Systems Physiology Cardiovascular PDF
Document Details
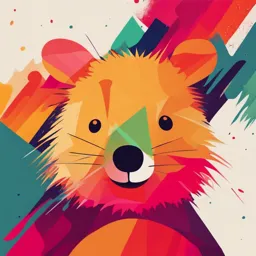
Uploaded by SuccessfulNavy3461
Faculty of Pharmacy
Rania Azar
Tags
Summary
This document presents an overview of the cardiovascular system, including outlines, summaries, and explanations of various aspects like cardiovascular physiology, components, the heart, and its function. It's a good resource for an undergraduate-level understanding of the subject.
Full Transcript
SYSTEMS PHYSIOLOGY Prof. Rania AZAR Pharm-D, MS, PhD OUTLINES Cardiovascular physiology Respiratory physiology The kidneys and regulation of water and inorganic The digestion and absorption of food Regulation of organic metabolism and energy balance Endocrine system Reproduction M...
SYSTEMS PHYSIOLOGY Prof. Rania AZAR Pharm-D, MS, PhD OUTLINES Cardiovascular physiology Respiratory physiology The kidneys and regulation of water and inorganic The digestion and absorption of food Regulation of organic metabolism and energy balance Endocrine system Reproduction Medical physiology: Integration SYSTEMS PHYSIOLOGY Cardiovascular Summary Overview of the Circulatory System The Vascular System – Components of the Circulatory System – Arteries – Pressure, Flow, and Resistance – Arterioles – Capillaries The Heart – Veins – Anatomy – The Lymphatic System – Heartbeat Coordination – Mechanical Events of the Cardiac Cycle Integration of Cardiovascular Function: Regulation of – The Cardiac Output Systemic Arterial Pressure – Measurement of Cardiac Function – Baroreceptor Reflexes – Blood Volume and Long-Term Regulation of Arterial Pressure – Other Cardiovascular Reflexes and Responses Overview of the Circulatory System Components of the Circulatory System We will begin with an overview of the components of the circulatory system and a discussion of some of the physical factors that determine its function. Blood is composed of formed elements (cells and cell fragments) suspended in a liquid called plasma. The hematocrit is defined as the percentage of blood volume that is erythrocytes. – It is measured by centrifuging (spinning at high speed) a sample of blood. – The erythrocytes are forced to the bottom of the centrifuge tube, the plasma remains on top, and the Estimate the hematocrit of a person with a plasma volume of 3 L leukocytes and platelets form a very thin layer and total blood volume of 4.5 L. between them called the buffy coat (Figure). Overview of the Circulatory System Components of the Circulatory System The rapid flow of blood throughout the body is produced by pressures created by the pumping action of the heart. This type of flow is known as bulk flow because all constituents of the blood move together. – The extraordinary degree of branching of blood vessels ensures that almost all cells in the body are within a few cell diameters of at least one of the smallest branches, the capillaries. – Nutrients and metabolic end products move between capillary blood and the interstitial fluid by diffusion. – Movements between the interstitial fluid and the cell interior are accomplished by both diffusion and mediated transport across the plasma membrane. At any given moment, only about 5% of the total circulating blood is actually in the capillaries. – Yet, it is this 5% that is performing the ultimate functions of the entire circulatory system: the supplying of nutrients and hormonal signals and the removal of metabolic end products and other cell secretions. – All other components of the system serve the overall function of getting adequate blood flow through the capillaries. Overview of the Circulatory System Components of the Circulatory System As British physiologist William Harvey reported in 1628, the circulatory system forms a closed loop, so that blood pumped out of the heart through one set of vessels returns to the heart by a different set. There are actually two circuits, both originating and terminating in the heart, which is divided longitudinally into two functional halves. – Each half of the heart contains two chambers: an upper chamber—the atrium —and a lower chamber—the ventricle. – The atrium on each side empties into the ventricle on that side, but there is usually no direct blood flow between the two atria or the two ventricles in the heart of a healthy adult. Overview of the Circulatory System Components of the Circulatory System In the systemic circuit, blood leaves the left ventricle via a single large artery, the aorta. The pulmonary circulation is composed of a similar circuit. As blood flows through the lung capillaries, it picks up oxygen supplied to the lungs by breathing. Therefore, the blood in the pulmonary veins, left side of the heart, and systemic arteries has a high oxygen content. As this blood flows through the capillaries of peripheral tissues and organs, some of this oxygen leaves the blood to enter and be used by cells, resulting in the lower oxygen content of systemic venous blood. As shown in the Figure, blood can pass from the systemic veins to the systemic arteries only by first being pumped through the lungs. Thus, the blood returning from the body’s peripheral organs and tissues via the systemic veins is oxygenated before it is pumped back to them. Overview of the Circulatory System Components of the Circulatory System Note that the lungs receive all the blood pumped by the right side of the heart, whereas the branching of the systemic arteries results in a parallel pattern so that each of the peripheral organs and tissues receives only a fraction of the blood pumped by the left ventricle. This arrangement – (1) guarantees that all systemic tissues receive freshly oxygenated blood and – (2) allows for independent variation in blood flow through different tissues as their metabolic activities change. – For reference, the typical distribution of the blood Predict how the blood flow to pumped by the left ventricle in an adult at rest is these various areas might given in the Figure. change in a resting person just after eating a large meal. Overview of the Circulatory System Pressure, Flow, and Resistance An important feature of the circulatory system is the relationship among blood pressure, blood flow, and the resistance to blood flow. – As applied to blood, these factors are collectively referred to as hemodynamics , and they demonstrate the general principle of physiology that physiological processes are dictated by the laws of chemistry and physics. Flow between two points – In all parts of the system, blood flow ( F ) is always within a tube is from a region of higher pressure to one of lower proportional to the pressure pressure. difference between the points. The flows in these two – The pressure exerted by any fluid is called a identical tubes are the same hydrostatic pressure , but this is usually shortened (10 mL/min) because the simply to “pressure” in descriptions of the pressure differences are the cardiovascular system, and it denotes the force same. exerted by the blood. Overview of the Circulatory System Pressure, Flow, and Resistance Knowing only the pressure difference between two points will not tell you the flow rate, however. For this, you also need to know the resistance ( R ) to flow—that is, how difficult it is for blood to flow between two points at any given pressure difference. what is it that actually determines the resistance? Resistance cannot be measured directly, but it can be calculated from the directly measured F and Δ P. For example, the resistances = fluid viscosity in both tubes can be L = length of the tube calculated: 90 mmHg / 10 R = inside radius of the tube mL/min = 9 mmHg/mL/min Overview of the Circulatory System Pressure, Flow, and Resistance In contrast, the radii of the blood vessels do not remain constant, and so vessel radius—the l/ r 4 term in our equation—is the most important determinant of changes in resistance along the blood vessels. – Figure demonstrates how radius influences the resistance and, as a consequence, the flow of fluid through a tube. – Decreasing the radius of a tube twofold increases its resistance 16-fold. If Δ P is held constant in this example, flow through the tube decreases 16-fold because F = Δ P / R. The equation relating pressure, flow, and resistance applies not only to flow through blood vessels but also to the flows into and out of the various chambers of the heart. – These flows occur through valves, and the resistance of a valvular opening determines the flow through the valve at any If outlet B in Figure (b) had two given pressure difference across it. individual outlet tubes, each with a radius of 1, would the flow be equal to side A? Overview of the Circulatory System Pressure, Flow, and Resistance THE HEART: Anatomy The heart is a muscular organ enclosed in a protective fibrous sac, the pericardium , and located in the chest. A fibrous layer is also closely affixed to the heart and is called the epicardium. The extremely narrow space between the pericardium and the epicardium is filled with a watery fluid that serves as a lubricant as the heart moves within the sac. THE HEART: Anatomy The wall of the heart, the myocardium , is composed primarily of cardiac muscle cells. The inner surface of the cardiac chambers, as well as the inner wall of all blood vessels, is lined by a thin layer of cells known as endothelial cells , or endothelium. As noted earlier, the human heart is divided into right and left halves, each consisting of an atrium and a ventricle. – The two ventricles are separated by a muscular wall, the interventricular septum. – Located between the atrium and ventricle in each half of the heart are the one-way atrioventricular ( AV ) valves , which permit blood to flow from atrium to ventricle but not backward from ventricle to atrium. – The right AV valve is called the tricuspid valve because it has three fibrous flaps, or cusps. – The left AV valve has two flaps and is therefore called the bicuspid valve. – Its resemblance to a bishop’s headgear (a “mitre”) has earned the left AV valve another commonly used name, mitral valve. THE HEART: Anatomy The openings of the right ventricle into the pulmonary trunk and of the left ventricle into the aorta also contain valves, the pulmonary and aortic valves , respectively. There are no valves at the entrances of the superior and inferior venae cavae (plural of vena cava ) into the right atrium, and of the pulmonary veins into the left atrium. – However, atrial contraction pumps very little blood back into the veins because atrial contraction constricts their sites of entry into the atria, greatly increasing the resistance to backflow. – (Actually, a little blood is ejected back into the veins, and this accounts for the venous pulse that can often be seen in the neck veins when the atria are contracting.) How would this diagram be different if it included a systemic portal vessel? THE HEART: Anatomy Cardiac Muscle The bulk of the heart is comprised of specialized muscle cells with amazing resiliency and stamina. cardiac muscle is similar to smooth and skeletal muscle. – It is an electrically excitable tissue that converts chemical energy stored in the bonds of ATP into force generation. – Action potentials propagate along cell membranes, Ca 2+ enters the cytosol, and the cycling of force-generating cross-bridges is activated. Approximately 1% of cardiac cells do not function in contraction but have specialized features that are essential for normal heart excitation. – These cells constitute a network known as the conducting system of the heart and are in electrical contact with the cardiac muscle cells via gap junctions. – The conducting system initiates the heartbeat and helps spread an action potential rapidly throughout the heart. THE HEART: Anatomy Cardiac Muscle Innervation The heart receives a rich supply of sympathetic and parasympathetic nerve fibers, the latter contained in the vagus nerves ( Figure). Blood Supply The blood being pumped through the heart chambers does not exchange nutrients and metabolic end products with the myocardial cells. They, like the cells of all other organs, receive their blood supply via arteries that branch from the aorta. The arteries supplying the myocardium are the coronary arteries , and the blood flowing through them is the coronary blood flow. The coronary arteries exit from behind the aortic valve cusps in the very first part of the aorta and lead to a branching network of small arteries, arterioles, capillaries, venules, and veins similar to those in other organs. Most of the cardiac veins drain into a single large vein, the coronary sinus, which empties into the right atrium. THE HEART: Heart beat coordination The heart is a dual pump in that the left and right sides of the heart pump blood separately—but simultaneously— into the systemic and pulmonary vessels. Efficient pumping of blood??? Contraction of cardiac muscle, like that of skeletal muscle and many smooth muscles, is triggered by depolarization of the plasma membrane. – Gap junctions interconnect myocardial cells and allow action potentials to spread from one cell to another. – The initial excitation of one cardiac cell therefore eventually results in the excitation of all cardiac cells. This initial depolarization normally arises in a small group of conducting-system cells called the sinoatrial ( SA ) node , – (1) What is the path of spread of excitation? – (2) How does the SA node initiate an action potential? THE HEART: Heart beat coordination Sequence of Excitation The SA node is normally the pacemaker for the entire heart. Its depolarization generates the action potential that leads to depolarization of all other cardiac muscle cells. – As we will see later, electrical excitation of the heart is coupled with contraction of cardiac muscle. – Therefore, the discharge rate of the SA node determines the heart rate , the number of times the heart contracts per minute. The action potential initiated in the SA node spreads throughout the myocardium, passing from cell to cell by way of gap junctions. – Depolarization first spreads through the muscle cells of the atria, with conduction rapid enough that the right and left atria contract at essentially the same time. The spread of the action potential to the ventricles involves a more complicated conducting system, which consists of modified cardiac cells that have lost contractile capability but that conduct action potentials with low resistance. THE HEART: Heart beat coordination Cardiac Action Potentials and Excitation of the SA Node The mechanism by which action potentials are conducted along the membranes of heart cells is basically similar to other excitable tissues like neurons and skeletal muscle cells. – However, different types of heart cells express unique combinations of ion channels that produce different action potential shapes. – In this way, they are specialized for particular roles in the spread of excitation through the heart. The current due to outward K+ movement is nearly equal to the current due to inward Ca2+ movement during the plateau of the action potential, and yet the membrane permeability to Ca2+ is much greater. How can the currents be similar despite the permeability difference? THE HEART: Heart beat coordination Cardiac Action Potentials and Excitation of the SA Node In contrast, there are extremely important differences between action potentials of cardiac muscle cells and those in nodal cells of the conducting system. – Figure a illustrates the action potential of a cell from the SA node. – Three ion channel mechanisms, which are shown in Figure b , contribute to the pacemaker potential. The pacemaker potential provides the SA node with automaticity , the capacity for spontaneous, rhythmic self-excitation. Conducting cells of the ventricles contain all of the ion channel types found in both cardiac muscle cells and node cells. Draw a graph showing a Purkinje cell action potential. THE HEART: Heart beat coordination The Electrocardiogram The electrocardiogram ( ECG , also abbreviated EKG — the k is from the German elektrokardiogramm ) is a tool for evaluating the electrical events within the heart. – When action potentials occur simultaneously in many individual myocardial cells, currents are conducted through the body fluids around the heart and can be detected by recording electrodes at the surface of the skin. – Figure a illustrates an idealized normal ECG recorded as the potential difference between the right and left wrists. How would the timing of the waves in (a) be changed by a drug that reduces the L-type Ca2+ current in AV node cells? THE HEART: Heart beat coordination The Electrocardiogram Because many myocardial defects alter normal action potential propagation, and thereby the shapes and timing of the waves, the ECG is a powerful tool for diagnosing certain types of heart disease. Figure 12.16 gives one example. – However, note that the ECG provides information concerning only the electrical activity of the heart. – If something is wrong with the heart’s mechanical activity and the defect does not give rise to altered electrical activity, the ECG will be of limited diagnostic value. THE HEART: Heart beat coordination Excitation–Contraction Coupling The mechanisms linking cardiac muscle cell action potentials to contraction were described in detail last year in the chapter on muscle physiology. You may recall that in skeletal muscle, a single action potential releases sufficient Ca 2+ to fully saturate the troponin sites that activate contraction. Refractory Period of the Heart Cardiac muscle is incapable of undergoing summation of contractions like that occurring in skeletal muscle, and this is a very good thing. As in the case of neurons and skeletal muscle fibers, the main mechanism is the inactivation of Na+ channels. THE HEART: Mechanical events 0f cardiac cycle The orderly process of depolarization described in the previous sections triggers a recurring cardiac cycle of atrial and ventricular contractions and relaxations. THE HEART: Mechanical events 0f cardiac cycle During the first part of diastole, the ventricles begin to relax and the aortic and pulmonary valves close. – (Physiologists and clinical cardiologists do not all agree on the dividing line between systole and diastole; as presented here, the dividing line is the point at which ventricular contraction stops and the pulmonary and aortic valves close.) – At this time, the AV valves are also closed; therefore, no blood is entering or leaving the ventricles. – Ventricular volume is not changing, and this period is called isovolumetric ventricular relaxation. – Note, then, that the only times during the cardiac cycle that all valves are closed are the periods of isovolumetric ventricular contraction and relaxation. Next, the AV valves open and ventricular filling occurs as blood flows in from the atria. – Atrial contraction occurs at the end of diastole, after most of the ventricular filling has taken place. – The ventricle receives blood throughout most of diastole, not just when the atrium contracts. – Indeed, in a person at rest, approximately 80% of ventricular filling occurs before atrial contraction. THE HEART: Mechanical events 0f cardiac cycle Mid-Diastole to Late Diastole – Our analysis of events in the left atrium and ventricle and the aorta begins at the far left of Figure with the events of mid- to late diastole. Systole – Thus far, the ventricle has been relaxed as it fills with blood. But immediately following the atrial contraction, the ventricles begin to contract. Early Diastole – This phase of diastole begins as the ventricular muscle relaxes and ejection comes to an end. Early Diastole – The fact that most ventricular filling is completed during early diastole is of great importance. THE HEART: Mechanical events 0f cardiac cycle Pulmonary Circulation Pressures Note that the pressures are lower than in the left The pressure changes in the right ventricle and aorta. ventricle and pulmonary arteries ( Figure) are qualitatively similar to those just described for the left ventricle and aorta. There are striking quantitative differences, however. Typical pulmonary If a person had a hole in the arterial systolic and diastolic pressures interventricular septum, are 25 and 10 mmHg, respectively, would the blood ejected compared to systemic arterial pressures into the aorta have lower of 120 and 80 mmHg. than normal oxygen levels? THE HEART: Mechanical events 0f cardiac cycle Heart Sounds Two heart sounds resulting from cardiac contraction are normally heard through a stethoscope placed on the chest wall. Murmurs can be produced by heart defects that cause blood flow to be turbulent. The exact timing and location of the murmur provide the physician with a powerful diagnostic clue. THE HEART: The cardiac output The volume of blood each ventricle pumps as a function of time, usually expressed in liters per minute, is called the cardiac output ( CO ). – In the steady state, the cardiac output flowing through the systemic and the pulmonary circuits is the same. – The cardiac output is calculated by multiplying the heart rate ( HR )—the number of beats per minute—and the stroke volume ( SV )—the blood volume ejected by each ventricle with each beat: CO = HR*SV The following description of the factors that alter the two determinants of cardiac output—heart rate and stroke volume—applies in all respects to both the right and left sides of the heart because stroke volume and heart rate are the same for both under steady-state conditions. – Heart rate and stroke volume do not always change in the same direction. – For example, stroke volume decreases following blood loss, whereas heart rate increases. – These changes produce opposing effects on cardiac output. THE HEART: The cardiac output Control of Heart Rate Rhythmic beating of the heart at a rate of approximately 100 beats/min will occur in the complete absence of any nervous or hormonal influences on the SA node. – This is the inherent autonomous discharge rate of the SA node. – The heart rate may be slower or faster than this, however, because the SA node is normally under the constant influence of nerves and hormones. A large number of parasympathetic and sympathetic postganglionic neurons end on the SA node. – Activity in the parasympathetic neurons (which travel within the vagus nerve) causes the heart rate to decrease, whereas activity in the sympathetic neurons causes an increase. – In the resting state, there is considerably more parasympathetic activity to the heart than sympathetic, so the normal resting heart rate of about 70 beats/min is well below the inherent rate of 100 beats/min. THE HEART: The cardiac output Control of Stroke Volume The second variable that determines cardiac output is stroke volume—the volume of blood each ventricle ejects during each contraction. Recall that the ventricles do not completely empty themselves during contraction. Therefore, a more forceful contraction can produce an increase in stroke volume by causing greater emptying. Changes in the force during ejection of the stroke volume can be produced by a variety of factors, but three are dominant under most physiological and pathophysiological conditions: – (1) changes in the end-diastolic volume (the volume of blood in the ventricles just before contraction, sometimes referred to as the preload ); – (2) changes in the magnitude of sympathetic nervous system input to the ventricles; and – (3) changes in afterload (i.e., the arterial pressures against which the ventricles pump). THE HEART: The cardiac output Control of Stroke Volume Relationship Between Ventricular End-Diastolic Volume and Stroke Volume: The Frank–Starling Mechanism – The mechanical properties of cardiac muscle form the basis for an inherent mechanism for altering the strength of contraction and stroke volume; the ventricle contracts more forcefully during systole when it has been filled to a greater degree during diastole. – In other words, all other factors being equal, the stroke volume increases as the end-diastolic volume increases. – This is illustrated graphically as a ventricular-function curve ( Figure ). – This relationship between stroke volume and end-diastolic volume is known as the Frank–Starling mechanism (also called Starling’s law of the heart ) in recognition of the two physiologists who identified it. – What accounts for the Frank–Starling mechanism? – What is important in it? Or the significance THE HEART: The cardiac output Control of Stroke Volume Sympathetic Regulation The Frank–Starling mechanism still applies, but during sympathetic stimulation, the stroke volume is greater at any given end-diastolic volume. In other words, the increased contractility leads to a more complete ejection of the end-diastolic ventricular volume. Estimate the ejection fraction and end-systolic volumes under control and sympathetic stimulation conditions at an end-diastolic volume of 140 mL. THE HEART: The cardiac output Control of Stroke Volume Sympathetic Regulation One way to quantify contractility is through the ejection fraction ( EF ) , defined as the ratio of stroke volume ( SV )to end-diastolic volume ( EDV ): EF = SV/EDV Cellular mechanisms involved in sympathetic regulation of myocardial contractility are shown in Figure. THE HEART: The cardiac output Control of Stroke Volume Sympathetic Regulation THE HEART: The cardiac output Control of Stroke Volume Figure integrates the factors that determine stroke Afterload volume and heart rate into a summary of the control of cardiac output. An increased arterial pressure tends to reduce stroke volume. This is because, like a skeletal muscle lifting a weight, the arterial pressure constitutes a “load” that contracting ventricular muscle must work against when it is ejecting blood. A term used to describe how hard the heart must work to eject blood is afterload. The greater the load, the less contracting muscle fibers can shorten at a given contractility. This factor will not be dealt with further, because in the normal heart, several inherent adjustments minimize the overall influence of arterial pressure on stroke volume. Parasympathetic nerves do not innervate the ventricles. Is it therefore impossible for parasympathetic activity to influence stroke volume? THE HEART: Measurement of cardiac function Human cardiac output and heart function can be measured by a variety of methods. For example, echocardiography can be used to obtain two- and three-dimensional images of the heart throughout the entire cardiac cycle. – In this procedure, ultrasonic waves are beamed at the heart and returning echoes are electronically plotted by computer to produce continuous images of the heart. – It can detect the abnormal functioning of cardiac valves or contractions of the cardiac walls, and it can also be used to measure ejection fraction. Echocardiography is a noninvasive technique because everything used remains external to the body. Other visualization techniques are invasive. One, cardiac angiography , requires the temporary threading of a thin, flexible tube called a catheter through an artery or vein into the heart. A liquid containing radiopaque contrast material is then injected through the catheter during high- speed x-ray videography. This technique is useful not only for evaluating cardiac function but also for identifying narrowed coronary arteries. The Vascular System Although the action of the muscular heart provides the overall driving force for blood movement, the vascular system plays an active role in regulating blood pressure and distributing blood flow to the various tissues. Elaborate branching and regional specializations of blood vessels enable efficient matching of blood flow to metabolic demand in individual tissues. the pressure changes that occur along the rest of the systemic and pulmonary circulations. The Vascular System Endothelial cells have a large number of functions: – Serve as a physical lining that blood cells do not normally adhere to in heart and blood vessels – Serve as a permeability barrier for the exchange of nutrients, metabolic end products, and fluid between plasma and interstitial fluid; regulate transport of macromolecules and other substances – Secrete paracrine agents that act on adjacent vascular smooth muscle cells, including vasodilators such as prostacyclin and nitric oxide (endothelium-derived relaxing factor [EDRF]), and vasoconstrictors such as endothelin-1 – Mediate angiogenesis (new capillary growth) Play a central role in vascular remodeling by detecting signals and releasing paracrine agents that act on adjacent cells in the blood vessel wall – Contribute to the formation and maintenance of extracellular matrix – Produce growth factors in response to damage – Secrete substances that regulate platelet clumping, clotting, and anticlotting – Synthesize active hormones from inactive precursors – Extract or degrade hormones and other mediators – Secrete cytokines during immune responses – Influence vascular smooth muscle proliferation in the disease atherosclerosis The Vascular System: Arteries Arterial Blood Pressure The term used to denote how easily a structure stretches is compliance : Compliance = Volume/Pressure – The greater the compliance of a structure, the more easily it can be stretched. – These principles apply to an analysis of arterial blood pressure. – The contraction of the ventricles ejects blood into the arteries during systole. – If a precisely equal quantity of blood were to simultaneously drain out of the arteries into the arterioles during systole, the total volume of blood in the arteries would remain constant and arterial pressure would not change. Such is not the case, however. Therefore, the arterial pressure does not decrease to zero. The Vascular System: Arteries Arterial Blood Pressure The aortic pressure pattern shown in Figure a is typical of the pressure changes that occur in all the large systemic arteries. The difference between systolic pressure and diastolic pressure (120-80 = 40 mmHg in the example) is called the pulse pressure. – The most important factors determining the magnitude of the pulse pressure are (1) stroke volume, (2) speed of ejection of the stroke volume, and (3) arterial compliance. – Specifically, at a typical resting heart rate it is approximately equal to the diastolic pressure plus one- third of the pulse pressure: At an elevated heart rate, the amount of time spent in diastole MAP = DP + 1/3 (SP – DP) is reduced more than the amount of time spent in systole. How would you estimate the mean arterial blood pressure at a Thus, in Figure a , MAP = 80 + 1/3(40) = 93 mmHg heart rate elevated to the point at which the times spent in systole and diastole are roughly equal? The Vascular System: Arteries Measurement of Systemic Arterial Pressure The Vascular System: Arterioles The arterioles play two major roles. – (1) The arterioles in individual organs are responsible for determining the relative blood flows to those organs at any given mean arterial pressure. – (2) The arterioles, all together, are the major factor in determining mean arterial pressure itself. Figure illustrates the major principles of bloodflow distribution in terms of a simple model, a fluid-filled tank with a series of compressible outflow tubes. Physical model of the relationship between arterial pressure, arteriolar What determines the rate of flow through each radius in different organs, and blood-flow distribution. In (a), blood flow is high through tube 2 and low through tube 3, whereas just the exit tube? opposite is true for (b). This shift in blood flow was achieved by – As stated previously, flow ( F ) is a function constricting tube 2 and dilating tube 3. of the pressure gradient (Δ P ) and the Assuming the reservoir is refilled at a constant rate, how would the resistance to flow ( R ): F = Δ P/R flows shown in (b) be different if tube 2 remained the same as it was in condition (a)? The Vascular System: Arterioles The arteries branch within each organ into progressively smaller arteries, which then branch into arterioles. The smallest arteries are narrow enough to offer significant resistance to flow, but the still narrower arterioles are the major sites of resistance in the vascular tree and are therefore analogous to the outflow tubes in the model. This explains the large decrease in mean pressure—from about 90 mmHg to 35 mmHg—as blood flows through the arterioles. Pulse pressure also decreases in the arterioles, so flow is much less pulsatile in downstream capillaries, venules, and veins. Like the model’s outflow tubes, the arteriolar radii in individual organs are subject to independent adjustment. The blood flow ( F ) through any organ is represented by the following equation: – F (organ) = (MAP – Venous pressure)/Resistance (organ) – Venous pressure is normally close to zero, so we may write F(organ) = MAP/Resistance (organ) The Vascular System: Arterioles Local Controls The term local controls denotes mechanisms independent of nerves or hormones by which organs and tissues alter their own arteriolar resistances, thereby self-regulating their blood flows. – This includes changes caused by autocrine and paracrine agents. – This self-regulation is apparent in phenomena such as active hyperemia, flow autoregulation, reactive hyperemia, and local response to injury. The Vascular System: Arterioles Local Controls Active Hyperemia Most organs and tissues manifest an increased blood flow ( hyperemia ) when their metabolic activity is increased ( Figure a ); this is termed active hyperemia. A number of other chemical factors increase when metabolism increases, including: – 1. carbon dioxide, an end product of oxidative metabolism; – 2. hydrogen ions (decrease in pH), for example, from lactic acid; – 3. adenosine, a breakdown product of ATP; – 4. K+ ions, accumulated from repeated action potential repolarization; – 5. eicosanoids, breakdown products of membrane phospholipids; – 6. osmotically active products from the breakdown of high-molecular-weight substances; – 7. bradykinin , a peptide generated locally from a circulating protein called kininogen by the action of an enzyme, kallikrein , secreted by active gland cells; and – 8. nitric oxide , a gas released by endothelial cells, which acts on the immediately adjacent vascular smooth muscle. The Vascular System: Arterioles Local Controls Flow Autoregulation The change in resistance is in the direction of maintaining blood flow nearly constant despite the pressure change, and is therefore termed flow autoregulation. What is the mechanism of flow autoregulation? Flow autoregulation is not limited to circumstances in which arterial pressure decreases. Although the role of local chemical factors in mediating flow autoregulation is important, another mechanism also participates in this phenomenon in certain tissues and organs. – Arteriolar smooth muscle also responds directly, by contracting when increased arterial pressure causes increased wall stretch. – Conversely, decreased stretch because of decreased arterial pressure causes this vascular smooth muscle to decrease its tone. – These direct responses of arteriolar smooth muscle to stretch are termed myogenic responses. – They are caused by changes in Ca 2+ movement into the smooth muscle cells through Ca 2+ channels in the plasma membrane. The Vascular System: Arterioles Local Controls Active Hyperemia & Flow Autoregulation An experiment is performed in which the blood flow through a single arteriole is measured. Initially, arterial pressure and flow through the arteriole are constant, but then the arterial pressure is experimentally increased and maintained at a higher level. How will blood flow through the arteriole change in the minutes that follow the increase in arterial pressure? The Vascular System: Arterioles Local Controls Reactive Hyperemia – When an organ or tissue has had its blood supply completely occluded, a profound transient increase in its blood flow occurs if flow is reestablished. – This phenomenon, known as reactive hyperemia , is essentially an extreme form of flow autoregulation. – During the period of no blood flow, the arterioles in the affected organ or tissue dilate, owing to the local factors described previously. – As soon as the occlusion to arterial flow is removed, blood flow increases greatly through these wide-open arterioles. – This effect can be demonstrated by wrapping a string tightly around the base of your finger for 1–2 minutes. – When it is removed, your finger will turn bright red due to the increase in blood flow. Response to Injury – Tissue injury causes eicosanoids and a variety of other substances to be released locally from cells or generated from plasma precursors. – These substances make arteriolar smooth muscle relax and cause vasodilation in an injured area. – This phenomenon, a part of the general process known as inflammation. The Vascular System: Arterioles Extrinsic Controls Sympathetic Neurons – Most arterioles are richly innervated by sympathetic postganglionic neurons. – Control of the sympathetic neurons to arterioles can also be used to produce vasodilation. – In contrast to active hyperemia and flow autoregulation, the primary functions of sympathetic neurons to blood vessels are concerned not with the coordination of local metabolic needs and blood flow but with reflexes that serve whole-body needs. Parasympathetic Neurons Noncholinergic, Nonadrenergic, Autonomic Neurons – there is a population of autonomic postganglionic neurons that are referred to as noncholinergic, nonadrenergic neurons because they release neither acetylcholine nor norepinephrine. Instead, they release other vasodilator substances—nitric oxide, in particular. The Vascular System: Arterioles Extrinsic Controls Hormones The Vascular System: Arterioles Endothelial Cells and Vascular Smooth Muscle The Vascular System: Arterioles Arteriolar Control in Specific Organs. The importance of local and reflex controls varies from organ to organ. – Heart High intrinsic tone; oxygen extraction is very high at rest, so flow must increase when oxygen consumption increases if adequate oxygen supply is to be maintained. Controlled mainly by local metabolic factors, particularly adenosine, and flow autoregulation; direct sympathetic influences are minor and normally overridden by local factors. During systole, aortic semilunar cusps block the entrances to the coronary arteries, and vessels within the muscle wall are compressed; therefore, coronary flow occurs mainly during diastole. – Skeletal Muscle Controlled by local metabolic factors during exercise. Sympathetic activation causes vasoconstriction (mediated by a-adrenergic receptors) in reflex response to decreased arterial pressure. Epinephrine causes vasodilation via b2-adrenergic receptors when present in low concentration, and vasoconstriction via a-adrenergic receptors when present in high concentration. The Vascular System: Arterioles Arteriolar Control in Specific Organs. The importance of local and reflex controls varies from organ to organ. – GI Tract, Spleen, Pancreas, and Liver (“Splanchnic Organs”) Actually two capillary beds partially in series with each other; blood from the capillaries of the GI tract, spleen, and pancreas flows via the portal vein to the liver. In addition, the liver receives a separate arterial blood supply. Sympathetic activation causes vasoconstriction, mediated by a-adrenergic receptors, in reflex response to decreased arterial pressure and during stress. In addition, venous constriction causes displacement of a large volume of blood from the liver to the veins of the thorax. Increased blood flow occurs following ingestion of a meal and is mediated by local metabolic factors, neurons, and hormones secreted by the GI tract. – Kidneys Flow autoregulation is a major factor. Sympathetic stimulation causes vasoconstriction, mediated by a-adrenergic receptors, in reflex response to decreased arterial pressure and during stress. Angiotensin II is also a major vasoconstrictor. These reflexes help conserve sodium and water. The Vascular System: Arterioles Arteriolar Control in Specific Organs. The importance of local and reflex controls varies from organ to organ. – Brain Excellent flow autoregulation. Distribution of blood within the brain is controlled by local metabolic factors. Vasodilation occurs in response to increased concentration of carbon dioxide in arterial blood. Influenced relatively little by the autonomic nervous system. – Skin Controlled mainly by sympathetic nerves, mediated by a-adrenergic receptors; reflex vasoconstriction occurs in response to decreased arterial pressure and cold, whereas vasodilation occurs in response to heat. Substances released from sweat glands and noncholinergic, nonadrenergic neurons also cause vasodilation. Venous plexus contains large volumes of blood, which contributes to skin color. – Lungs Very low resistance compared to systemic circulation. Controlled mainly by gravitational forces and passive physical forces within the lung. Constriction mediated by local factors in response to low oxygen concentration—just the opposite of what occurs in the systemic circulation. The Vascular System: Capillaries At any given moment, approximately 5% of the total circulating blood is flowing through the capillaries. The essential role of capillaries in tissue function has stimulated many questions concerning how capillaries develop and grow ( angiogenesis ). Anatomy of the Capillary Network The Vascular System: Capillaries Anatomy of the Capillary Network The Vascular System: Capillaries Velocity of Capillary Blood Flow The Vascular System: Capillaries Diffusion Across the Capillary Wall: Exchanges of Nutrients and Metabolic End Products The extremely slow forward movement of blood through the capillaries maximizes the time for substance exchange across the capillary wall. Three basic mechanisms allow substances to move between the interstitial fluid and the plasma: diffusion, vesicle transport, and bulk flow. Mediated transport constitutes a fourth mechanism in the capillaries of some tissues, including the brain. Bulk Flow Across the Capillary Wall: Distribution of the Extracellular Fluid The Vascular System: Veins Distribution of the total blood volume in different parts of the Blood flows from capillaries into venules and then into cardiovascular system. veins. The veins are the last set of tubes through which blood flows on its way back to the heart. In addition to their function as low-resistance conduits, the veins perform a second important function: Their diameters are reflexively altered in response to changes in blood volume, thereby maintaining peripheral venous pressure and venous return to the heart. Determinants of Venous Pressure The factors determining pressure in any elastic tube are the volume of fluid within it and the compliance of its walls. The Vascular System: Veins Determinants of Venous Pressure The walls of the veins contain smooth muscle innervated by sympathetic neurons. During skeletal muscle contraction? The respiratory pump is somewhat more difficult to visualize. The Vascular System: Veins Determinants of Venous Pressure Figure shows the typical distribution of blood in a normal, resting individual. How would the percentages change during vigorous exercise? The Vascular System: The Lymphatic System The lymphatic system is a network of small organs (lymph nodes) and tubes ( lymphatic vessels or simply “lymphatics”) through which lymph —a fluid derived from interstitial fluid—flows. The Vascular System: The Lymphatic System Mechanism of Lymph Flow – In large part, the lymphatic vessels beyond the lymphatic capillaries propel the lymph within them by their own contractions. – The lymphatic vessel smooth muscle is responsive to stretch, so when no interstitial fluid accumulates and, therefore, no lymph enters the lymphatics, the smooth muscle is inactive. – In addition, the smooth muscle of the lymphatic vessels is innervated by sympathetic neurons, and excitation of these neurons in various physiological states such as exercise may contribute to increased lymph flow. Integration of Cardiovascular Function: Regulation of Systemic Arterial Pressure The mean systemic arterial pressure is the arithmetic product of two factors: – (1) the cardiac output and – (2) the total peripheral resistance ( TPR ) , which is the combined resistance to flow of all the systemic blood vessels. Integration of Cardiovascular Function: Regulation of Systemic Arterial Pressure Integration of Cardiovascular Function: Regulation of Systemic Arterial Pressure During exercise, the skeletal muscle arterioles dilate, thereby decreasing resistance. Bleeding that results in significant blood loss ( hemorrhage ) leads to a decrease in mean arterial pressure. In the short term—seconds to hours—these homeostatic adjustments to mean arterial pressure are brought about by reflexes called the baroreceptor reflexes. – They utilize mainly changes in the activity of the autonomic neurons supplying the heart and blood vessels, as well as changes in the secretion of the hormones that influence these structures (epinephrine, angiotensin II, and vasopressin). Over longer time spans, the baroreceptor reflexes become less important and factors controlling blood volume play a dominant role in determining blood pressure. Integration of Cardiovascular Function: Regulation of Systemic Arterial Pressure Baroreceptor Reflexes Arterial Baroreceptors When you first stand up after getting out Note in part (a) that the normal resting value on this of bed, how does the pressure detected pressure–frequency curve is on the steepest, center by the carotid baroreceptors change? part of the curve. What might be the physiological significance of this? Integration of Cardiovascular Function: Regulation of Systemic Arterial Pressure Baroreceptor Reflexes The Medullary Cardiovascular Center The primary integrating center for the baroreceptor reflexes is a diffuse network of highly interconnected neurons called the medullary cardiovascular center , located in the medulla oblongata. Integration of Cardiovascular Function: Regulation of Systemic Arterial Pressure Baroreceptor Reflexes Operation of the Arterial Baroreceptor Reflex Other Baroreceptors The large systemic veins, the pulmonary vessels, and the walls of the heart also contain baroreceptors, most of which function in a manner analogous to the arterial baroreceptors. By keeping brain cardiovascular control centers constantly informed about changes in the systemic venous, pulmonary, atrial, and ventricular pressures, these other baroreceptors provide a further degree of regulatory sensitivity. In essence, they contribute a feedforward component of arterial pressure control. For example, a slight decrease in ventricular pressure reflexively increases the activity of the sympathetic nervous system even before the change decreases cardiac output and arterial pressure enough to be detected by the arterial baroreceptors. Integration of Cardiovascular Function: Regulation of Systemic Arterial Pressure Blood Volume and Long-Term Regulation of Arterial Pressure Integration of Cardiovascular Function: Regulation of Systemic Arterial Pressure Other Cardiovascular Reflexes and Responses – Stimuli acting upon receptors other than baroreceptors can initiate reflexes that cause changes in arterial pressure. For example, the following stimuli all cause an increase in blood pressure. – Many physiological states such as eating and sexual activity are also associated with changes in blood pressure. – Mood also has a significant effect on blood pressure, which tends to be lower when people report that they are happy than when they are angry or anxious. – An important clinical situation involving reflexes that regulate blood pressure is Cushing’s phenomenon (not to be confused with Cushing’s syndrome and disease, which are endocrine diseases).