Cellular Energetics 2024 PDF
Document Details
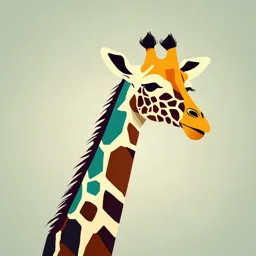
Uploaded by WittyVision4473
American University of Antigua
Tags
Related
- El Mundo de la Célula - Metabolismo Quimiótrofo - PDF
- Guía Bioquímica 0801B - Guía de Respiración Celular - PDF
- Elementi di Scienze Naturali PDF
- Clases Teóricas: Metabolismo - ITBA
- Unit 3 Cell Energetics Ch 8 9 and 10 Enzymes Cellular Respiration and Photosynthesis notes PDF
- Biologia Cellulare - Appunti PDF
Summary
This document provides a detailed overview of cellular energetics, including metabolism, ATP hydrolysis, cellular respiration, and different types of fermentation, such as alcoholic and lactic acid fermentation. The information is presented using diagrams and text, which makes the material easy to follow and digest.
Full Transcript
Cellular Energetics Metabolism Metabolism = sum of all chemical reactions in the body Human Metabolic Map is Highly Complex Overview: Aerobic Oxidation & Photosynthesis Reactions may be Exergonic or Endergonic Exergonic reactions: Endergonic reactio...
Cellular Energetics Metabolism Metabolism = sum of all chemical reactions in the body Human Metabolic Map is Highly Complex Overview: Aerobic Oxidation & Photosynthesis Reactions may be Exergonic or Endergonic Exergonic reactions: Endergonic reactions: release energy (downhill) require energy (uphill) ΔG = negative ΔG = positive – spontaneous – non-spontaneous catabolic (breakdown) anabolic (build-up) Energy Coupling Endergonic reactions are coupled to exergonic reactions – Theoretically, any endergonic reaction can be coupled to any exergonic reaction. (However, this is not the case.) ATP Hydrolysis ATP: major energy currency of cells ATP hydrolysis: the most common exergonic coupling reaction – ATP ADP + Pi ΔG = -7.3 kcal/mol After ATP is hydrolyzed, how does a cell obtain more ATP? – Cells can synthesize new ATP or regenerate ATP from ADP + Pi Regeneration of ATP is more energetically favorable – Cellular respiration evolved to regenerate ATP from ADP and Pi. Average human regenerates about 100 moles ATP per day – = ~6X1020 ATP regenerated per second! Cellular Respiration Chemical equation for aerobic cellular respiration is: C6H12O6 + 6O2 6H2O + 6CO2 – in this reaction: glucose is oxidized to CO2 oxygen is reduced to H2O complete eukaryotic oxidation of glucose requires both the cytosol and mitochondria Mitochondrion Origin of Mitochondria Process called endosymbiosis: – Mitochondria evolved from free-living bacteria that invaded or was engulfed by an early eukaryotic cell (or another prokaryote) – It then evolved to co-exist with its host, with some of its genes moving to the nucleus. Aerobic Cellular Respiration Obligate aerobes, facultative aerobes and facultative anaerobes can undergo aerobic respiration. 4 subsets of reactions that are spatially separated: Reactions Location Glycolysis Cytosol Oxidation of pyruvate Mitochondrial matrix TCA cycle Mitochondrial matrix Chemiosmosis, Oxidative Mitochondrial matrix, Phosphorylation & Electron intermembrane space & inner Transport Chain mitochondrial membrane Summary of Aerobic Oxidation of Glucose & Fatty Acids Comparing Substrate-Level & Oxidative Phosphorylation 1. Substrate-level phosphorylation: 2. Oxidative Phosphorylation: uses the electron transport chain and oxygen most of the ATP made during aerobic cellular respiration is made here requires electrons, which are “captured” during glycolysis, the oxidation of pyruvate and the Kreb’s cycle Electron Carriers Two electron carriers of respiration: – NAD+: Nicotinamide Adenine Dinucleotide – FAD: Flavin Adenine Dinucleotide (Vitamin B2) Redox of NAD+ and FAD: Oxidized forms of these compounds do not carry e-, while reduced forms carry 2 e- each NAD+NADH + H+ (2e- gained) FADFADH2 (2e- gained) Glycolysis 2 subsets of reactions – Energy-requiring phase: need 2 ATP per glucose – Energy-yielding phase: produce 4 ATP per glucose By the end of glycolysis, per glucose: 2NADH (4 e-) 2 pyruvate 4 ATP (2 net) by substrate level phosphorylation What happens if there is no O2?. Cell can only regenerate ATP by glycolysis – Typically increases uptake of glucose (Pasteur effect). Pasteur effect: the shift from slow aerobic to rapid anaerobic consumption of glucose was first noted by Pasteur. This shift also happens anytime you are unable to provide oxygen to your own mitochondria- they consume glucose faster in an attempt to produce ATP via the less efficient fermentation to lactate, and lactic acid accumulates in your muscles. To continue break-down of glucose need NAD+, so NADH is oxidized to NAD+ by fermentation. 2 common forms of fermentation are: – alcohol fermentation in yeast – lactic acid fermentation in human muscle cells Fermentation Yeast would produce ethanol from glucose via a process called alcoholic fermentation When oxygen is scarce in muscle cells, NADH reduces pyruvate to form lactic acid, regenerating NAD+, via a process called lactic acid fermentation In Presence of O2, Pyruvate Enters Mitochondria Pyruvate Acetyl-CoA TCA Cycle TCA Cycle, Kreb’s Cycle, Citric Acid Cycle Tally-Sheet After TCA Cycle yield of various substances from 1 glucose molecule thus far: – NADH 10 [2 glycolysis, 2 pyruvate oxidation, 6 TCA cycle] – FADH2 2 [TCA cycle] – CO2 6 [2 oxidation of pyruvate, 4 TCA cycle] – ATP 6 [4 net] Electron Transport Chain & Oxidative Phosphorylation NADH and FADH2 e- carriers release their e- into the electron transport chain (ETC) – located within the inner mitochondrial membrane – e- transfer between ETC members driven by final e- acceptor O2, so the process is named: oxidative phosphorylation. Electron Transport Chain & Oxidative Phosphorylation ETC consists of: – 5 protein complexes (Complexes I-V): Complex I = NADH dehydrogenase or NADH-CoQ reductase Complex II = Succinate coenzyme Q reductase Complex III = Cytochrome c reductase Complex IV = Cytochrome c oxidase Complex V = ATP Synthase – 2 carriers: Coenzyme Q (CoQ) or ubiquinone CoQ/CoQH2 (a lipid soluble coenzyme Q) Cytochrome c (Cyt c)- water soluble protein e- Flow through Complexes I-IV & Carriers NADH e- : I → CoQ → III → Cyt C → IV → O2 FADH2 e-: II → CoQ → III → Cyt C → IV→ O2 e- from NADH e- from FADH2 What happens as e- flow? As e- flow, protons (H+) move from the mitochondrial matrix to the intermembrane space. – the e- transferred from each NADH result in the movement of ~10 H+ into the intermembrane space – the e- transferred from each FADH2 result in the movement of ~ 6 H+ into the intermembrane space Why the difference? Complex V = ATP Synthase H+ cannot return to the matrix through the membrane ATP synthase provides a channel through which they can move movement of 3 H+ through ATP synthase powers regeneration of ~ 1 ATP ATP Made by Oxidative Phosphorylation Total ATP by Oxidative Phosphorylation: – From each NADH, 3ATP: 10 NADH X 3 = 30 ATP – From each FADH2, 2 ATP: 2 FADH2 X 2 = 4 ATP But, remember that 2 NADH were made in the cytosol during glycolysis: – NADH cannot move through the mitochondrial inner membrane. these e- cannot be used in the ETC unless they are transported across the membrane. – Process of e- shuttle (malate shuttle and others) uses energy to move NADH across (~1 ATP per NADH) so the net ATP yield from one cytosolic made NADH is 2 (= 3 – 1) The Malate Shuttle Cylical series of reactions transfers e- from NADH in cytosol across inner mitochondrial membrane (NADH impermeable) to NAD+ in the matrix Result: cytosolic NADH NAD+ and matrix NAD+ NADH Revised ATP Yield from all of Aerobic Cellular Respiration Oxidative Phosphorylation: NADH: 10 NADH X 3 = 30 ATP - 2 ATP (e- shuttle) = 28 ATP FADH2: 2 FADH2 X 2 = 4 ATP Therefore, 32 net ATP from oxidative phosphorylation Substrate-level phosphorylation: 4 net ATP from substrate-level phosphorylation Grand total = 36 net ATP per glucose Summary of Aerobic Oxidation of Glucose & Fatty Acids Transport Across Mitochondrial Membranes Outer mitochondrial membrane contains many porins: – Relatively non-selective transmembrane proteins that allow ions, small molecules, NADH, ATP, ADP, etc. to move through Inner mitochondrial membrane is more selective – major selectivity barrier for movement of substances into and out of matrix – IMM Transport examples: Malate Shuttle Phosphate transporter ATP/ADP antiporter Control of Respiration Rate of respiration is affected in a variety of ways: 1. Allosteric control of respiration occurs when phosphofructokinase-1 is stimulated or inhibited. AMP is an activator, whereas ATP and citrate are inhibitors. Control of Respiration 2. ADP levels: endogenous ADP is depleted by ATP formation low levels of ADP decrease rate of respiration, while increased amounts of ADP increases respiratory rate 3. Uncouplers: a substance that separates the proton-motive force across the inner mitochondrial membrane from ATP synthesis and inhibits ATP synthesis include chemical uncouplers & natural substances 4. Metabolic Blocks prevent e- flow through the ETC Chemical Uncoupler: 2,4-dinitrophenol (DNP) NORMAL: WITH 2,4-DNP: Natural substance Uncoupler: Thermogenin Thermogenin: a proton carrier protein found on the inner mitochondrial membrane of brown-fat mitochondria (brown fat tissue) – by moving H+ across the inner membrane and not through ATP synthase, heat (but not ATP) is generated – brown fat is more common in children than adults Metabolic Blocks typically prevent the flow of electrons through the ETC – disrupt proton gradient, little or no ATP generated – some block ATP synthase so protons cannot move through examples: cyanide, rotenone, malonate, antimycin-a, oligomycin Alternative Fuels Most cells “prefer” glucose as the molecule of choice for respiration. In its absence, cells can metabolize other organic compounds to regenerate ATP. 2 mechanisms are: – β-oxidation: use of fatty acids for mitochondrial respiration – Gluconeogenesis: production of glucose from sources other than glycogen Note: Some cells such as neurons, erythrocytes, and kidney medullar cells use only glucose for respiration, whereas others may preferentially use fatty acids. For example, fatty acids are the major energy source for cardiomyocytes. β-Oxidation using fatty acids for mitochondrial respiration fatty acids are stored in adipose cells (adipocytes) as triglycerides – when needed, triglycerides are oxidized to fatty acids in cytosol – triglycerol + H20 Fatty acid + glycerol fatty acids are esterified to CoA to form fatty acyl CoA – fatty acid + HSCoA + ATP Fatty acyl CoA + AMP + PPi fatty acyl group transferred to cytosolic carnitine molecule and moves thru the IMM by acyl carnitine transporter protein in matrix: carnitine releases fatty acyl group, which combines with another CoA molecule to form fatty acyl CoA – fatty acyl CoA is oxidized to Acetyl Co-A Fatty Acid Oxidation Fatty acids converted to Acetyl CoA by repeated series of 4 enzyme catalyzed reactions Termed β-oxidation b/c it occurs by sequential removal of 2-carbon units by oxidation at the β-carbon position of the fatty acyl-CoA molecule Each round of mitochondrial β-oxidation of 1 molecule of fatty acyl CoA to Acetyl CoAs produces 1 FADH2 & 1 NADH for each Acetyl CoA made – Example: 18 C fatty acyl molecule yields 9 Acetyl CoA, 8 NADH & 8 FADH2 β-oxidation cont. Acetyl-CoA (end product of each round of β-oxidation) enters the TCA cycle where it is further oxidized to CO2 – also generated in TCA cycle are 3 molecules of NADH, 1 molecule of FADH2 and 1 molecule of ATP – NADH and FADH2 generated during the fat oxidation and Acetyl- CoA oxidation in the TCA cycle enter ETC to produce ATP Fatty acids oxidation yields significantly more energy per carbon atom than does the oxidation of carbohydrates – Eg) net result of the oxidation of one mole of 18-C fatty acyl CoA will be 146 moles of ATP; in comparison 114 moles would be generated from an equivalent number of glucose carbon atoms. Very long Chain Acyl CoA Dehydrogenase Deficiency (VLCADD) an autosomal recessive disorder resulting in an intramitochondrial defect in β-oxidation body cannot oxidize fatty acids b/c an enzyme is absent or not functioning correctly can cause severe hypoketotic hypoglycemia, liver dysfuction w/ hepatomegaly, cardiomyopathy, encephalopathy, lethargy, sudden death Gluconeogenesis Metabolic pathway resulting in glucose formation from noncarbohydrate sources – Site: occurs in liver & kidney when dietary carbohydrate sources are insufficient to meet the body’s glucose demands it occurs partly in cytosol and partly in mitochondria Importance of gluconeogenesis: – chief source of blood glucose after the 1st 18 hrs fasting – removes blood lactate produced by RBC and muscles and blood glycerol produced by adipose tissue or absorbed by intestine when glycogen stores are depleted (muscle exertion, liver during fasting), muscle protein catabolism to AA contributes major carbon source to maintain blood glucose levels Precursors include: pyruvate, lactate, glycerol, glucogenic AA – all 20 AA (except L & K) can be degraded to TCA intermediates – allows the carbon skeletons of the AA to be converted to those in OAA and pyruvate Gluconeogenesis notes: – Non-carbohydrate sources and entry points for gluconeogenesis (yellow) – Enzymes used only for gluconeogenesis (pink) – Enzymes used for both gluconeogenesis and glycolysis (blue)