Carbohydrates.pdf
Document Details
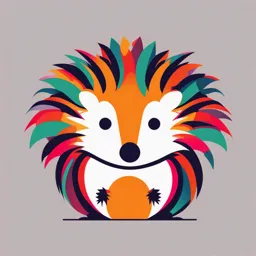
Uploaded by IdealSalamander
Tags
Full Transcript
Macronutrients CARBOHYDRATES Contents 1. Introduction ........................................................................................................................3 2. Monosaccharides ........................................................................................................
Macronutrients CARBOHYDRATES Contents 1. Introduction ........................................................................................................................3 2. Monosaccharides ................................................................................................................3 2.1. Classifications and basic nomenclature .................................................................. 3 2.1.1. Chiral centres: isomerism ....................................................................................... 4 2.1.2. Optical activity ........................................................................................................ 6 2.2. Fischer projections .................................................................................................. 6 2.3. D-L Classification ..................................................................................................... 7 2.3.1. Aldoses .................................................................................................................... 8 2.3.2. Ketoses .................................................................................................................... 9 2.4. Ring structure of monosaccharides ...................................................................... 10 2.4.1. Mutarotation......................................................................................................... 10 2.4.2. Cycle formation and hemiacetal formation .......................................................... 11 2.4.3. Haworth projections ............................................................................................. 12 2.5. Properties and Reactions of monosaccharides..................................................... 14 2.5.1. Strong reducing agents ......................................................................................... 14 2.5.1.1. Fehling’s test ......................................................................................................... 14 2.5.1.2. Barfoed’s test ........................................................................................................ 15 2.5.2. Other colorimetric tests ........................................................................................ 16 2.5.2.1. Seliwanoff’s reagent ............................................................................................. 16 2.5.2.2. Aniline Acetate paper ........................................................................................... 16 2.5.3. Osazone formation................................................................................................ 17 2.5.4. Reduction to alditols ............................................................................................. 17 2.5.5. Glycoside formation .............................................................................................. 18 3. Disaccharides ................................................................................................................... 18 3.1. Properties .............................................................................................................. 18 3.2. Maltose ................................................................................................................. 19 3.3. Lactose.................................................................................................................. 21 3.4. 3.5. CARBOHYDRATES Sucrose ................................................................................................................. 22 Trehalose.............................................................................................................. 22 Page 1 of 27 Macronutrients 4. Polysaccharides ................................................................................................................ 23 4.1. Pentosans – Hexosans: A few examples ............................................................... 23 4.2. Properties .............................................................................................................. 23 4.3. 4.3.1. Starch.................................................................................................................... 23 4.3.2. Amylopectin .......................................................................................................... 24 4.3.3. Enzymatic hydrolysis of Starch.............................................................................. 25 4.3.4. Glycogen................................................................................................................ 26 4.4. Cellulose ................................................................................................................ 26 CARBOHYDRATES Amylose................................................................................................................. 24 Page 2 of 27 Macronutrients 1. Introduction Carbohydrates are a very important and near ubiquitous class of organic compounds originating from the plant kingdom by photosynthesis, and as such found largely in plants either as structural material (cellulose in wood), to energy storage (starch in grains) to simple sugars in fruits (fructose) and tubers (sucrose in beets). Although animals cannot synthesise carbohydrates, they are also found in the animal kingdom as free glucose in blood or as in-cell storage in the form of glycogen, but they are very often found attached to other entities such as lipids (glycolipids) or proteins (glycoproteins on the cell membranes). One way to characterise these carbohydrates is to differentiate them as sugars because of their sweetness (from the Greek σάκχαρον, sakcharon) and comprise the monosaccharides and disaccharides, and the more complex carbohydrates such as the oligosaccharides and polysaccharides that are not sweet but are condensation products of monosaccharides. 2. Monosaccharides Carbohydrate classification • • Sugars – Simple sugars (monosaccharides) • (CH2O)n n=3, 4, 5, 6, 7 • Examples: ribose, glucose, fructose – Oligosaccharides (di- tri- tetra- etc.) – Examples: the disaccharides sucrose, lactose, maltose – Examples: the oligosaccharide inulin Polysaccharides – High MW Polymers (100s or 1000s of monosaccharide units) • E.g., amylose and amylopectin in starch 2.1.Classifications and basic nomenclature All the monosaccharide compounds are characterised by a chemical formula (CH2O)n as if each Carbon atom in these molecules was bound to one molecule of water, hence the term hydrates of carbon, or carbohydrates. In fact, although this simple molecular formula holds, the need for one unsaturated carbonyl functional group in the molecule gives two classes of compounds the aldoses (with an aldehyde in position 1) or the ketoses (with a ketone in position 2). In the simplest case of monosaccharides with three carbons, this gives either glyceraldehyde or dihydroxyacetone. CARBOHYDRATES Page 3 of 27 Macronutrients Aldose/ketose classification (CH2O)n with n=3, trioses 2 classes of compounds Glyceraldehyde (aldotriose) Dihydroxyacetone (ketotriose) Depending on the number of carbons in the molecule (or the value of n in the formula (CH2O)n) the monosaccharides are then called as trioses (n=3), tetroses (n=4), pentoses (n=5), hexoses (n=6), etc. 2.1.1. Chiral centres: isomerism One important characteristics of carbohydrates is that they contain chiral carbons (denoted C*) or carbon atoms with four different substituents attached to them. n=3 trioses Aldotriose (Glyceraldehyde) CARBOHYDRATES Examples of aldoses n=4 n=5 tetroses pentoses aldotetrose (Erythreose) Page 4 of 27 aldopentose (Ribose) n=6 hexoses aldohexose (Allose) Macronutrients This asymmetry in the carbon substitution will give rise to two stereoisomers called enantiomers that have a non-super-imposable mirror image. mirror B X C* A A Y X C* B Y B X C* A Y Although these two molecules are very similar – they share the same chemical (e.g., reactivity, etc.) and physical (e.g., melting point, etc.) properties, they are not identical as they differ in one property, their optical activity. Biologically this difference is very important, as most cellular receptors are also chiral and will only recognise one enantiomer as the bioactive molecule, the other being either redundant or possibly toxic. As the number of chiral centres or asymmetric carbons C* increases, so do the number of possible stereoisomers. As a general rule, for n asymmetric carbons, there will be 2n stereoisomers and therefore 2(n-1) enantiomeric pairs. In the simplest case of Glyceraldehyde (1 C* asymmetric carbon) that gives: CHO C H HO CHO CH2OH HOH2C C H OH chiral centres or asymmetric carbons C* (Definition): Chiral centres are tetrahedral atoms (usually carbons) that have four different substituents. Each chiral centre in a molecule will be either R or S. As noted above, molecules with a single chiral centre are chiral. CARBOHYDRATES Page 5 of 27 Macronutrients 2.1.2. Optical activity In solution, these enantiomers have different optical activity. Each enantiomer causes the plane of a monochromatic polarised light to rotate by a specific angle, equal but reversed for both enantiomers in an enantiomeric pair. Polarimeter From: Organic and biological chemistry / John R. Holum This optical property is characterised by the specific rotation of the compound: [α ] T λ = α obs l ⋅c T: temperature, λ: wavelength, αobs: observed rotation, l,: cell length in dm, c: sample concentration in g/mL In the case of glyceraldehyde, a 1g/mL solution of one enantiomer will rotate light (D line of Sodium, λ =570 nm) to the right by 14° for a cell length of 10dm at 20°C, and this enantiomer is called dextrogyre, noted (+). The other enantiomer will rotate light 14° to the left, and is called levogyre, noted (-). An equimolar mixture of these two enantiomers will not rotate the light, as their optical activity cancel each other, and this particular mixture will be called racemic mixture. 2.2. Fischer projections When the carbohydrates contain 2 or more asymmetric carbons, the representation of these carbohydrates becomes cumbersome and the common use has been to follow the Fischer representation, with the D, L nomenclature. Page 6 of 27 CARBOHYDRATES Macronutrients This two-dimensional representation predates the Cahn-Ingold-Prelog (R, S) absolute representation and it is still used today for carbohydrates and amino acids. The rules are as follow: • • • • • The asymmetric carbon is placed in the projection plan. The longest carbon chain is vertical and behind the plan. The vertical carbon chain is oriented with C1 at the top in the case of aldose or with the carbonyl group in C2 in the case of ketoses. The two other substituents, which are in front of the projection plan, are represented as horizontal lines. D and L nomenclature is in relation with the penultimate carbon along the chain (n-1) with the D series having the –OH group on the right and the L series having the –OH group on the left. CHO C H HO CHO CHO CH2OH H C OH H OH CH2OH CH2OH (D) (R) CHO CHO CHO HOH2C C C HO H OH C H CH2OH (S) HO C H CH2OH (L) Fischer projection of (R) and (S) Glyceraldehyde 2.3.D-L Classification All monosaccharides made from D-glyceraldehyde by asymmetric synthesis are the Dseries sugars. All have the last (furthest from -CHO) -OH group on the right. No relationship to optical activity!!! CARBOHYDRATES Page 7 of 27 Macronutrients 2.3.1. Aldoses In the case of aldotetroses (4 carbons, 2 chiral centres, aldehyde in position 1), the 22= 4 stereoisomers are represented in Fischer projection as follows: Stereoisomers of aldotetrose mirror H (E) C O H C OH H C OH H Enantiomers H (T) C Diastereoisomers H (2S, 3S) H Enantiomers H C OH C O H C OH HO C CH2OH H (T') CH2OH (2S, 3R) (2R, 3S) L series D series • • • (E') CH2OH O HO C H O HO C H HO C CH2OH (2R, 3R) C E and E’ are enantiomers. So are T and T’ E and E’ are diastereoisomers of T and T’ E and T are epimers. So too are E and T’ The two pairs of enantiomers Erythrose (E, E’) and Threose (T, T’) are diastereoisomers of each other. As such they do not share the same chemical characteristics and can be separated, by crystallisation for example. Because these diastereoisomers differ by the configuration around 1 carbon only, they are called epimers. The D-L classification refers to the position of the penultimate hydroxyl group on the chain (-OH on carbon 3 for Threose and Erythreose above) so that both E and T are of configuration D, while their enantiomers E’ and T’ are of configuration L. But this classification has no bearing on the direction of the optical rotation of these sugars. Both (D) Threose and (D) Erythreose rotate the light to the left and are levogyre (-). CARBOHYDRATES Page 8 of 27 Macronutrients In the case of aldopentose, this classification will lead to 23=8 stereoisomers, or 4 enantiomeric pairs, the D series of which is being as follows: CHO H OH CHO OH H CHO H HO H OH H OH HO H OH H OH H CH2OH CH2OH D(-) ribose H CHO HO H HO OH H OH H CH2OH D(-) arabinose CH2OH D(+) xylose D(-) lyxose In the case of aldohexose, 24=16 stereoisomers are possible. The main 3 D enantiomers are represented below: 1 CHO 2 OH H HO 3 H 4 OH H 5 OH 6 H CH2OH D(+) Glucose CHO 2 HO H HO H CHO H OH HO H OH HO H OH H H 4 H OH CH2OH CH2OH D(+) Mannose D(+) Galactose Because glucose and mannose have a difference in configuration around only 1 *C, they are epimers (C2 epimers). The same is true for glucose and galactose (C4 epimers). But mannose and galactose are not epimers, they differ both in configuration around C2 and C4, and are therefore diastereoisomers. 2.3.2. Ketoses In the case of ketoses, the D-L classification is also defined by the position of the penultimate hydroxyl group on the carbon chain furthest away from the ketone function and gives rise to the D series as follows: CARBOHYDRATES Page 9 of 27 Macronutrients 1 CH2OH H 2 O 3 OH 4 44 C C=> *C =>1 1*C CH2OH 1 1 CH2OH CH2OH 2 H 3 H 4 5 O 5C 5C =>=> 2 2*C *C OH 2 O HO 3 H H 4 OH OH CH2OH CH2OH 1 1 CH2OH 2 H 3 H 4 H 5 6 O OH HO 3 H 4 H 5 OH OH CH2OH 6 OH CH2OH CH2OH O H 1 1 CH2OH 2 5 2 =>33*C 66CC=> *C OH H HO H CH2OH 3 4 5 6 2 O HO 3 H HO 4 H H 5 OH O OH H OH CH2OH 6 CH2OH D (-) Fructose 2.4.Ring structure of monosaccharides 2.4.1. Mutarotation Crystallisation of glucose in different solvents (ethanol, pyrimidine) leads not only to one compound but 2, with different melting points and optical properties. One crystal, named α-D-glucose, has a melting point of 146°C and a specific rotation of +112° while the other, named β-D-Glucose, has a melting point of 152°C and a specific rotation of +19°. When either of these crystals is dissolved in water, the optical rotation of the solution will change with time to settle after a few hours to 52.5°. This phenomenon is called mutarotation. CARBOHYDRATES Page 10 of 27 Macronutrients [α] a-D-Glucose b-D-Glucose Time 2.4.2. Cycle formation and hemiacetal formation An aldehyde and an alcohol react to form a hemiacetal. In case both functions occur on the same molecule, this reaction will result in a cyclic hemiacetal as represented below: O R H + H O O R' H O R' R H In the case of glucose, we can envisage the reaction of the aldehyde on carbon 1 with the hydroxyl group on carbon 5 to form the C1-C5 hemiacetal. H C 1 OH 2 OH H H C1 O 2 OH H HO 3 H H 4 OH H 5 OH 6 CH2OH HO 3 H 4 H 5 H OH O 36% 6 CH2OH HO C 1 H 2 OH H HO 3 H 4 H 5 6 H OH CH2OH CARBOHYDRATES α-D-Glucose Page 11 of 27 β-D-Glucose O 64% Macronutrients This cyclic hemiacetal formation makes carbon C1 asymmetric and leads to two anomers, α-D-glucose and β-D-glucose. These two anomers differ only on the carbon that was the carbonyl carbon in the open chain and are therefore not enantiomers but epimers on C1. This C1 carbon, the only one bonded to two oxygens, is called the anomeric carbon. In a neutral (pH7) aqueous solution, the open-chain conformation is in equilibrium with the two cyclic conformations, albeit in a very small percentage (<1%), while there is almost twice β-D-glucose (64%) than α-D-glucose (36%). This equilibrium is pH sensitive. In alkaline conditions (pH>7), the predominant form is the linear one (99%). 2.4.3. Haworth projections Fischer projections are not the best way to represent a cyclic structure of a monosaccharide, because of the elongated C-O-C bond. A more elegant representation is the Haworth projection, where the sugar is viewed edge-on as below: H C 1 OH 2 H OH HO 3 H 4 H 5 H OH 6 H 5 H 4 OH O OH 3 H 6 CH2OH Fischer projection OH O H 2 H 1 OH OH Howarth projection α-D-Glucose α-D-Glucopyranose The cycle is then perpendicular to the page, with the ring oxygen typically in back righthand corner so that the anomeric carbon is displayed on the right-hand side of the molecule. Functional groups which are on the right in the Fischer projection are down (below the cycle) in the Haworth projection, whereas groups which are on the left in the Fischer projection are up (above the cycle) in the Haworth projection. For Glucose, the conformation on carbon C5 determines the D-L series to which the sugar belongs. Therefore, in Haworth projection, the up-down position of the primary alcohol on C6 which will indicate which D-L series the sugar belongs to: D-series for CH2OH above the cycle and L-series for CH2OH below the cycle. By analogy, all other sugars will follow this notation. Because the six atoms cycle formed by the C1-C5 hemiacetal is reminiscent of a pyran molecule, these cyclic sugars are called pyranoses. Consequently, α-D-Glucose will also be called α-D-Glucopyranose. CARBOHYDRATES Page 12 of 27 Macronutrients In the case of D-Fructose (ketohexose), reaction of the C5 hydroxyl group with the ketone carbonyl in position C2 will form a 5 atoms ring structure reminiscent of a furan and these cyclic sugars will be called furanoses. 1 CH2OH 2 O HO 3 H 4 OH H 5 OH HO H 6 6 5 C2 - C5 H H hemiketal 1 O 2 HO 3 OH 4 OH CH2OH OH H Notice than in fructose, the anomeric carbon is not C1 but C2. Cycle formation in the case of aldopentoses (ribose, deoxyribose) leads to C1-C5 cycle formation (ribopyranose) and C1-C4 cycle formation (ribofuranose). CHO H OH H OH H OH HO O O OH HO OH CH2OH OH OH OH Ribopyranose ~80% Ribofuranose ~20% D (-) Ribose <1% OH Although the furanose ring is well represented by the Howarth projection as it is near planar, this planar projection is quite misleading in the case of pyranoses which are preferentially in chair conformation. H OH OH H H OH O OH H HO H HO H H OH Howarth projection OH H 3D representation β-D-Glucopyranose CARBOHYDRATES OH HO H H O Page 13 of 27 Macronutrients In the case of β-D-glucopyranose above, the pseudo 3-D chair representation clearly indicates that all large hydroxyl groups on the six-atom ring are in equatorial position, away from the ring and one another, thereby minimising steric hindrance. On the other hand, all the axial positions are occupied by Hydrogen atoms (not represented for simplification) which are small and therefore, put the cycle under little steric strain. No other sugar exists in this strain-free conformation, which explains why β-D-Glucopyranose is the most stable aldohexose and may explain why it is also the most abundant. In the case of α-D-glucopyranose, the anomeric carbon will have the large hydroxyl group in axial position. This single change with β-D-glucopyranose will be responsible for enough steric strain to destabilise this conformation and will lead to β-DGlucopyranose being more stable and therefore predominates at room temperature in water. OH OH HO CH2 HO O OH HO HO CH2 H HO HO H β-D-Glucopyranose 64% O OH α-D-Glucopyranose 36% 2.5. Properties and Reactions of monosaccharides All monosaccharides are white crystalline solids, highly soluble in water but insoluble in organic solvents such as hexane or chloroform. Most have sweet taste. Like all organic aldehydes and hydroxyketones, they are easily oxidised to carboxylic acids and are therefore strong reducing agents. 2.5.1. Strong reducing agents Because of this characteristic, monosaccharides are easily oxidised by Tollen’s reagent (ammoniacal silver nitrate) H C O R 2.5.1.1. CARBOHYDRATES + Ag+ NO3- + NH4OH O C R Fehling’s test Page 14 of 27 O Ag (0) Macronutrients They also reduce Fehling’s solution (alkaline solution of Cu2+) to yield insoluble red cuprous oxide. This reaction is unique to reducing sugars. H C O +2 Cu2+ +4 OH- HO C O R R + Cu2O + 2 H2O Cu2+ Cu2O Cupric ions Cuprous oxide Deep blue Red 2.5.1.2. Barfoed’s test In acidic condition, monosaccharide reduce Barfoed’s solution (acidic solution of Cu2+) to yield insoluble red cuprous oxide. This reaction is unique to monosaccharides, not disaccharides. H C O +2 R Cu2+ + 7 H2O O C R O + Cu2O + 5 H3O+ Cu2+ Cu2O Cupric ions Cuprous oxide Deep blue Red The reaction of Fructose, a ketose, with Fehling’s reagent is not obvious, due to the absence of aldehyde group on the molecule. The solution lies in the fact that in alkaline solutions, ketones can be converted to aldehyde by keto-enol tautomerism. In the case of fructose, this gives: CARBOHYDRATES Page 15 of 27 Macronutrients H OH H O HO OH- H H OH H OH H2O OH HO H H OH H OH CH2OH H OH H2O OH- H HO H O OH HO H H HO H O H OH H OH H OH H OH CH2OH CH2OH Fructose Glucose Mannose So that, in alkaline conditions, fructose is in equilibrium with both glucose and mannose, and therefore can react with Fehling’s solution. 2.5.2. Other colorimetric tests 2.5.2.1. Seliwanoff’s reagent Seliwanoff’s test is a useful test that distinguishes ketose from aldoses. This reagent is a solution of the phenolic compound resorcinol in concentrated HCl. When heated in the reagent, the dehydration products of ketohexoses but not aldohexoses give a red colour. Positive Seliwanoff with Fructose Negative Seliwanoff with Glucose 2.5.2.2. Aniline Acetate paper On heating with acids, pentoses yield furfural which is steam volatile. Its vapour can condense with aniline acetate (elimination of H2O) to give a red coloured product. The hydroxymethyl furfural from hexoses is much less volatile so very little vapour reaches the test paper and consequently the test is negative for hexoses. O CARBOHYDRATES C H H2C O HO O C H O Furfural Hydroxymethyl furfural (From pentose ring) (From hexose ring) Page 16 of 27 CH2OH Macronutrients 2.5.3. Osazone formation Aldoses and ketoses react with Phenylhydrazine to form an insoluble osazone. 1 CHO 2 OH H HO 3 H 4 OH H 5 OH H N H 6 H N 1 HC N + H N ∆ H Phenylhydrazine CH2OH 2 N HO 3 H H 4 OH H 5 OH 6 N H C6H5 C6H5 CH2OH Because the configuration in C2 is lost during this reaction, C2 epimers such as Glucose and mannose will form the same osazone. As the same 2 carbons react in the case of ketoses, glucose and fructose will also form the same osazone. On the opposite, glucose and galactose, which are C4 epimers, will have different osazones. Glucose, Fructose and Mannose osazone 2.5.4. Galactose osazone Reduction to alditols Reduction of aldoses and ketoses give a polyalcohol known as alditol. But although the reduction of an aldose gives one alditol, the reduction of a ketose gives 2 alditols due to the creation of a new asymmetric centre in C2. 1 HO 2 H HO 3 H H 4 H 5 6 OH OH CH2OH D-Mannitol CARBOHYDRATES 1 1 CH2OH CH2OH 2 O NaBH4 H3O+ HO 3 H 4 H 5 6 H OH OH CH2OH D-Fructose Page 17 of 27 H NaBH4 H3O+ CH2OH 2 OH HO 3 H H 4 OH H 5 OH 6 CH2OH D-Glucitol Macronutrients So, the reduction of Fructose will give 2 alditols, D-Mannitol, also formed from the reduction of D-Mannose, and D-Glucitol, formed from the reduction of D-Glucose. 2.5.5. Glycoside formation Monosaccharides can link to an alcohol via the reactive -OH group present on the anomeric carbon (hemi-acetal) to form the corresponding acetal. O H 1 OH O H3O+ 1 HO R α-sugar H O R + O 1 H α-glycoside β-glycoside Because this reaction occurs in acidic conditions, it results in both anomers being produced. Once formed, these glycosides are acetals and therefore not in equilibrium with their open-chain aldehyde or ketone conformation. This change will prevent glycosides from ring opening and are therefore non-reducing sugars and will exhibit no mutarotation. 3. Disaccharides When the hemi-acetal group of a monosaccharide reacts with an alcohol group from another monosaccharide, the glycoside formed is called a disaccharide. 3.1. Properties All disaccharides are sweet, crystallisable solids, highly soluble in water to give optically active solutions and insoluble in non-polar organic solvents such as hexane and chloroform. Two types of disaccharides can be distinguished: • Reducing disaccharides such as maltose and lactose These disaccharides will exhibit mutarotation, indicating that they possess a hemi-acetal group on an anomeric carbon, and will react positively with Fehling’s test (as they are reducing), negatively with Barfoed Test (not monosaccharides) and will react with phenylhydrazine to form osazones. Maltose osazone CARBOHYDRATES Page 18 of 27 O Lactose osazone R Macronutrients Non-reducing disaccharides such as sucrose and trehalose These non-reducing disaccharides do not mutarotate, do not react with Fehling or Barfoed reagents and do not form osazones. • 3.2. Maltose Maltose does not occur freely in nature but is the product of hydrolysis of starch from cereal grains and malt, hence its name. Upon further hydrolysis in strong acid, Maltose only gives Glucose. If we look at the condensation of two α-Glucose, we get: 6 OH H 5 H 4 OH OH 6 O H 2 3 H H 1 OH OH H 5 H 4 OH HO OH 6 O H 2 3 H H H 5 H 4 OH - H2O 1 OH OH OH O 1 H H OH H 2 3 OH 6 O H 5 H 4 OH O 1 H 2 3 OH H H OH OH α-1-4 Glycosidic link α-Glucose α-Maltose α-Glucose The glycosidic link formed is called α-1-4-glycosidic link because it links the anomeric carbon C1 of the first Glucose unit (here in conformation α) with carbon C4 of the second Glucose unit. The α−β conformation of the resulting Maltose is dictated by the conformation of the anomeric carbon for the second glucose unit (here also in conformation α ) to give in this example α-Maltose. If the second glucose had a β conformation, the resulting disaccharide would be β−maltose as below: 6 H 5 H 4 OH OH 3 H OH 6 O H 2 OH H 1 OH H 5 H 4 OH HO 3 H OH 6 O H 2 OH 1 - H2O H OH H 5 H 4 OH OH 3 H OH O H 2 OH H 1 O H 5 H 4 OH 3 H α-1-4 Glycosidic link α-Glucose β-Glucose In pseudo 3D representation, this disaccharide will take the form: CARBOHYDRATES 6 Page 19 of 27 OH O OH H 1 2 H OH β-Maltose Macronutrients OH O HO OH HO OH 1 α or β-Maltose O 6 4 O 5 HO 2 3 OH OH α-1-4 Glycosidic link On the other hand, if the first glucose were in conformation β, the link would then be a β−1-4-glycosydic link and the disaccharide not maltose but cellobiose: 6 H 5 H 4 OH OH 3 H OH 6 O OH H 1 2 OH H H 5 H 4 OH HO 3 H OH 6 O H 2 H 1 - H2O OH OH H 5 H 4 OH OH 3 H OH OH 6 O H 1 2 OH H H 5 H O 4 OH 3 H H 2 H 1 OH OH β-1-4 Glycosidic link β-Glucose α-Glucose α-Cellobiose Cellobiose, like maltose, does not exist freely in nature, but it is the product of hydrolysis of cellulose. CARBOHYDRATES O Page 20 of 27 Macronutrients 3.3. Lactose Lactose is the reducing disaccharide found in milk to a concentration of circa 50g/L. The inoculation of milk with certain cultures like Lactobacillus will convert lactose to lactic acid, thereby reducing the pH of milk from 6.8 down to 4.6 causing coagulation of milk protein (casein) and is at the basis of yogurt and cheese production. CH3 H OH COOH Lactic acid Lactose is made from the condensation of one β-galactose with one glucose unit through a β-1-4 glycosidic link as below: 6 HO 5 H 4 OH H 3 H OH 6 O OH H 1 2 H OH OH H 5 H 4 OH HO 6 O H 2 3 H - H2O 1 H HO 5 H 4 OH H OH 3 H OH OH O H 1 2 H OH In pseudo 3D representation, this disaccharide will take the form: OH OH O OH 4 1 O α or β-Lactose O 6 5 HO 2 3 β-1-4 Glycosidic link CARBOHYDRATES 3 H O H 1 H 2 OH OH α-Lactose α-Glucose HO H 5 H O 4 OH β-1-4 Glycosidic link β-Galactose HO OH 6 Page 21 of 27 OH 1 OH Macronutrients 3.4. Sucrose The most abundant and important disaccharide, it occurs widely in plants, and is the reserve sugar in sugar cane (14-26% w/w) and sugar beet (13-17% w/w). Sucrose consists of a Glucose unit linked in C1 to a fructose unit in C2. As both the C1 on the Glucose and the C2 on the fructose are anomeric carbons, the resulting sucrose does not possess a hemiacetal group. This sugar is therefore non-reducing (negative Fehling Test) and does not mutarotate. 6 H 5 H OH 4 OH 3 H OH O H 2 H H HO OH 4 13 HO 1 2 OH HO H O - H2O H H 5 H 4 OH 5 6 OH α-Glucose 6 OH OH 3 H β-Fructose OH H O H HO 2 1 H 4 HO H O O 2 OH 13 H 5 6 OH α-1-2 Glycosidic link Sucrose has a specific rotation of +66.5°. Its hydrolysis results in a mixture of specific rotation of -22° as it is a 1:1 mixture of D-(+)glucose and D-(-)fructose. This light rotation change gives its name to the hydrolysed mixture: invert sugar. This procedure is undertaken on an industrialised scale because the resulting mixture is also sweeter than the starting Sucrose material because of the high sweetness of fructose. 3.5. Trehalose Trehalose is found abundantly in mushrooms, bacteria and insects. Numerous organisms accumulate this sugar in order to sustain thermal (cold) and dehydration shocks. It is a dimer of α-glucose, linked on both units with their anomeric carbon, to form a α-1-1-glycosidic link. As a result, trehalose has no hemi-acetal and is also non-reducing disaccharide. OH O HO HO OH O HO α-1-1 Glycosidic link OH OH O OH CARBOHYDRATES Page 22 of 27 OH Macronutrients 4. Polysaccharides 4.1. Pentosans – Hexosans: A few examples Polysaccharides can contain from 10 to thousand monosaccharide units linked together with glycosidic links. As with other polymers, polysaccharides can be homo-polymers if consisting of the same repeating monosaccharide unit, or hetero-polymer, if consisting of two or more repeating units. In nature, two main types of polysaccharides exist: pentosans, formed of repeating pentoses, and hexosans, made up of repeating hexoses. Pentosans are found in fibrous and gummy parts of plants, in the hemicellulose fraction of plant cell wall. Examples include xylans (made up of xyloses) which are the lignified tissues found in bran and straw, and arabinans (made up of arabinose) found in cherry gum and gum arabic. Hexosans, formed by 6 carbon monosaccharides are the more abundant polysaccharides. They include: • • • Mannans and galactans widely distributed in cell wall of fibrous tissue. Fructosans, for example in artichokes. Glucosans, by far the most abundant type of hexosans, with for example the two storage polysaccharides starch and glycogen and the plant structural polysaccharide cellulose. 4.2. Properties Polysaccharides are amorphous solids (they do not crystallise) and are quite tasteless (not sweet), either totally or poorly soluble in water. In some cases, they form colloidal aqueous solutions. Chemically, they do not reduce Fehling’s solution and do not form osazones. Some give characteristic blue complex with Iodine. 4.3. Starch Starch is a typical plant storage polysaccharide, accumulating in leaves during daylight and being translocated at night to storage organs to form up to 60% of reserve material in cereal seeds or 20% of potato tubers. In all cases, starch is found in the form of granules, whose shape and form are characteristic of the plant type. Singh et al., (2003) Potato starch granules CARBOHYDRATES Page 23 of 27 Rice starch granules Macronutrients The properties of starch are similar to those of most polysaccharides in that it forms a tasteless, white amorphous solid. Chemically, it reacts negatively to Fehling’s test, is insoluble in cold water, but form colloidal solutions in warm water. It also gives a strong blue colour with Iodine which disappear upon heating but reforms upon cooling. Starch has been found to be composed of two distinct polymers depending on the plant source: • • Amylose (0-20%) Amylopectin (80-100%) 4.3.1. Amylose The structure of amylose is a linear polymer of glucose units all linked by α 1-4 glycosidic links. This linear structure has been found to extend to thousands of units of Glucose. OH O HO O OH OH 1 4 O 6 O OH 5 HO 2 3 OH 1 O O HO OH O Three subunits of amylose 4.3.2. Amylopectin Amylopectin is a branched polymer of glucose units. The core structure is made up of a chain of glucoses linked by α 1-4 glycosidic links with a α 1-6 glycosidic branch link every 20-25 units. This molecule is very large, one of the largest naturally occurring polymer, and can be up to 200 times larger than amylose. Five subunits of amylopectin CARBOHYDRATES Page 24 of 27 Macronutrients In fact, the spacial arrangement of both amylose and amylopectin in starch granules is still a topic of research at present, although a general concensus has emerged around the picture below where molecules of amylopectin (d) are crystallised in a unique branch and stem system (c) themeselves packed within a semi-crystalline portion (b) of the granule (a). Picture taken from The molecular structures of starch components and their contribution to the architecture of starch granules by Serge Pérez and Eric Bertoft; Starch ‐ Stärke (2010) 62(8),389-420. 4.3.3. Enzymatic hydrolysis of Starch Amylases, digestive enzymes present in our digestive tract, can hydrolyse starch, first into large dextrins, then, upon further hydrolysis, into smaller dextrins and sugars such as maltose. But these amylases cannot convert the maltose into glucose, the acidic gastric conditions allow this to occur chemically. These dextrins are the product of partial hydrolysis of starch and as such comprise many molecules of various size and structure, water soluble and sweet. Chemically, they react positively with Fehling’s test. Industrially these molecules are at the basis of corn syrups and are also used as binders and adhesives. Two major types of amylases exist: • • α-amylases found in saliva and pancreatic juices, split the more central glycosidic link (endo- type hydrolysis) to liberate products of α conformation such as αdextrins, α-maltose and α-glucose. β-amylases so called because they liberate only β-maltose from the non-reducing end of the polymer (exo- type hydrolysis). But these amylases can only hydrolyse α-1-4 glycosidic links. Neither amylase can hydrolyse the α-1-6 glycosidic links at the branch points of amylopectin, so that the final product is a highly branched core known as limit dextrin. CARBOHYDRATES Page 25 of 27 Macronutrients Hydrolysis of amylopectin by β-amylase to give β-maltoses and limit dextrin 4.3.4. Glycogen Glycogen is the storage polysaccharide found in the liver and muscles of animals. Its structure is highly similar to that of amylopectin, in that it is a highly branched polymer of a core of glucose units linked by α 1-4 glycosidic links with a α 1-6 glycosidic branch link every 8-10 units. This high degree of branching is thought to be due to the high availability of many glucose units from the ends of many branches when the body needs energy. 4.4. Cellulose Cellulose is the simplest and yet the most abundant structural cell wall polysaccharide, very important as it is responsible for plant rigidity and form. It can be found in many vegetal products such as Cotton (90% cellulose), Hemp(70%), Flax (75-90%), Wood (5060%) and Straw (~40%). Cotton wool that has been treated by an alkali is 100% cellulose. This polymer is also an amorphous white solid, totally insoluble in water, and can only be dissolved using special reagents such as Schweitzer solution (Cu(OH)2 and conc. NH4OH) Upon reaction in strong and hot acidic solution, Cellulose will first hydrolyse into cellodextrins, then into cellobiose before giving Glucose. Its structure is that of a linear polymer of glucose units linked by β 1-4 glycosidic links as below: OH OH O O HO OH 4 1 O OH O 6 5 HO O 2 3 OH 1 O HO Three sub units of Cellulose CARBOHYDRATES Page 26 of 27 OH O Macronutrients In fact, it has been found that each alternate glucose unit is rotated by 180° to give the following structure with extra hydrogen bonds between glucose subunits within the same polymer (intra-molecular H bonds) which is thought to be the basis for the lack of solubility of cellulose in water. OH OH 3 O IIIIIIII HO O HO OH 1 5 O 4 OH 1 6 2 O O O IIIIIIII HO OH O OH Three subunits of Cellulose with intra-molecular H-bonds (in green) In cell walls, the cellulose polymers are organised into parallel bundles called microfibrils, where the polymer develop inter-molecular H bonds rendering these assemblies highly insoluble in water. CARBOHYDRATES Page 27 of 27