and (v) Formation of insoluble chromates : With soluble salts of lead, barium etc., potassium dichromate gives insoluble chromates. Lead chromate is an important yellow pigment. 2Pb NO3 2 K2Cr2O7 H 2O 2 PbCrO4 2KNO 3 2 HNO3 7 H 2O arsenites to D YG to K2Cr2O7 4 H 2 SO 4 K2 SO 4 Cr2 (SO 4 )3 4 H 2O 3O U K 2 Cr2 O7 6 FeSO 4 7 H 2 SO 4 K 2 SO 4 Cr2 SO 4 3 3 Fe2 SO 4 3 7 H 2 O (b) Sulphites arsenates. K2Cr2O7 4 H 2SO4 K2SO4 Cr2 SO4 3 4 H 2O 3O (vi) Chromyl chloride test : When potassium dichromate is heated with conc. H2SO4 in the presence of a soluble chloride salt, the orange-red vapours of chromyl chloride (CrO2Cl2) are formed. heat K 2 Cr2 O7 4 NaCl 6 H 2 SO 4 2 KHSO 4 4 NaHSO 4 2CrO2 Cl 2 chromyl chloride orange red vapours Na 2 SO 3 [O] Na 2 SO 4 ] 3 K2Cr2O7 4 H 2SO4 3 Na 2 SO3 K2SO4 Cr2 SO4 3 3 Na 2SO4 4 H 2O Ionic equation U Cr2 O72 8 H 3 SO 32 2Cr 3 3 SO 42 4 H 2 O Cr2 O72 8 H 3 AsO 33 2Cr 3 3 AsO 43 4 H 2 O ST (ii) In chrome tanning (iii) The raw meterial for preparing large number of chromium compounds 2HX O H 2O X 2 ] 3 K2Cr2O7 4 H 2 SO 4 6 HX K2 SO 4 Cr2 SO 4 3 7 H 2O 3 X 2 where, X may be Cl, Br, I. equation 8 H 6 HX 2Cr (d) Iodides to iodine 3 3 X 2 7 H 2O H 2 CrO4 Chromic acid. (yellow solution ) (i) An oxidising agent K2Cr2O7 4 H 2 SO 4 K2 SO 4 Cr2 SO 4 3 4 H 2O 3O CrO2 Cl 2 2 H 2 O 2 HCl Uses : Potassium dichromate is used as, (c) Hydrogen halides to halogens. Cr2 O72 Chromyl chloride vapours when passed through water give yellow-coloured solution containing chromic acid. Chromyl chloride test can be used for the detection of chloride ion is any mixture. Similarly, arsenites are oxidised to arsenates. Ionic 7 H 2 O 3 I2 ID 2 FeSO4 H 2SO4 O Fe2 SO4 3 H 2O 3 2Cr : Cr2 O72 8 H 3 H 2 S 2Cr 3 3 S 7 H 2 O K2CrO7 4 H 2SO4 K2SO4 Cr2 SO4 3 4 H 2O 3O 14 H 6 Fe 14 H 6 I 2Cr 3 Ionic equation (a) Ferrous salts to ferric salts 3 (e) It oxidises H2S to S. This indicates that dichromate ion is a fairly strong oxidising agent, especially in strongly acidic solutions. That is why potassium dichromate is widely used as an oxidising agent, for quantitative estimation 2 equation 60 Cr2 O72 14 H 6 e 2Cr 3 7 H 2 O is +1.31V. Cr2 O72 Thus, when KI is added to an acidified solution of K2Cr2O7 iodine gets liberated. standard electrode potential for the reaction, Cr2 O72 Ionic E3 are in equilibrium with CrO42 : (iv) Primary standard in the volumetric analysis. Structures of Chromate and Dichromate Ions Chromates and dichromates are the salts of chromic acid (H2CrO4). In solution, these ions exist in equilibrium with each other. Chromate ion has four oxygen atoms arranged tetrahedrally around Cr atom. (see Fig). Dichromate ion involves a Cr–O–Cr bond as shown in Fig. 842 d and f-Block Elements O 180 pm Cr O 131° Cr O 161 O O O O O pm Chromate ion Dichromate The structure of chromate (CrO2–ion 4) and dichromate (Cr2O72–) ions O At anode: 2 MnO 42 2 MnO 4 2e green At cathode: 2 H 2e H 2 g The purple solution containing KMnO4 is evaporated under controlled condition to get crystalline sample of potassium permanganate. Manganese containing compound Potassium Permanganate, (KMnO4) Potassium permanganate is a salt of an unstable acid HMnO4 (permanganic acid). The Mn is an +7 state in this compound. Preparation : Potassium permanganate obtained from pyrolusite as follows. is K2 MnO 3 potassium manganite 4 H 2O] 2 2 MnO 2 4 KOH O2 2 K 2 MnO 4 2 H 2O potassium manganate pyrolusite dark green mass D YG fused Oxidation of potassium manganate to potassium permanganate : The potassium manganate so obtained is oxidised to potassium permanganate by either of the following methods. U By chemical method : The fused dark-green mass is extracted with a small quantity of water. The filtrate is warmed and treated with a current of ozone, chlorine or carbon dioxide. Potassium manganate gets oxidised to potassium permanganate and the hydrated manganese dioxide precipitates out. The reactions taking place are, When CO2 is passed 2 H 2O ST 3 K 2 MnO 4 potassium manganate KMnO4 crystallizes as dark purple crystals with greenish luster (m.p. 523 K). It is soluble in water to an extent of 6.5g per 100g at room temperature. The aqueous solution of KMnO4 has a purple colour. Chemical properties : Some important chemical reactions of KMnO4 are given below, Action of heat : KMnO4 is stable at room temperature, but decomposes to give oxygen at higher temperatures. heat 2 KMnO 4 s K 2 MnO 4 s MnO 2 O 2 g Oxidising actions : KMnO4 is a powerful agent in neutral, acidic and alkaline media. The nature of reaction is different in each medium. The oxidising U 2 K2 MnO 3 O2 2 K2 MnO 4 2 H 2O Physical properties ID Conversion of pyrolusite to potassium manganate : When manganese dioxide is fused with potassium hydroxide in the presence of air or an oxidising agent such as potassium nitrate or chlorate, potassium manganate is formed, possibly via potassium manganite. fused MnO 2 2 KOH purple 60 Cr Electrolytic method : Presently, potassium manganate (K2MnO4) is oxidised electrolytically. The electrode reactions are, 2– O E3 2– O 2 KMnO 4 potassium permangana te MnO 2 4 KOH 2CO 2 4 KOH 2 K2CO3 2 H 2O When chlorine or ozone is passed 2 K2 MnO 4 Cl2 2 KMnO 4 2 KCl 2 K2 MnO 4 O3 H 2O 2 KMnO 4 2 KOH O2 g The purple solution so obtained is concentrated and dark purple, needle-like crystals having metallic lustre are obtained. character of KMnO4 (to be more specific, of MnO 4 ) is indicated by high positive reduction potentials for the following reactions. Acidic medium MnO 4 8 H 5 e ⇌ Mn 2 4 H 2 O E o 1.51 V Alkaline medium MnO 4 2 H 2 O 3 e ⇌ MnO 2 4 OH E o 1. 23 V In strongly alkaline solutions and with excess of MnO 4 , the reaction is MnO 4 e ⇌ MnO 42 E o 0.56 V There are a large number of oxidation-reduction reactions involved in the chemistry of manganese compounds. Some typical reactions are, In the presence of excess of reducing agent in acidic solutions permanganate ion gets reduced to manganous ion, e.g., 5 Fe 2 MnO 4 8 H 5 Fe 3 Mn 2 4 H 2 O An excess of reducing agent in alkaline solution reduces permanganate ion only to manganese dioxide e.g., 3 NO 2 MnO 4 2OH 3 NO 3 MnO 2 H 2 O d and f-Block Elements In faintly acidic and neutral solutions, manganous ion is oxidised to manganese oxidised to manganese dioxide by permanganate. In strongly basic solutions, permangante oxidises manganese dioxide to manganate ion. MnO 2 2 MnO 4 4 OH 3 MnO 42 2 H 2 O 2 KMnO 4 H 2O 2 KOH 2 MnO 2 3O 2 KOH H 2 SO 4 K2 SO 4 2 H 2O 2KMnO 4 3 MnSO 4 2H 2O 5 MnO 2 K2 SO 4 2H 2 SO 4 Ionic equation In acidic medium, KMnO4 oxidises, 2 FeSO 4 H 2 SO 4 O Fe 2 SO 4 3 H 2O] 5 2KMnO 4 8 H 2 SO 4 10 FeSO 4 K2 SO 4 2MnSO 4 5 Fe 2 SO 4 3 8 H 2 O Ionic equation Ammonia to nitrogen 2 KMnO 4 H 2O 2 MnO 2 2 KOH 3O 2 NH 3 3O N 2 g 3 H 2O 2 KMnO 4 2 NH 3 2 MnO 2 2 KOH 2 H 2O N 2 g E3 2KMnO 4 3 H 2 SO 4 K2 SO 4 2 MnSO 4 3 H 2O 5O 60 2 MnO 4 3 Mn 2 2 H 2 O 5 MnO 2 4 H Ferrous salts to ferric salts Uses : KMnO4 is used, 2 MnO 4 16 H 10 Fe 2 2 Mn 2 10 Fe 3 8 H 2 O (i) As an oxidising agent. (ii) As a disinfectant against disease-causing germs. (iii) For sterilizing wells of drinking water. (iv) In volumetric estimation of ferrous salts, oxalic acid etc. (v) Dilute alkaline KMnO 4 solution known as Baeyer’s reagent. ID The reaction forms the basis of volumetric estimation of Fe2+ in any solution by KMnO4. Oxalic acid to carbon dioxide 3 H 2 O 5 O 4 Manganous sulphate (MnSO4) to manganese dioxide (MnO2) MnSO 4 H 2O O MnO 2 H 2 SO 4 3 2 MnO 4 3 Mn 2 2 H 2 O 5 MnO 2 4 H 2 KMnO 4 3 H 2 SO 4 K 2 SO 4 2 MnSO COOH 2 O 2CO2 H2O] 5 Structure of Permanganate Ion (MnO4–) : Mn in MnO4– is in +7 oxidation state. Mn7+ exhibits sp3 hybridisation in this ion. The structure of MnO4– is, shown in fig. U 2 KMnO4 3 H 2 SO4 5COOH 2 K2 SO4 2 MnSO4 10CO 2 8 H 2O Ionic equation O D YG 2 MnO 4 6 H 5 COOH 2 2 Mn 2 10 CO 2 8 H 2 O Sulphites to sulphates 2 KMnO 4 3 H 2 SO 4 K 2 SO 4 2 MnSO 4 O 3 H 2 O 5 O 4 Mn O– O Strucutre of MnO4– ion Na 2 SO 3 O Na 2 SO 4 ] 5 2 KMnO 4 3 H 2 SO 4 5 Na 2 SO 3 K 2 SO 4 2 MnSO 843 5 Na 2 SO 4 3 H 2 O Ionic equation 2 MnO 4 6 H 5 SO 32 2 Mn 2 5 SO 42 3 H 2 O U Iodides to iodine in acidic medium Iron and its Compounds (1) Ores of iron : Haematite Fe 2O3 , Magnetite 2 KI H 2O O I2 2 KOH 5 Copper pyrities (CuFeS 2 ) etc. ST 2 KMnO 4 3 H 2 SO 4 K2 SO 4 2 MnSO 4 3 H 2O 5O (Fe 3O4 ), 2 KOH H 2 SO 4 K2 SO 4 2 H 2O ] 5 2KMnO 4 8 H 2 SO 4 10 KI 6 K2 SO 4 2MnSO 4 5 I2 8 H 2O Ionic equation 2 MnO 4 16 H 10 I 2 Mn 2 5 I2 8 H 2 O Hydrogen peroxide to oxygen 2 KMnO 4 3 H 2 SO 4 K2 SO 4 2 MnSO 4 3 H 2O 5O Limonite (Fe 2O3.3 H 2O) , Iron pyrites (FeS 2 ), (2) Extraction : Cast iron is extracted from its oxides by reduction with carbon and carbon monoxide in a blast furnace to give pig iron. Roasting : Ferrous oxide convert into ferric oxide. Fe 2O3. 3 H 2O Fe 2O3 3 H 2O ; 2 FeCO 3 2 FeO 2CO2 4 FeO O2 2 Fe 2O3 H 2 O2 O H 2 O O2 5 Smelting : Reduction of roasted ore of ferric oxide carried out in a blast furnace. 2KMnO 4 3 H 2 SO 4 5 H 2O2 K2 SO 4 2MnSO 4 8 H 2O 5O2 (i) The reduction of ferric oxide is done by carbon and carbon monoxide (between 1473k to 1873k) 844 d and f-Block Elements 2C O2 2CO (ii) Fe 2O3 3CO 673 K 2 Fe 3CO2. It is a reversible and exothermic reaction. Hence according to Lechatelier principle more iron will be produced in the furnace at lower temp. Fe 2O3 CO 2 FeO CO 2 (it is not reversible ) (d) Case-hardening : It is a process of giving a thin coating of hardened steel to wrought iron or to a strong and flexible mild steel by heating it in contact with charcoal followed by quenching in oil. (e) Nitriding : It is a process of heating steels at about 700 o C in an atmosphere of ammonia. This process imparts a hard coating of iron nitride on the surface of steel. 1073 K Fe CO (iii) FeO C endothermi c reaction Properties of steel : The properties of steel depend upon its carbon contents. With the increase in carbon content, the hardness of steel increases while its ductility decreases. Varieties of iron : The three commercial varieties of iron differ in their carbon contents. These are; (i) Low carbon or soft steels contain carbon upto 0.25%. (1) Cast iron or Pig-iron : It is most impure form of iron and contains highest proportion of carbon (2.5– 4%). (ii) Medium carbon steels or mild steels contain 0.25–0.5% carbon. E3 (iii) High carbon or hard steels contains 0.1 – 1.5 percent carbon. (iv) Alloy steels or special steels are alloys of steel with Ni, Cr, Co , W, Mn, V etc., For example ID (2) Wrought iron or Malleable iron : It is the purest form of iron and contains minimum amount of carbon (0.12–0.25%). 60 The gases leaving at the top of the furnace contain up to 28% CO and are burnt in cowper's stove to preheat the air for blast (a) Stainless steel (Fe = 73%, Cr = 18%, Ni = 8% + C) is resistant to corrosion and is used for making ornamental pieces, cutlery etc. (b) Invar (Fe = 64%, Ni = 36%) has small coefficient of expansion and is used for making metre scales, pendulum rods and watches. U (3) Steel : It is the most important form of iron and finds extensive applications. Its carbons content (Impurity) is mid-way between cast iron and wrought iron. It contains 0.2–1.5% carbon. Steels containing 0.2– 0.5% of carbon are known as mild steels, while those containing 0.5–1.5% carbon are known as hard steels. D YG Steel is generally manufactured from cast iron by three processes, viz, (i) Bessemer Process which involves the use of a large pear-shaped furnace (vessel) called Bessemer converter, (ii) L.D. process and (iii) open hearth process, Spiegeleisen (an alloy of Fe, Mn and C) is added during manufacture of steel. U Heat treatment of steels : Heat treatment of steel may be defined as the process of carefully heating the steel to high temperature followed by cooling to the room temperature under controlled conditions. Heat treatment of steel is done for the following two purposes, (c) Manganese steel (Fe = 86%, Mn 13% + carbon) is very hard and resistant to wear and hence is used for making rock drills, safes etc. (d) Tungsten steel (Fe = 94%, W = 5% + carbon) is quite hard and is used for making high speed cutting tools. (e) Permalloy (Fe = 21%, Ni = 78% + carbon) is strongly magnetised by electric current but loses magnetism when current is cut off. It is used for making electromagnets, ocean cables etc. Properties of iron (1) Dry or moist air has no action on pure iron but impure iron when exposed to moist air is covered with a layer of rust Fe 2 O3 Fe(OH )3. However, finely divided (ii) To remove some undesirable properties or gases like entrapped gases, internal stresses and strains. The various methods of heat treatment are, pure iron burns in air or oxygen forming ST (i) To develop certain special properties like hardness, strength, ductility etc. without changing the chemical composition. (magnetic oxide of iron). 3 Fe 2O2 Fe 3 O4 (a) Annealing : It is a process of heating steel to redness followed by slow cooling. (b) Quenching or hardening : It is a process of heating steel to redness followed by sudden cooling by plunging the red hot steel into water or oil. (c) Tempering : It is a process of heating the hardened or quenched steel to a temperature much below redness (473–623K) followed by slow cooling. Fe 3 O4 (2) Iron decomposes steam at red heat Red heat 3 Fe 4 H 2 O Fe 3 O 4 4 H 2 Steam (3) Action of acids : Iron reacts with dil. HCl and dil. H 2 SO 4 liberating hydrogen. with hot conc. H 2 SO 4 , it gives SO 2 , with dil. HNO 3 , it gives NH 4 NO 3 and moderately conc. HNO 3 reacts with iron forming NO 2. d and f-Block Elements (c) Due to oxidising nature Fe 3 ions FeCl 3 is Cold conc. HNO 3 makes iron passive due to the deposit of a thin layer of iron oxide (Fe 3 O4 ) on the 845 used in etching metals such as copper surface. Hot conc. HNO 3 reacts with iron liberating NO. 2 Fe 3 Cu 2 Fe 2 Cu 2 (aq) (d) In vapour state FeCl 3 exists as a dimer, Fe 2Cl6 Fe 4 HNO3 (hot conc. ) Fe(NO3 )3 NO 2H2O Cl (4) Iron does not react with alkalies. (5) It displaces less electropositive metals (e.g., Cu, Ag etc.) from their salts CuSO 4 Fe FeSO 4 Cu Cl Cl (e) FeCl 3 is used as stypic to stop bleeding from a cut. (4) Ferrous sulphate, FeSO 4 , 7 H 2 O (Green vitriol) : It is prepared as follow , Fe H2SO 4 FeSO 4 H2 (i) One pressure to moist air crystals become brownish due to oxidation by air. 4 FeSO 4 2H2O O2 4 Fe(OH )SO 4 (ii) On heating, crystals become anhydrous and on strong heating it decomposes to Fe 2 O3 , SO 2 and SO 3. ID magnetic oxide or load stone). (i) Ferrous oxide, FeO : It is a black powder, basic in nature and reacts with dilute acids to give ferrous salts. FeO H 2 SO 4 FeSO 4 H 2O ; It is used in glass Cl 60 (7) Iron does not form amalgam with Hg. (8) Iron is the most abundant and most widely used transition metal. Compounds of iron (1) Oxides of Iron : Iron forms three oxides FeO , Fe 2O3 (Haematite), Fe 3 O4 (magnetite also called Cl Fe E3 (6) Finely divided iron combines with CO forming penta carbonyl Fe 5CO Fe(CO)5 Cl Fe Strong 2 FeSO 4 Fe 2 O3 SO 2 SO 3 heating (iii) It can reduce acidic solution of KMnO 4 and U industry to impart green colour to glass. (ii) Ferric oxide Fe 2O3 : It is a reddish brown heat FeSO 4.7 H 2 O FeSO 4 7 H 2 O D YG powder, not affected by air or water; amphoteric in nature and reacts both with acids and alkalis giving salts. It can be reduced to iron by heating with C or CO. Fe2O3 3C 2 Fe 3CO ; Fe 2O3 3CO 2 Fe 3CO2 It is used as red pigment to impart red colour to external walls and as a polishing powder by jewellers. (iii) Ferrosoferricoxide Fe3O4 (FeO. Fe 2O3 ) : It is more stable than FeO and Fe 2O3 , magnetic in nature U and dissolves in acids giving a mixture of iron (II) and iron (III) salts. Fe3O4 4 H 2 SO 4 (dil) FeSO 4 Fe2 (SO 4 )3 4 H 2O (2) Ferrous sulphide FeS : It is prepared by heating iron filing with sulphur. With dilute H 2 SO 4 , it FeS H 2 SO 4 (dil) FeSO 4 H 2 S ST gives H 2 S. (3) Ferric chloride FeCl 3 : (i) preparation : It is prepared by treating Fe(OH )3 with HCl Fe(OH )3 3 HCl FeCl 3 3 H 2O FeCl 3 forms reddish-black deliquescent crystals. (b) FeCl 3 is hygroscopic and dissolves in H 2 O giving brown acidic solution due to formation of HCl FeCl 3 3 H 2O Fe(OH )3 3 HCl (Brown) (iv) It is generally used in double salt with ammonium sulphate. (NH 4 )2 SO 4 FeSO 4 6 H 2O FeSO 4.( NH 4 )2 SO 4.6 H 2O Mohr' s salt Mohr’s salt is resistant to atmospheric oxidation. (v) It is used in the ring test for nitrate ions where it gives brown coloured ring of compound FeSO 4. NO. FeSO 4 NO FeSO 4. NO (vi) FeSO 4 is used in manufacture of blue black ink. (vii) FeSO 4 H 2O2 is known as a name of Fenton’s reagent. (5) Mohr's salt FeSO 4. (NH 4 )2 SO 4. 6 H 2O : It is a double salt and is prepared by crystallising a solution containing equivalent amounts of FeSO 4.7 H2O and (NH 4 )2 SO 4. It may be noted that Mohr’s salt contains The solution on evaporation give yellow crystals of FeCl 3. 6 H2O (ii) Properties : (a) Anhydrous K 2 Cr2 O7 only Fe 2 ions without any trace of Fe 3 ions. In contrast FeSO 4.7 H 2 O always contains some Fe 3 ions due to aerial oxidation of Fe 2 ions. Mohr salt is, therefore, used as a primary standard in volumetric analysis since a standard solution of Fe 2 ions can be obtained directly by weighing a known amount of the Mohr salt. 846 d and f-Block Elements It acts as a reducing agent and as such reduces acidified KMnO 4 and K 2 Cr2 O7 solutions. O2 Below 1370 K 4 Cu O2 2Cu 2O 4 CuO MnO 4 5 Fe 2 8 H 5 Fe 3 Mn 2 4 H 2 O 14 H 6 Fe (From mohrs salt) 3 2Cr 3 (Red) 7 H 2O High temp. CuO Cu Cu 2 O Copper and its Compounds (1) Ores : Copper pyrites (chalcopyrite) CuFeS 2 , Cuprite (ruby copper) Cu 2 O, Copper glance (Cu 2 S ) , Malachite [Cu(OH )2. CuCO 3 ], Azurite [Cu(OH )2. 2CuCO 3 ] (2) Extraction : Most of the copper (about 75%) is extracted from its sulphide ore, copper pyrites. Concentration of ore : Froth floatation process. Roasting : Main reaction : 2CuFeS 2 O2 Cu 2 S 2 FeS SO 2. Side reaction : 2Cu 2S 3O2 2Cu 2O 2SO 2 (3) Action of acids. Non oxidising dil. acids such as HCl, H2SO 4 have no action on copper. However, copper dissolves in these acids in presence of air. 1 Cu 2 HCl O 2 (air) CuCl 2 H 2 O 2 With dil. HNO 3 , Cu liberates NO (nitric oxide) 3Cu 8 HNO3 3Cu(NO3 )2 2 NO 4 H2O With conc. HNO 3 , copper gives NO 2 Cu 4 HNO 3 Cu(NO 3 )2 2 NO 2 2 H 2 O With hot conc. H 2 SO 4 , copper gives SO 2 Cu 2H2SO 4 CuSO 4 SO 2 2H2O 2 FeS 3O2 2 FeO 2SO 2. Compounds of Copper (1) Halides of copper : Copper (II) chloride, CuCl 2 is prepared by passing chlorine over heated Smelting : FeO SiO 2 FeSiO 3 (slag) ID Cu 2O FeS FeO Cu 2 S Above 1370 K (Black) 60 6 Fe 2 E3 Cr2 O72 further heating. If the temperature is too high, cupric oxide changes back to cuprous oxide The mixture of copper and iron sulphides melt together to form 'matte' (Cu 2S FeS ) and the slag floats brown but changes first to green and then to blue on dilution. On heating, it disproportionates to copper (I) chloride and chlorine D YG U on its surface. Conversion of matte into Blister copper (Bessemerisation) : Silica is added to matte and a hot blast of air is passed FeO SiO 2 FeSiO 3 (slag). Slag is copper. Concentrated aqueous solution of CuCl 2 is dark removed. By this time most of iron sulphide is removed. Cu 2S 2Cu 2O 6Cu SO 2 U Blister copper : Which contain about 98% pure copper and 2% impurities (Ag, Au, Ni, Zn etc.) Properties of copper : It has reddish brown colour. It is highly malleable and ductile. It has high electrical conductivity and high thermal conductivity. Copper is second most useful metal (first being iron). It undergoes displacement reactions with lesser reactive metals e.g. with Ag. It can displace Ag from AgNO 3. The ST finally divided Ag so obtained is black in colour. Copper shows oxidation states of +1 and +2. Whereas copper (I) salts are colourless, copper (II) salts are blue in colour. Cu (I) salts are less stable and hence are easily oxidised to Cu (II) salts (2Cu Cu 2 Cu ). This reaction is called disproportionation. (1) In presence of atmospheric CO 2 and moisture, copper gets covered with a green layer of basic copper carbonate (green layer) which protects the rest of the metal from further acton. Cu O2 CO 2 H 2 O Cu (OH )2 CuCO 3 (green lay er) (2) In presence of oxygen or air, copper when heated to redness (below 1370K) first forms red cuprous oxide which changes to black cupric oxide on Heat 2CuCl 2 2CuCl Cl 2 It is used as a catalyst in the Daecon’s process for the manufacture of chlorine. Copper (I) chloride, CuCl is a white solid insoluble in water. It is obtained by boiling a solution of CuCl 2 with excess of copper turnings and conc. HCl. HCl CuCl 2 Cu 2CuCl It dissolves in conc. HCl due to the formation of complex H[CuCl 2 ] CuCl HCl H[CuCl 2 ] It is used as a catalyst alongwith NH 4 Cl in the preparation of synthetic rubber. (2) Cuprous oxide Cu 2 O : It is a reddish brown powder insoluble in water but soluble in ammonia solution, where it forms diammine copper (I) ion. Cu 2 NH 3 [Cu ( NH 3 )2 ]. It is used to impart red colour to glass in glass industry. (3) Cupric oxide CuO : It is dark black, hygroscopic powder which is reduced to Cu by hydrogen, CO etc. It is used to impart light blue colour to glass. It is prepared by heating copper nitrate. 2Cu ( NO 3 )2 2CuO 4 NO 2 O2 d and f-Block Elements (4) Copper sulphate CuSO 4. 5 H 2 O (Blue vitriol) : It is prepared by action of dil H 2 SO 4 on copper scrap in presence of air. 2Cu 2 H 2 SO 4 O2 CuSO 4 2 H 2 O (air ) 1000 K CuO SO CuSO 4 3 (ii) It gives a deep blue solution of tetrammine copper (II) sulphate with NH 4 OH. Cu 2 SO 4 4 NH 4 OH , [Cu(NH 3 )4 ]SO 4 4 H 2O Blue colour (iii) With KCN it first gives yellow precipitate of CuCN which decomposes of give Cu 2 (CN )2. Cu 2 (CN )2 4 KI 2CuSO 4 2 K2 SO 4 Cu 2 I2 I2 White ppt. They are used as green and blue painter’s pigments under the name ‘malachite green’ and azurite blue’. Silver and its Compounds (1) Ores : Argentite (silver glance) Ag 2 S , Horn silver (AgCl ), Ruby silver (Pyrargyrite) 3 Ag 2S. Sb 2S 3. (2) Extraction : Cyanide process or Mac ArthusForrest cyanide process : This method depends on the fact that silver, its sulphide or chloride, forms soluble complex with alkali cyanides in the silver. This implies that silver compounds will dissolve in solution of alkali cyanides in the presence of blast of air. 4 Ag 8 NaCN 2 H 2 O O 2 ⇌ 4 Na[ Ag (CN )2 ] 4 NaOH air U Cu 2 [Fe(CN )6 ] 2 K 2 SO 4 Reddish brown ppt. D YG 2CuSO 4 K 4 [Fe(CN )6 ] On heating, both decompose to give black cupric oxide, water and CO 2. or 4 Ag 8 CN 2 H 2 O O 2 ⇌ 4[ Ag (CN )2 ] 4 OH (v) With K4 [Fe(CN )6 ], CuSO 4 gives a reddish brown ppt. of Cu 2 [Fe(CN )6 ] 3CaSO 4 CO2 ID dissolves in excess of KCN to give K3 [Cu(CN )4 ] Azurite 60 and SO 3. 423 K 3 CuSO 4 3 CaCO 3 H 2 O 2CuCO 3.Cu (OH )2 E3 CuSO 4. 5 H 2O CuSO 4 5 H 2O Blue White At about 1000 K, CuSO 4 decomposes to give CuO 503 K (iv) With KI it gives white ppt. of Cu 2 I2 2CaSO 4 CO 2 At lower temperature azurite is formed (i) On heating this blue salt becomes white due to loss of water of crystallization. 2CuSO 4 4 KCN Cu 2 (CN )2 2 K2SO 4 (CN )2 847 Uses : For electroplating and electrorefining of copper. As a mordant in dyeing. For making Bordeaux mixture (11 parts lime as milk of lime + 16 parts copper sulphate in 1,000 parts of water). It is an excellent fungicide. For making green pigments containing copper carbonate and other compounds of copper. Like Verdigris which is Cu(CH 3COO )2 Cu(OH )2 i.e. basic U copper acetate and is used as a green pigment in paints. As a fungicide in starch paste for book binding work. ST (5) Cupric sulphide CuS : It is prepared as follows : Cu ( NO 3 )2 H 2 S CuS 2 HNO 3. Black ppt. (6) Basic copper carbonates : Because of lower solubility of the hydroxide, the normal carbonate does not exist. Two basic copper carbonates occur in nature viz malachite CuCO 3.Cu(OH )2 which has a fine green colour, and azurite, 2CuCO 3 , Cu(OH )2 which is deep blue in colour. Malachite is prepared by heating a mixture of CuSO 4 solution and limestone in a sealed tube at 423 – 443 K 423 - 443K 2CuSO 4 2CaCO 3 H 2 O CuCO 3 Cu (OH )2 Malachite Ag 2 S 4 NaCN ⇌ 2 Na[ Ag(CN )2 ] Na2S AgCl 2 NaCN ⇌ Na[ Ag(CN )2 ] NaCl. The reaction with the sulphide is reversible and accumulation of Na2S must be prevented. A free excess of air is continuously passed through the solution which oxidizes Na2S into sulphate and thiosulphate. 2 Na2 2O2 H2O Na2S 2O3 2 NaOH Na2 S 2O3 2 NaOH 2O2 2 Na2 SO 4 H 2O 2 Na[ Ag(CN )2 ] 4 NaOH Zn Na2 ZnO2 4 NaCN 2 H 2O 2 Ag (3) Extraction of Ag from argentiferrous lead (PbS + Ag2S)– Parke’s Process : It is based upon the following facts (i) Molten Zn and Pb are immiscible, zinc forms the upper layer (ii) Ag is more soluble in molten Zn (iii) Zn-Ag alloy solidifies earlier than molten Pb (IV) Zn being volatile can be separated from Ag by distillation. Ag is purified by cupellation. Properties of Silver: Silver is a white lustrous metal, best conductor of heat and electricity. Being soft, it is alloyed. The silver alloy used for making jewellery contain 80% Ag and 20% Cu. The composition of a silver alloy is expressed as its purity i.e. the amount of Ag present in 1000 parts of the alloy Ag does not react with dilute HCl or dil. H 2 SO 4 and aqua regia but reacts with dil. HNO 3 and conc. HNO 3 848 d and f-Block Elements forming NO and NO 2 respectively. Chlorine also reacts with Ag to form AgCl. dyes. like Cu Compounds of Silver: (1) Silver oxide ( Ag 2 O) : It is unstable and decomposes into Ag and O 2 on slow heating. 2 Ag 2O 4 Ag O2 (2) Silver halides (AgF, AgCl, AgBr and Agl) : Only AgF is soluble in H 2 O. AgCl is insoluble in H 2 O but dissolves in NH 4 OH , Na 2 S 2 O3 and KCN solutions. AgBr is partly soluble whereas Agl is completely insoluble in NH 4 OH. Except AgF , all the remaining three silver halides are photosensitive. AgCl 2 NH 4 OH [ Ag (NH 3 )2 ]Cl 2 H 2 O Diamine silver (I)chloride Sod. Dithiosulp hato argentate (I) The film is finally fixed by dipping in a solution of sodium thiosulphate or hypo which removes unchanged AgBr as complex ion. AgBr 2 Na 2 S 2 O3 Na 3 [ Ag (S 2 O3 )2 ] NaBr After taking a print of toned by dipping in a dilute impart a beautiful golden potassium chloro platinate D YG Above 485 K 2 AgNO 3 2 AgNO 2 O2 Red heat 2 AgNO 3 2 Ag 2 NO 2 O2 When treated with alkali, AgNO 3 Gold and its Compounds (1) Occurrence of gold : Gold is mainly found in native state either as vein gold, placer gold or alluvial gold. It is also present to a small extent in the combined state as sulphide, telluride and arsenosulphide. It is considered to be the king of metal. Some important ores of gold are: forms silver (i) Calaverite, AuTe2 (ii) Sylvanite, AuAgTe2 and dissolves to form 2 AgNO 3 2 NaOH Ag 2O 2 NaNO 3 H 2O 2 AgNO 3 2 NH 4 OH Ag 2O 2 NH 4 NO3 H 2O Ag 2 O 4 NH 4 OH 2[ Ag (NH 3 )2 ]OH 3 H 2 O U Diamine silver hy droxide reacts with iodine in two ways 6 AgNO 3 (excess) + 3l2 3 H 2 O AglO3 5 AgI 6 HNO3 ST 5 AgNO 3 3l2 (iii) Bismuth aurite, BiAu 2 (2) Extraction of gold : (i) Mac-Arthur-Forest Cyanide process : The powdered gold ore, after concentration by Froth-floatation process, is roasted to remove easily oxidisable impurities of tellurium, arsenic and sulphur. The roasted ore is then treated with a dilute solution of KCN in presence of atmospheric oxygen when gold dissolves due to the formation of an aurocyanide complex. (excess) 4 Au 8 KCN 2 H 2O O2 4 K[ Au (CN )2 ] 4 KOH Solution + 3 H 2O HIO3 5 Agl 5 HNO3 The metal is then extracted by adding zinc dust. In contact with organic matter (skin, cloth, paper etc.) AgNO 3 is reduced to metallic silver (black) 2 AgNO 3 H2O 2 Ag 2 HNO3 [O] oxidises organic matter gives AgNO 3 different different anions like etc. the photograph it is finally solution of gold chloride to colour or it is dipped in K2 PtCl 6 solution to get a U (3) Silver nitrate (AgNO3) : Silver nitrate (AgNO3) is called lunar caustic silver nitrate on heating above its m.p. (485 K) decomposes to silver nitrite but on heating to red heat gives silver. AgNO 3 C6 H 4 (OH )2 2 AgBr 2 Ag C6 H 4 O2 2 HBr Quinol Quinone AuCl 3 3 Ag 3 AgCl Au AgCl 2 Na 2 S 2 O3 Na3 [ Ag (S 2O3 )2 ] NaCl NH 4 OH The exposed film is developed. The developer used is an alkaline solution of hydroquinone or quinol which reduces some of the exposed AgBr to black silver. shining grey tinge. Pot. Dicy ano argentate (I) oxide which in case of 2 AgBr 2 Ag Br2 ID AgCl 2 KCN K[ Ag (CN )2 ] KCl exposure, AgBr is reduced to metallic silver. 60 Hot conc. H 2 SO 4 reacts with Ag forming SO 2 Photography : The photographic plate is coated with a colloidal gelatinised solution of AgBr. During E3 2 Ag Cl2 2 AgCl complex ion. AgNO 3 is used in the preparation of ink and hair coloured ppt. with Cl , Br , I , S 2 , S 2 O 32 , CrO42 , PO 43 2 K [ Au (CN )2 ] Zn K 2 [Zn(CN )4 ] 2 Au ppt. (ii) Plattner’s chlorine process : The roasted ore is moistened with water and placed in wooden vats with false perforated bottoms. It is saturated with current of chlorine, gold chloride thus formed is leached with water and the solution is treated with a reducing agent such as FeSO 4 or H 2 S to precipitate gold. d and f-Block Elements AuCl 3 3 FeSO 4 Au FeCl 3 Fe 2 (SO 4 )3 H 2S 2 AuCl 3 3 H 2 S 6 HCl 3 S 2 Au The impure gold thus obtained contains impurities of Ag and Cu. The removal of Ag and Cu from gold is called parting. This is done by heating impure gold with conc. H 2 SO 4 (or HNO3 ) when Ag and Cu dissolve leaving behind Au. Cu 2H2SO 4 CuSO 4 SO 2 2H2O 849 (2) Aurous sulphide, Au2S : It is prepared when is passed through an acidified solution of potassium aurocyanide, K[ Au(CN )2 ] 2 K[ Au(CN )2 ] H 2 S Au 2 S 2 KCN 2 HCN It is a dark brown solid, not attached by dilute mineral acids and hence is probably the most stable gold compound. Zinc and its Compounds of heat and electricity. (2) Extraction of zinc : Zinc blende, after concentration by Froth floatation process, is roasted in air to convert it into ZnO. In case of calamine, ore is calcined to get ZnO. The oxide thus obtained is mixed with crushed coke and heated at 1673 K in fire clay retorts (Belgian Process) when ZnO gets reduced to metallic zinc. Being volatile at this temperature, the metal distils over and is condensed leaving behind Cd, Pb and Fe as impurities. The crude metal is called spelter. The metal may be refined either by electrolysis or by fractional distillation. ID Pure gold is soft. It is alloyed with Ag or Cu for making jewellery. Purity of gold is expressed in terms of carats. Pure gold is 24 carats. Gold ’14 carats’ means that it is an alloy of gold which contains 14 parts by weight of pure gold and 10 parts of copper per 24 parts by weight of the alloy. Thus the percentage of gold in 100 14 58.3 %. ’14 carats” of gold is = 24 (1) Occurrence of zinc: Zinc does not occur in the native form since it is a reactive metal. The chief ores of zinc are (i) Zinc blende (ZnS) (ii) Calamine or zinc spar (ZnCO3) and (iii) Zincite (ZnO) 60 Properties of Gold: Gold is a yellow, soft and heavy metal. Gold and Ag are called noble metals since they are not attacked by atmospheric oxygen. However, Ag gets tarnished when exposed to air containing traces of H 2 S. Gold is malleable, ductile and a good conductor E3 2 Ag 2 H2SO 4 Ag 2SO 4 SO 2 2 H2O Properties of Zn : Zinc is more reactive than mercury. It is a good conductor of heat and electricity. Zinc readily combines with oxygen to form ZnO. Pure zinc does not react with non-oxidising acids (HCl or H 2 SO 4 ) but the impure metal reacts forming Zn 2 ions U D YG Most of the jewellery is made from 22 carat gold (91.66% pure gold). Gold is quite inert. It does not react with oxygen, water and acids but dissolves in aqua regia 3 HCl HNO 3 NOCl 2 H 2O 2Cl] 3 Au 3 Cl AuCl 3 ] 2 and evolving H 2 gas. Zn 2 HCl ZnCl 2 H 2 2 Au 9 HCl 3 HNO 3 2 AuCl 3 6 H 2 O 3 NOCl Auric chloride Nitrosyl chloride Oxidation states of gold: The principal oxidation states of gold are + 1 and + 3 though + 1 state is more stable than + 3. Compounds of gold Hot and conc. H 2 SO 4 attacks zinc liberating SO 2 gas Zn 2 H 2 SO 4 ZnSO 4 SO 2 2H 2O Zinc also reacts with both dilute (hot and cold) HNO3 and conc. HNO3 liberating nitrous oxide ( N 2 O) , ammonium nitrate K (NO 2 ) respectively. ST U (1) Auric chloride, AuCl3 : It is prepared by passing dry Cl 2 over finely divided gold powder at 573 573 K 2 Au 3Cl 2 2 AuCl 3 It is a red coloured crystalline solid soluble in water and decomposes on heating to give gold (I) chloride and Cl 2 Heat AuCl 3 AuCl Cl 2 It dissolves in conc. HCl forming chloroauric acid AuCl 3 HCl H[ AuCl 4 ] Chloroauric acid is used in photography for toning silver prints and as an antidote for snake poisoning. (NH 4 NO3 ) and nitrogen dioxide 4 Zn 10 HNO3 (warm, dilute) 4 Zn(NO3 )2 N 2O 5 H 2O 4 Zn 10 HNO 3 (coldvery dilute) 4 Zn(NO3 )2 NH 4 NO3 3 H 2 O Zn 4HNO3 (hot and conc.) Zn(NO3 )2 2 NO2 2 H 2O Zinc dissolves in hot concentrated NaOH forming the soluble sod. Zincate Zn 2 NaOH 2 H 2 O Na 2 [Zn(OH )4 ] H 2 or Zn 2 NaOH Na 2 ZnO 2 H 2 850 d and f-Block Elements (ii) Granulated zinc : It is prepared by pouring molten zinc into cold water. Both these varieties of zinc are used as reducing agents in laboratory. Compounds of zinc (1) Zinc oxide (Zinc white or Chinese white), ZnO : It is obtained by burning zinc in air or by heating zinc carbonate or zinc nitrate. 373 K 723 K ZnSO 4.7 H 2 O ZnSO 4.H 2 O 1073 K 2 ZnSO 4 2 ZnO 2 SO 2 O2 It is used to prepare lithopone (BaSO 4 ZnS ) , a white paint and also in galvanising iron. ZnSO 4 BaS ZnS BaSO 4 It is also used as an eye lotion. Mercury and its compounds (1) Occurrence and extraction of mercury : Heat 2 Zn O 2 2 ZnO Heat 2 Zn( NO 3 ) 2 2 ZnO 4 NO 2 O 2 (Basic) ZnO 2 NaOH Na 2 ZnO2 H 2O 273 873 K HgS O2 Hg SO 2 The mercury vapours thus obtained are condensed to give liquid metal. Hg thus obtained contains impurities of Zn, Sn and Pb. These are removed by treating the impure metal with dil HNO3 , mercurous nitrate, Sod.zincat e or ZnO 2 NaOH H 2 O (auto reduction). ID It is a white powder but becomes yellow on heating and again white on cooling. It is insoluble in water and is very light and hence commonly known as philosopher’s wool. It is amphoteric in nature. ZnO 2 HCl ZnCl 2 H 2 O E3 Cinnabar (HgS) is the only important ore of Hg. It is concentrated by froth floatation method and mercury is extracted from this ore by heating it in air at 773-873 K Heat ZnCO 3 ZnO CO 2 (Acidic) 373 K. At 723 K, it becomes anhydrous and on further heating, it decomposes. 60 (3) Special varieties of zinc. (i) Zinc dust : It is prepared by melting zinc and then atomising it with a blast of air. Na 2 [ Zn(OH )4 ] thus formed react with metals present as impurities forming their nitrates which pass U Sod. tetrahy droxozincate (II) Hg 2 (NO 3 )2 It is reduced both by carbon and H 2 and is used D YG as a white paint ZnO C Zn CO ; ZnO H2 Zn H2O (2) Zinc chloride, ZnCl2 : It is obtained when Zn metal, ZnO or ZnCO3 is treated with dil. HCl. It crystallizes as ZnCl 2.2 H 2O and becomes anhydrous on heating. ZnCl 2 is highly deliquescent and is highly soluble in H 2O and also readily dissolves in organic U solvents like acetone, alcohol, ether etc. its aqueous solution is acidic due to hydrolysis. ZnCl 2 H 2O Zn(OH )Cl HCl Anhydrous ZnCl 2 is used as a Lewis acid catalyst ST in organic reactions. Mixed with moist zinc oxide, it is used for filling teeth and its solution is used for preserving timber. Anhydrous ZnCl 2 used as a Lucas reagent with conc. HCl. (3) Zinc sulphide, ZnS : It is a white solid. It is soluble in dil. HCl and thus does not get precipitated by H 2 S in the acidic medium. ZnS 2 HCl ZnCl 2 H 2 S. It is a constituent of lithopone (ZnS BaSO 4 ) (4) Zinc sulphate, ZnSO4.7H2O : It is commonly known as white vitriol and is obtained by the action of dil. H 2 SO 4 on zinc metal, ZnO or ZnCO3. On heating, it first loses six molecules of water of crystallization at into solution leaving behind pure mercury. However, it is best purified by distillation under reduced pressure. warm 6 Hg 8 HNO 3 (dil.) 3 Hg2 (NO3 )2 4 H 2O 2 NO Zn Hg2 (NO3 )2 Zn(NO3 )2 2 Hg Similar reaction is given by Pb and Sn. Properties of mercury : Mercury is less reactive than Zn. It is a liquid at room temperature and has low thermal and electrical conductivity. Mercury forms dimeric mercury (I) ions, Hg 22 in which the two atoms are bonded by a covalent bond. It is slowly oxidised to HgO at about its boiling point. Hg does not react with dil. HCl or dil. H 2 SO 4 but reacts with hot concentrated H 2 SO 4 to form HgSO 4 , it reacts with both warm dil. and conc. HNO 3 evolving NO and NO 2 respectively. Hg 2H2SO 4 (hot, conc. ) HgSO 4 SO 2 2H2O Hg 4 HNO3 (conc. ) Hg(NO3 )2 2 NO2 2 H 2O Hg does not react with steam or water hence can’t form any hydroxide. Compounds of mercury (1) Mercuric oxide, HgO : It is obtained as a red solid by heating mercury in air or oxygen for a long time at 673 K d and f-Block Elements Below 400 K, HgI 2 is red but above 400 K, it 673 K 2 Hg O2 2 HgO(red ) or by heating mercuric nitrate alone or in the presence of Hg above 400 turns yellow HgI 2 Kbelow 400 K HgI2 (y ellow) (red) Heat 2 Hg( NO 3 )2 2 HgO 4 NO 2 O2 HgI2 readily dissolves in excess of KI solution to red When NaOH is added to a solution of HgCl2 , yellow precipitate of HgO are obtained. Red and yellow forms of HgO differ only in their particle size. On heating to 673 K, yellow form changes to red form. K 2 HgI4 soluble colourless solution An alkaline solution of K2 [HgI4 ] is called Nessler’s reagent and is used to test NH 4 ions. It gives a brown ppt. of NH 2 Hg O Hg I red It is used in oil paints or as a mild antiseptic in ointments. E3 (Iodide of Millon’s base) with NH 4 ions. 673 K HgO HgO 2K2[HgI4 ] NH 3 3 KOH NH 2.HgO.Hgl 7 KI 2 H 2O It is used in ointments in presence of small amount of MnO 2 Heat HgSO 4 2 NaCl HgCl 2 Na 2 SO 4 MnO 2 treating skin (4) Mercurous chloride, Hg2Cl2 : It is obtained as under : (a) Hg 2 ( NO 3 )2 2 NaCl Hg 2 Cl 2 2 NaNO 3 white ppt. Heat in an iron retort Hg 2 Cl 2 (condenses (b) HgCl 2 Hg U (which oxidises any Hg(I) salts formed during the reaction). for infections. ID (2) Mercuric chloride, HgCl2 : It is obtained by treating Hg with Cl2 or by heating a mixture of NaCl D YG It is a white crystalline solid and is commonly known as corrosive sublimate. It is a covalent compound since it dissolves in organic solvents like ethanol and ether. It is extremely poisonous and causes death. Its best antidote is white of an egg. When treated with stannous chloride, it is first reduced to white ppt. of mercurous chloride and then to mercury (black). 2 KI 60 (y ellow) and HgSO 4 form the (HgI 4 )2 complex ion. HgI2 Red ppt. Hg 2 Cl 2 2 NaOH HgO H 2 O 2 NaCl y ellow 851 U 2 HgCl 2 SnCl 2 Hg 2 Cl 2 SnCl 4 on cooling) It is purified by sublimation. Mercurous chloride is also called calomel. It is a white powder insoluble in H 2O. On heating, it decomposes to give HgCl2 and Hg. Heat Hg 2 Cl 2 HgCl 2 Hg It dissolves in chlorine water forming mercuric chloride. Hg2Cl2 Cl2 2HgCl 2 white ppt. Hg2Cl2 SnCl 2 2 Hg SnCl 4 ST grey With ammonia it gives a white ppt. known as infusible white ppt. HgCl 2 2 NH 3 Hg(NH 2 )Cl NH 4 Cl A dilute solution of HgCl2 is used as an antiseptic. (3) Mercuric iodide, HgI2 : It is obtained when a required amount of KI solution is added to a solution of HgCl2. HgCl 2 2 KI HgI2 2 KCl (red) With ammonia, it turns black due to the formation of a mixture of finely divided black Hg and mercuric amino chloride. Hg2 Cl 2 2NH 3 Hg NH 2 HgCl NH 4 Cl (black) It is used to prepare standard calomel electrode and as a purgative in medicine. (5) Mercuric sulphide, HgS : The solubility product of HgS is lower than that of ZnS and hence it gets precipitated as black solid when H 2 S is passed through an acidic solution of any mercury (II) salt. 852 d and f-Block Elements HgCl 2 H 2 S HgS 2 HCl general electronic configuration is, [ Xe ]4 f 1 14 5 d 0 10 6 s 2. It is insoluble in water and HCl but dissolves in aqua regia (1 part conc. HNO 3 3 parts conc. HCl) NOCl Nitrosy l chloride 2 H 2O (i) These are highly dense metals and possess high melting points. 2[Cl ] Nascent chlorine HgS 2 | Cl | HgCl 2 S (Soluble) On sublimation, its colour changes to red and hence it is used as a red pigment. (6) Mercuric sulphate, HgSO4 : It is obtained when HgS is treated with conc. H 2 SO 4. Hg 2 H 2 SO 4 HgSO 4 SO 2 2H 2O (iii) Oxidation state : Most stable oxidation state of lanthanides is +3. Oxidation states + 2 and + 4 also It is a white solid which decomposes on heating to give mercurous sulphate. electron to become +3 and hence are good reducing agents, where as Ce4+, Pr4+, Tb4+ in aqueous solution gain electron to become + 3 and hence are good oxidizing agents. There is a large gap in energy of 4 f and 5 d subshells and thus the number of oxidation states is limited. (iv) Colour : Most of the trivalent lanthanide ions are coloured both in the solid state and in aqueous solution. This is due to the partly filled f-orbitals which permit f–f transition. The elements with xf electrons have a similar colour to those of (14 – x) electrons. U It is used as a catalyst in the hydration of alkynes to give aldehydes or ketones. It is also used as a cosmetic under the name Vermillon and in ayurvedic medicine as makardhwaj. exist but they revert to +3 e.g. Sm 2 , Eu 2 , Yb 2 lose ID 3 HgSO 4 Hg 2 SO 4 Hg 2 SO 2 2O2 675 K (ii) They form alloys easily with other metals especially iron. e.g. misch metal consists of a rare earth element (94–95%), iron (upto 5%) and traces of S, C, Ca and Al, pyrophoric alloys contain Ce (40–5%), La + neodymium (44%), Fe (4–5%), Al (0–5%) and the rest is Ca, Si and C. It is used in the preparation of ignition devices e.g., trace bullets and shells and flints for lighters and cigarette. 60 Aqua regia Properties of lanthanides E3 3 HCl HNO 3 Promethium (Pm), atomic number 61 is the only synthetic (man made) radioactive lanthanide. D YG (7) Amalgams : Mercury forms alloys commonly known as amalgams, with all metals except iron and platinum. Hence it is transported in iron containers. (8) Alloy of transition metal : See in table discuss earlier in metallurgy. Lanthanides and Actinides ST U Lanthanides and actinides are collectively called f-block elements because last electron in them enters into f-orbitals of the antepenultimate (i.e., inner to penultimate) shell partly but incompletely filled in their elementary or ionic states. The name inner transition, elements is also given to them because they constitute transition series with in transition series (dblock elements) and the last electron enters into antepenultimate shell (n-2)f. In addition to incomplete d-subshell, their f-subshell is also incomplete. Thus, these elements have three incomplete outer shells i.e., (n–2), (n–1) and n shells and the general electronic configuration of f-block elements is (n– (v) Magnetic properties : All lanthanide ions with the exception of Lu3+, Yb3+ and Ce 4+ are paramagnetic because they contain unpaired electrons in the 4 f orbitals. These elements differ from the transition elements in that their magnetic moments do not obey the simple “spin only” formula eff nn 2 B.M. where n is equal to the number of unpaired electrons. In transition elements, the orbital contribution of the electron towards magnetic moment is usually quenched by interaction with electric fields of the environment but in case of lanthanides the 4forbitals lie too deep in the atom for such quenching to occur. Therefore, magnetic moments of lanthanides are calculated by taking into consideration spin as well as orbital contributions and a more complex formula eff 4 S S 1 LL 1 B.M. 2) f 114 (n 1)d 0 10 ns 2. which involves the orbital quantum number L and spin quantum number S. (1) Lanthanides : The elements with atomic numbers 58 to 71 i.e. cerium to lutetium (which come immediately after lanthanum Z = 57) are called lanthanides or lanthanones or rare earths. These elements involve the filling of 4 f-orbitals. Their (vi) Complex formation : Although the lanthanide ions have a high charge (+3) yet the size of their ions is very large yielding small charge to size ratio i.e., low charge density. As a consequence, they have poor tendency to form complexes. They form complexes d and f-Block Elements (vii) Lanthanide contraction : The regular decrease in the size of lanthanide ions from La 3 to 3 Lu is known as lanthanide contraction. It is due to greater effect of the increased nuclear charge than that of the screening effect. Consequences of lanthanide contraction (a) It results in slight variation in their chemical properties which helps in their separation by ion exchange (b) Each element beyond lanthanum has same atomic radius as that of the element lying above it in the group (e.g. Zr 145 pm, Hf 144 pm); Nb 134 pm, Ta 134 pm ; Mo 129 pm, W 130 pm). (c) The covalent character of hydroxides of lanthanides increases as the size decreases from La 3 Similarly, the basicity of oxides also decreases in the order from La 3 to Lu 3 . (d) Tendency to form stable complexes from La 3 oxidation states of actinides is due to the fact that there is a very small energy gap between 5f, 6d and 7s subshells and thus all their electrons can take part in bond formation. (ii) Actinide contraction : There is a regular decrease in ionic radii with increase in atomic number from Th to Lr. This is called actinide contraction analogous to the lanthanide contraction. It is caused due to imperfect shielding of one 5f electron by another in the same shell. This results in increase in the effective nuclear charge which causes contraction in size of the electron cloud. (iii) Colour of the ions : Ions of actinides are generally coloured which is due to f – f transitions. It depends upon the number of electrons in 5 f orbitals. (iv) Magnetic properties : Like lanthanides, actinide elements are strongly paramagnetic. The magnetic moments are lesser than the theoretically predicted values. This is due to the fact that 5f electrons of actinides are less effectively shielded which results in quenching of orbital contribution. U to Lu 3 increases as the size decreases in that order. e.g., UO22 , NpO 22 etc. The exhibition of large number of ID to Lu 3 . However basic strength decreases. Thus La(OH )3 is most basic whereas Lu(OH )3 is least basic. configuration. When the oxidation number increases to + 6, the actinide ions are no longer simple. The high charge density causes the formation of oxygenated ions 60 diketones, oxine etc. No complexes with -bonding ligands are known. E3 mainly with strong chelating agents such as EDTA, - 853 D YG (e) There is a slight increase in electronegativity of the trivalent ions from La to Lu. (f) Since the radius of Yb 3 ion (86 pm) is comparable to the heavier lanthanides Tb, Dy, Ho and Er, therefore they occur together in natural minerals. (2) Actinides : The elements with atomic numbers 90 to 103 i.e. thorium to lawrencium (which come immediately after actinium, Z = 89) are called actinides or actinones. These elements involve the filling of 5 f-orbitals. Their general electronic U configuration is, [Rn ] 5 f 1 14 6 d 0 1 7 s 2. ST They include three thorium, protactinium transuranium elements produced artificially by synthetic or man made radioactive. naturally occuring elements and uranium and eleven or transuranics which are nuclear reactions. They are elements. All actinides are (v) Complex formation : Actinides have a greater tendency to form complexes because of higher nuclear charge and smaller size of their atoms. They form complexes even with -bonding ligands such as alkyl phosphines, thioethers etc, besides EDTA, -diketones, oxine etc. The degree of complex formation decreases in the order. M 4 MO 22 M 3 MO 2 Where M is element of actinide series. There is a high concentration of charge on the metal atom in MO 22 which imparts to it relatively high tendency towards complex formation. Properties of actinides (i) Oxidation state : The dominant oxidation state of actinides is +3 which shows increasing stability for the heavier elements. Np shows +7 oxidation state but this is oxidising and is reduced to the most stable state +5. Pu also shows states upto +7 and Am upto +6 but the most stable state drops to Pu (+4) and Am (+3). Bk in +4 state is strongly oxidising but is more stable than Cm and Am in 4 state due to f 7 configuration. Similarly, No is markedly stable in +2 state due to its f 14 Iron pyrites is also known as Fool’s gold (CuFeS2). Ni can be extracted by or ford process. Chromium oxide is also known as chrome green. Cr2O3/C5H5N or solution of chromic oxide in pyridine is known as Collin’s reagent. 854 d and f-Block Elements Cr2O3.2H2O is also known as Guignet’s green. Schweitzer reagent is [Cu(NH3)4]SO4. Zn, Cd and Hg are not expected to form covalent Etard reagent is CrO2Cl2. bond amongst themselves, hence they have relatively Barfoed’s reagent is Cu(CH3COO)2 + CH3COOH. lower melting point. The +2 oxidation state become more stable while the +3 state become less stable from left to right in Milon’s reagent is the solution of mercuric and mercurous nitrate. Zeigler natta catalyst is TiCl4 + (C2H5)3Al. For the first four element of a transition series Lindlar’s catalyst is Pd/BaSO4. higher oxidation states are more stable than lower oxidation state. For next five-element lower oxidation state are more stable than higher oxidation Wilkinson’s catalyst is [Ph3P]3RhCl. Many transition metal isomorphous e.g. FeSO4.7H2O compound are and ZnSO4.7H2O because of almost equal ionic sizes of these metals. Lightest transition metal is Sc and heaviest and Brown’s catalyst is Nickel boride (P-2 catalyst). ID densest transition element is osmium. Adam catalyst is Pt/PtO. E3 state. 60 the periodic table. Mercurous ion exist as dimer Hg22+ and not as Hg+ and this explains its diamagnetic nature. Pt, Pd and Ir are inert metal. U TiCl4 and TiO2 are used in smoke screens. Mo is used in X-ray tube, Ta in analytic weight D YG and instrument used in surgery of veins. Fe2+ is green and Fe3+ is yellow. Fe3+ is powerful oxidizing agent than Fe2+ ion. Iron carbide or cementite is Fe3C. Kipp’s base is FeS + H2SO4. Copper dissolves in an aqueous solution of FeCl3. CuSO4 reacts only with KI does not react with U KCl, KBr or KF. CuCl2 and CuBr2 are covalent and exist as polymer while CuF2 is an ionic solid. ST All silver halide except AgF are insoluble in water. Ag, Au and Cu are known as coinage metals. Fulminating gold is Au(NH2) = NH or N2H3. Purple of cassius is a colloidal solution of gold. Mercuric salts are more stable than mercurous salts. All the elements of f-block are in group 3 of the periodic table.