BIOL1131 Plant and Animal Biology Notes PDF
Document Details
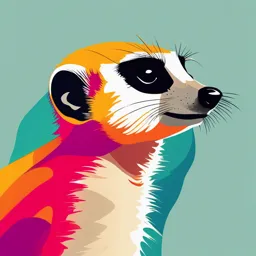
Uploaded by SparklingGraffiti755
University of Western Australia
Daria Harris
Tags
Summary
These notes cover the basics of plant and animal biology, including taxonomic classification, characteristics of different animal phyla, and animal adaptations for aquatic life. The document describes different plant and animal classification systems.
Full Transcript
lOMoARcPSD|40737841 BIOL1131 notes - All lectures Plant and Animal Biology (University of Western Australia) Scan to open on Studocu Studocu is not sponsored or endorsed by any college or university Downloaded by Harshdeep Kaur (kharshde...
lOMoARcPSD|40737841 BIOL1131 notes - All lectures Plant and Animal Biology (University of Western Australia) Scan to open on Studocu Studocu is not sponsored or endorsed by any college or university Downloaded by Harshdeep Kaur ([email protected]) lOMoARcPSD|40737841 Daria Harris BIOL1131 Plant and Animal Biology Lecture 1 The Advent of Animals Taxonomic Classification: way of grouping biological organisms based on shared characteristics Classification of organisms is hierarchical: Kingdom, phylum, class, order, family, genus, species kids playing catch on freeways get smashed -common names often overlap with many different species The Six Kingdoms Monera v’s the rest? Ans= Prokaryote v Eukaryote Plants and some protists v’s animals, fungi and some other protists? Ans=Autotrophic vs heterotrophic Plants and animals v’s most protists and monera? Ans= Multicellular vs unicellular Animals v’s fungi? Ans= Internal vs External digestion (absorb food from environment) Plants and animals v’s the rest? Ans= Complex internal organs PLANTS VS ANIMALS Plants Animals Eukaryote Eukaryote Multicellular Multicellular Autotrophic Heterotrophic Chloroplasts No chloroplasts Cells with cellulose cell walls Cells without cell walls Mostly terrestrial Mostly marine Complex organ systems Complex organ systems Definition Animals Kingdom Animalia Multicellular – division of labour with specialised cells – in animals other than sponges, cells form tissues and organs Embryos develop from a single celled zygote Chemoheterotrophs - must ingest organic building blocks Animals first evolve in sea Downloaded by Harshdeep Kaur ([email protected]) lOMoARcPSD|40737841 Daria Harris The big breakthrough: multicellularity Three theories of animal multicellularity: 1. ciliate protist in which partitioned cytoplasm gave rise to an organism resembling a flatworm 2. flagellate protist similar to choanocytes of sponges 3. two-layered organism similar to Trichoplax 90% of animals are in these 9 phyla Phylum Example (~#species) 1. Porifera…….. sponge (8,500) 2. Cnidaria …….hydroid, jellyfish, coral (10,000) 3. Platyhelminthes……..flat worm (10,000) 4. Nematoda…….. round worm (12,000) 5. Mollusca…….. snail, mussel, octopus (100,000) 6. Annelida……. segmented worm (13,000) 7. Arthropoda……… shrimp, insect, spider (3,000,000) 8. Echinodermata…….. sea urchin, sea star (6,800) 9. Chordata……….. sea squirt, fish, frog, bird, reptile, mammal (52,000) Must know about recall the names of these nine phyla and know: a) how to recognise animals that belong to each phyla, based on their basic characteristics b) how they are linked or differentiated by their early development and body plan Trait 1: Symmetry Radially symmetrical animals often are (for some part of their life) non-motile (sessile), and live attached to a substrate Bilaterally symmetrical animals have anterior and posterior ends – Promotes cephalisation, locomotion and concentration of sensory organs Cartogram of symmetry in 9 phyla = Downloaded by Harshdeep Kaur ([email protected]) lOMoARcPSD|40737841 Daria Harris Trait 2: Germ layers and tissues Germ layers form during embryological development – Endoderm - inner layer – Mesoderm - middle layer – Ectoderm - outer layer Develop into tissues - aggregations of similar cells connected by an extracellular matrix – eg: muscle, blood, bone Germ layers Animals may have – Layers of cells but no tissues OR CAN BE – DIPLOBLASTIC (two layers of cells, with tissues, but no organs) OR CAN BE – TRIPLOBLASTIC (tissues developed from three germ layers forming complex organs) Trait 3: Cleavage patterns Triploblastic animals may be Protostomes OR Deuterostomes, OR something in between, OR a mixture of the two! Protostomes and deuterostomes develop very differently There are two types of embryonic cleavage patterns, radial and spiral Downloaded by Harshdeep Kaur ([email protected]) lOMoARcPSD|40737841 Daria Harris Trait 4: Body Cavities Acoelomate (no cavity) Pseudocoelomate (cavity between the endoderm and mesoderm) Coelomate (cavity within the mesoderm) Trait 5: Segmentation No segmentation in table ANIMALS NEED FOOD Downloaded by Harshdeep Kaur ([email protected]) lOMoARcPSD|40737841 Daria Harris Animals need energy and nutrients to function – Build/repair bodies, reproduction Includes Oxygen (O2), Carbon (C), Nitrogen (N), Water (H2O), Trace elements (e.g. P, S, Fe) Needs are highly varied – Constant ingestion to once a month How do nutrients pass into our cells? DIFFUSION Nutrients dissolved in fluid to form a solute – diffusion is the movement from high concentration to low concentration until chemical equilibrium is reached **Active transport may also occur to facilitate the movement of certain compounds against gradients to increase concentrations within certain cells Cnidarian **just two thick cells (simple system) makes diffusion easy constantly working process Diffusion becomes less effective as surface area to volume ratio decreases -> need ways to improve diffusion 1. FOLDING - increases surface area for diffusion increase surface area inside 2. SHAPE - increases SA:V ratio 3. BODY CAVITY (coelom) - increases proximity to fluid reservoir Uses for coelom: decreases diffusion distances, acts as a buffer or protective cushion for internal organs, acts as a storage reservoir for nutrients and wastes, can be used as a hydrostatic skeleton 4. TRANSPORT SYSTEMS - movement of body fluids a mechanism for reducing the diffusion distance Types of transport systems: - Digestive Organ? Gut - Respiratory Organs? lungs, gills - Circulatory Organs? heart, blood vessels - Excretory Organ? “kidneys” Digestive systems Poriferans - have no gut But, create water currents to maximise rates of feeding Downloaded by Harshdeep Kaur ([email protected]) lOMoARcPSD|40737841 Daria Harris cnidarians, platyhelminths - blind ended guts All other phyla have a complete or a ‘straight through’ gut Echinoderm -Guts differ by diet -Simple system mouth, oesophagus, stomach, intestine, rectum, anus -Stomach can be everted for external digestion Raspatory systems No perfect separation by phyla Poriferans, Cnidarians, Platyhelminths, many Annelids, Nematodes and Echinoderms exchange gasses by diffusion Molluscs, some Annelids, Arthropods & most Chordates have have gills or lungs -Gills and lungs vastly increase the respiratory surface area Circulatory systems Poriferans, cnidarians, platyhelminths, nematodes and echinoderms have no specific circulatory system - nematodes and echinoderms (coelomates) use body cavities - fine structure of hydrostatic skeleton in echinododerms works for circulating fluids OPEN: Molluscs and arthropods have a simple heart and an OPEN blood system +fluid (haemolymph) flows through sinuses and bathes the tissues. contains a blue pigment haemocyanin CLOSED: Annelids have a CLOSED blood system with pumps **most chordates have a closed system, usually with a muscular heart Excretory systems Platyhelminths have a simple system - flame cells Cilia draw coelemic fluid to end of blindended tubules Nematodes have a system of simple channels Echinoderms use their coelom and tube feet to excrete waste Annelids and molluscs have nephridiopores or coelomoducts leading from the coelom to the exterior Also occur in primitive vertebrates, e.g. hagfish Downloaded by Harshdeep Kaur ([email protected]) lOMoARcPSD|40737841 Daria Harris chordates have kidneys - modified coelomoducts Nervous systems -Sponges have no nervous system -Cnidarians have a nerve net -Triploblastic animals show cephalisation +Cephalisation = concentration of neurons at the anterior (head) end -Many Protostomes have a nerve ring around the oesophagus and ventrally directed nerve cords +Ganglions are concentrations of neuronal cell bodies – receive information from sensors Downloaded by Harshdeep Kaur ([email protected]) lOMoARcPSD|40737841 Daria Harris Lecture 2 Life in the Sea 9 main phyla + 3 muscle beds Marine in Phyla Porifera – all aquatic, mostly marine Cnidaria – all aquatic, mostly marine Platyhelminthes – most free-living forms marine Mollusca – 90% marine Annelida – 80% marine Nemertea – 90% marine species Nematoda – some marine species Arthropoda – crustaceans almost entirely marine Echinodermata – exclusively marine Chordata – two minor sub-phyla entirely marine, all craniate classes with marine forms except Amphibia Marine animals are either benthic or pelagic Benthic animals are attached to or move around near the sea floor. benthic= bottom Pelagic animals live in the water column (plankton or nekton). o Planktonic not much ability to swim the ocean and wind currents drive direction o Nekton can swim fush Costs of a marine life Lower oxygen concentration o Solution = gills to maximise surface area to have greater access to oxygen Wind, waves and currents o Solution = being sessile (organism fixed in one position, e.g. barnacle) Benefits of a marine life Stable environment Iso-osmotic conditions No desiccation no need to rely on water, surrounded by it Support for body tissues e.g. whales get big water support body Surrounded by food Downloaded by Harshdeep Kaur ([email protected]) lOMoARcPSD|40737841 Daria Harris Things exclusive to aquatic lifestyles Suspension feeding (+) o Suspension/filter feeders remove particles from the water column with the aid of filters, traps, sticky surfaces. o Deposit feeders ingest deposited particulate food o Suspension and filter feeding is a strategy that involves carnivory, omnivory and/or detritivory Broadcast spawning send eggs & sperm into water like coral Sessile existence: o Examples senssile organisms: All sponges (poriferans), Hydroids and corals (cnidarians), Oysters (molluscs), Barnacles (crustaceans), Sea lilies (echinoderms), Sea squirts (chordates) Sessile Benefits Costs – No energy required to stay still – Can’t move if conditions change – No energy required to go to catch food – May be an easy target for predators – Have an established place to live – May be prone to disturbance **it’s a trade-off where benefits outweigh the costs SPECIFIC PHYLA/ ANIMALS Porifera: the sponges Asymmetrical, Monoblastic - single layer of cells. no tissues or organs, no nervous system, hermaphrodite Morphology (study of the size, shape, and structure of animals, plants, and microorganisms and of the relationships of their constituent parts): Asymmetrical or radial Porous, many internal holes Ecological role: Suspension feeding - Filter feeding (phytoplankton and sediment from water) Chemical defence coz can’t move Phylum Cnidaria What they are: Diploblastic, two tissue layers ectoderm and endoderm, no organs, blind gut. Hydrozoa, Cubozoa, Corals (mostly colonial) (reproduce asexually) Morphology: Radial with tentacles around mouth (nematocycsts) Ecological role: Suspension feeding Defence: Cnidarians have cnidae: cells that contain nematocysts (jelly fish sting primed and always ready) main defence mechanism for a lot of cnidarias Phylum Bryozoa What they are: Diploblastic, two tissue layers ectoderm and endoderm no organs, blind gut, lophophore. Morphology: o Colonies of Zooids Passive filter with cilia (NO nematocysts) Ecological role: Suspension feeding Platyhelminthes: the flatworms (Class Turbellaria - free living flatworms) Morphology: bilateral Dorso-ventrally flattened Ecological role: Predatory Chemical defence and can take up toxins from prey to use in defence Downloaded by Harshdeep Kaur ([email protected]) lOMoARcPSD|40737841 Daria Harris Phylum Mollusca most molluscs have a shell and are: triploblastic, coelomate with mantle cavity Effective filter feeders (1L and hour) Bivalve: mussel filter feeding and attachment defence: uses Bissel threads to move things away Gastropod (example still in same phyla) can be herbivore or predator Bivalve Morphology: Calcareous shell and muscular foot Two shells No radula Ecological role: Suspension feeding Active filter feeding Habitat provision Filter water* Behavioural defence Gastopod Morphology: Calcareous shell and muscular foot Single shell Radula (all others molluscs) Ecological role: Herbivore / Predator Phylum Nematoda Downloaded by Harshdeep Kaur ([email protected]) lOMoARcPSD|40737841 Daria Harris triploblastic, coelomate, Free living and parasites Morphology: Elongate “round” worms o No segments Phylum Sipuncula: the peanut worms Morphology: bilateral No segments Retractable proboscis Filter feeders Deposit feeders, triploblastic, coelomate Annelida (Polychaeta):bristle and tube worms Triploblastic, coelomate worms parapodia, segmented, sensory structures on head Errantia and Sedentaria based on the development of the anterior appendages and life habits. 2 differnet types o Errant- active swimmer or crawler o Sedentary - burrowers or tube Morphology: parapodia, segmented,sensory structures on head Ecological role: Deposit feeders Suspension feeders Predator Phylum Arthropoda Triploblastic, chitinous exoskeleton, jointed appendages Sub-Phylum Crustacea: two pairs of antennae and mandibulate mouthparts Similar pelagic morphology Different benthic morphology Morphology: chitinous exoskeleton, jointed appendages Ecological role: Suspension feeders Herbivores Detritivores Predator Echinodermata triploblastic, coelomate, radial as adults, tube feet, rough skin “pentaradial” Morphology: radial as adults, “pentaradial” Echinoidea with “test” Ecological role: Suspension feeders Herbivores Detritivores Predator Phylum Chordata notochord, dorsal nerve chord, pharyngeal gill slits Sub-phylum Tunicata (Urochordata) Class Ascidian Morphology: thick acellular body covering Incurrent and excurrent siphon Solitary or colonial Ecological role: Suspension feeders Downloaded by Harshdeep Kaur ([email protected]) lOMoARcPSD|40737841 Daria Harris Autotrophs - photosynthetic Algae Brown Algae (Phaeophyta) Green Algae (Chlorophyta) Red Algae (Rhodophyta) Autotrophs and heterotrophs Phytoplankton floating or weakly swimming algae Autotrophic photosynthetic Zooplankton floating or weakly swimming animals heterotrophic Autotrophs - chemosynthetic Bacteria Hydrothermal vents Tube worms Methane seeps Clams 2.2: Life history, Trophic Levels and Symbiosis Food Web Downloaded by Harshdeep Kaur ([email protected]) lOMoARcPSD|40737841 Daria Harris Colonial – zooids; Siphonophores polymorphic colonial hydrozoans Colonies of four kinds of highly modified individuals (zooids). The zooids are dependent on one another for survival. float (pneumatophore), tentacles for feeding (dactylozooids) digestive (gastrozooids), reproduction (gonozooids) Symbiosis - mutualistic Coral is a symbiosis between algal cells (zooxanthellae) and the cnidarian polyp Detritivorous Symbiosis - commensal - eats pseudofeces Edotia doellojuradoi Morphology adapted to habitat Being a parasite Parasitism is a - symbiosis Parasites do not usually kill their hosts Parasites can be external or internal on plants or animals Highly adapted life cycles Highly adapted morphology Phylum Platyhelminthes Class Turbellaria - free living Class Trematoda - parasitic Class Cestoda – parasitic o Triploblastic acoelomate flat worms o Blind gut, brain and nerve cords, excretory system, complex reproductive system Downloaded by Harshdeep Kaur ([email protected]) lOMoARcPSD|40737841 Daria Harris Lecture 3 Animal Development and Reproduction MEIOSIS IN A NUTSHELL: Reduces the amount of genetic information in a cell o reduces # chromosomes by half (2n to n, diploid to haploid) Mixes up the combination of alleles by ‘recombination’ o Recombination: pieces of DNA are broken and recombined to produce new combinations of alleles. o This creates genetic diversity at the level of genes that reflects differences in the DNA sequences of different organisms The gonads are the only place in the animal body where meiosis occurs, and its function is solely to produce gametes. How is this different to Mitosis? MITOSIS the genetic material (DNA) in a cell is duplicated and divided equally between two cells. ordered series of events called the cell cycle. Cell cycle triggered by presence of certain growth factors or other signals that indicate that the production of new cells is needed. Occurs in somatic cells eg include fat cells, blood cells, skin cells, or any body cell that is not a sex cell (or a neuron!). Mitosis is necessary to replace dead cells, damaged cells, or cells that have short life spans. Organisms grow and reproduce through cell division. In eukaryotic cells, the production of new cells occurs as a result of mitosis and meiosis. Processes are similar but distinct! Gametogenesis occurs in the primary sex organs (gonads) the production of gametes by primordial germ cells and it involves both mitosis and meiosis - spermatogenesis (production of sperm) oogenesis (production of eggs) Primordial germ cells undergo a series of mitotic divisions, ‘reproducing themselves’ Give rise to multiple DIPLOID (2n) oogonia/ spermatogonia Downloaded by Harshdeep Kaur ([email protected]) lOMoARcPSD|40737841 Daria Harris Then goes through meiotic divisions, eventually halving number of chromosomes Give rise to HAPLOID (n) oocytes/ spermatocytes Germ cells (germ cells are reproductive cells) e.g of migration in chick Spermatogenesis Primary spermatocyte (product of mitotic divisions) First meiotic division - primary spermatocyte gives rise to secondary spermatocytes Second meiotic division - secondary spermatocytes give rise to spermatids Sperm maturation Spermatocyte loss of most of cytoplasm development of long flagellum (tail) formation of secretory acrosome at anterior of head section Oogenesis Primary oocyte (product of mitotic divisions – end during gestation) First meiotic division - primary oocyte gives rise to secondary oocyte AND first polar body Second meiotic division - secondary oocyte gives rise to ovum (egg) AND second polar body first polar body usually gives rise to two more polar bodies Downloaded by Harshdeep Kaur ([email protected]) lOMoARcPSD|40737841 Daria Harris Egg maturation huge increase in size increase in organelles increase in nutritive materials (mammals vs other animals?) no placenta in some animals don’t get milk once born development of protective extracellular membranes, e.g. vitelline membrane A sperm must… Find an egg Find the RIGHT egg Prevent other sperm from fertilising the right egg This is easier when fertilisation is internal External versus internal fertilisation External - outside body e.g. corals, frogs Internal - inside body – i.e. sperm must be transferred to the female reproductive tract Several options - intromittent organ (penis, claspers) OR spermatophore (sperm packet) OR injected through body wall Fertilisation – fusion of egg and sperm Downloaded by Harshdeep Kaur ([email protected]) lOMoARcPSD|40737841 Daria Harris Step 1 – Egg activation - inactive egg is activated by fusion of plasma membranes of egg and sperm – resumes synthetic activity Step 2 - Nuclear fusion - pronuclei of egg and sperm fuse - creates diploid zygote To stop more than 1 sperm entering an egg 1. Fast block: Like an electric fence (waves of changes in the electrical potential across the membrane; lasts 1-2 minutes. Based on movement of calcium ions). 2. Slow block: Elevation of the fertilization membrane: **like giant wall around egg to keep out sperm +LOOK INTO+ Sexual reproduction Diploid zygote has a full set of chromosomes, one set from each parent Recombination allows alleles to combine in new arrangements to produce a unique genotype Fertilised egg… Now the zygote must … Proliferate to make many cells Eliminate unwanted cells Differentiate the remaining cells to form different types of cells Develop tissues, organs and body structures Downloaded by Harshdeep Kaur ([email protected]) lOMoARcPSD|40737841 Daria Harris Two types of cleavage: radial and spiral = Deuterostomes & Protostomes Example of Echinoderm cleavage: Cleavage Downloaded by Harshdeep Kaur ([email protected]) lOMoARcPSD|40737841 Daria Harris Gastrulation is the rearrangement of cells in the blastula to form a gastrula BLASTULA GASTRULA No organs Germ layers (ectoderm, mesoderm, No specialised tissue endoderm) Body cavities (archenteron, coelom) Bilateral symmetry All our cells come from one of these three layers EXAMPLE Gastrulation in the sea urchin Downloaded by Harshdeep Kaur ([email protected]) lOMoARcPSD|40737841 Daria Harris EXAMPLE Gastrulation in frog Tissue development Germ Layer Ectoderm Mesoderm Endoderm Outer layer Middle layer Inner layer Body covering Gonads Lungs Neural tissue Heart and blood vessels Gut Sensory cells Nephridia (kidneys) Gut derivatives (ducts and Digestive glands tubes) Internal skeleton Muscles Determination Fate of protostome cells are determined from onset of cleavage Deuterostome cells remain totipotent until later in development (‘stem cells’) indeterminate development Downloaded by Harshdeep Kaur ([email protected]) lOMoARcPSD|40737841 Daria Harris What is a stem cell? Why self-renew AND differentiate? Embryonic stem cells Use of stem cells in research Transplantation Diagnosis Personalised medicine Use of stem cells in the clinic (patient treatment) is still controversial and experimental Protostomes vs Deuterostomes Neurulation Purpose: to form the nervous system Ectoderm (outside Mesoderm (middle) Endoderm (inside) Downloaded by Harshdeep Kaur ([email protected]) lOMoARcPSD|40737841 Daria Harris The brain starts off as a tube: The neural tube is induced by the notochord Cell development cells may either proliferate, OR undergo apoptosis (programmed cell death) surviving cells develop through a lineage of cell division by a process called differentiation in deuterostomes, undifferentiated cells that have the capacity to form many different types of cells are called stem cells embryonic stem cells (cells that make up the blastula) are able to become any cell in the fully formed body after gastrulation (process of creating three cell layers) each cell can only become a cell in the tissues that the layer will go on to produce – mesodermal cells can still form vast array of cells 3.2 Animal Life Histories Strategy a) a method of conducting operations b) skilful management in attaining an end c) the way in which something is achieved A good strategy has the lowest costs and the highest benefits of those available Animals do not “choose” between strategies Strategies evolve if they represent the best trade-off between the costs and benefits of a particular way of doing things Strategies may be constrained by an organism’s environment, and by its own characteristics Reproductive strategy a way in which genes are passed to the next generation A strategy evolved by any particular species is the one produces the maximum survival of offspring under the particular set of circumstances in which the animal exists Sexual and asexual reproduction are ‘strategies’ Asexual reproduction o one parent o offspring are genetically identical to parent and to each other Sexual reproduction o two parents with haploid gametes o offspring are genetically unique (novel combinations of genes from both parents) Downloaded by Harshdeep Kaur ([email protected]) lOMoARcPSD|40737841 Daria Harris Why bother with sexual reproduction? produces new genetic combinations provides insurance against uncertainty in the environment offspring are less well suited to the existing environment can be ‘expensive’ for parents – gamete production, predation risk, mate competition, parental care Benefits outweigh costs Sexual individuals can be… Dioecious (gonochoristic) - one sex only, either male or female Hermaphrodites – produce male and female gametes o sponges, some cnidarians, all flatworms, sea slugs, garden snails, sea squirts... even some fish and amphibians. Two types of hermaphrodites Sequential - change sex – thereby avoiding self fertilisation Simultaneous - maintain two sets of reproductive organs Examples: Sequential hermaphroditism – Animals can alternate between male and female at different stages of their life cycle Protandry: male → female – e.g. clownfish Protogyny: female → male – e.g. parrot fish Simultaneous hermaphroditism increases mating frequency useful when the chance of meeting a con-specific is low production and upkeep of TWO reproductive systems Strategies to avoid self-fertilisation anatomical separation of gonads complex courtship behaviour eggs fertility delayed until sperm are non-functional Tapeworm example - being a hermaphrodite is handy, as low chance of meeting a sexual partner in the primary host Asexual reproduction: Benefits: Don’t need a partner Can produce many offspring rapidly Maximises exploitation of favorable conditions mitotic cell division produces diploid daughter cells offspring are genetically identical to the parent (clones) Some asexual animals are colonial e.g. Bryozoans Corals Downloaded by Harshdeep Kaur ([email protected]) lOMoARcPSD|40737841 Daria Harris Hydroids Others divide asexually to produce new separate individuals Some animals have an asexual life stage **In a favourable environment, identical offspring will be well adapted Parthenogenesis Haploid gamete is produced by meiosis – nucleus fuses and becomes diploid o egg cells develop into embryos without fertilisation o offspring are usually female Obligate parthenogenesis o example: Heteronotia binoei (Binoe’s gecko), Whiptail lizard Cyclical parthenogenesis o example: some species of aphids and Daphnia Asexual reproduction: when not to do it Costs: High risk of local extinction - only successful if conditions remain favorable No genetic diversity, so can’t adapt to new or changing conditions Red queen hypothesis “sexual reproduction is maintained in populations because of the need to continually create genotypes that confer resistance against rapidly evolving pathogens and parasites” Bet hedging change reproductive strategy to get the best of both worlds ‘Strawberry-Coral Model’ (Williams 1966) Sex and dispersal is often linked Organisms often reproduce asexually ‘locally’, but dispersive phases are sexual. Reproduction in many animals has profound costs Reproductive, or ‘parental’ effort o complicated reproductive organs o provisioning of eggs o mating behaviour/competition o protection of offspring Downloaded by Harshdeep Kaur ([email protected]) lOMoARcPSD|40737841 Daria Harris o nourishment of young o parental care A ‘strategic’ trade off Reproductive costs are influenced by the mode of fertilisation External fertilisation fertilisation not assured, but a cheap option Internal fertilisation higher chance of fertilisation but energetically costly requires complex reproductive ‘equipment’ can only evolve if reproductive success increases Fertilisation mode also influences the type of development in animals External fertilisation 1. Free-living embryos, many larval stages - indirect development Internal fertilisation 1. Embryos retained initially then released - mixed development 2. Embryos retained until metamorphosis - direct development External fertilization → Indirect development Many eggs produced o small amount of yolk in each egg o embryonic development is very quick Change in form from larva to adult (metamorphosis) Planktotrophic larvae parents are broadcast spawners eggs have little yolk - cheap to make must develop rapidly must feed in the plankton very high mortality Downloaded by Harshdeep Kaur ([email protected]) lOMoARcPSD|40737841 Daria Harris wide dispersal Key advantage: separation of larval and adult niche Indirect development with planktotrophic larvae: good strategy in warm shallow tropical or warm- temperate seas Mixed development Offspring briefly protected by parent Lecithotrophic eggs – obtain some nourishment from yolk Lecithotrophic animals parents can be broadcast spawners OR internal fertilisers fewer eggs produced more costly as each egg has more yolk larvae don’t need to feed less time in the plankton reduced dispersal reduced mortality Mixed development with lecithotrophic eggs and larvae: good strategy in deep or cold water Internal fertilization → direct development Hatched or born in an adult form Direct developers parents must be internal fertilisers very few, very yolky eggs energetically costly to females embryos protected during development (sometimes ovipary, vivipary) emerge as juveniles no dispersal even lower mortality Direct development in marine animals: suits heterogeneous or unpredictable environments What are the genetic consequences of each type of animal development? Direct – recruit locally - low gene flow - genetic isolation - possibility of speciation Indirect – recruit long distances from parents - mixing of gene pools of many populations → Adaptive traits in parents may be irrelevant to offspring in new environments Consequences for population regulation? Indirect developers – events (natural or anthropogenic) in any population have little or no influence on local recruitment Direct developers - recruitment determined by circumstances within the population Profound implications for management, as populations may be regulated elsewhere Downloaded by Harshdeep Kaur ([email protected]) lOMoARcPSD|40737841 Daria Harris 4.1 Being a Vertebrate What is a chordate? GENERAL Bilateral symmetry Triploblastic Coelomate Deuterostome DEFINITIVE Pharyngeal gill slits Notochord Hollow dorsal nerve cord Post-anal tail Phyllo genetic tree (shows differences between different groups clades animals etc) Vertebrata Jaws Vertebral column Paired fins Myelinated nerves increase nerve speed travles in nerve cords Craniata Distinct head Well developed brain protected by skull Downloaded by Harshdeep Kaur ([email protected]) lOMoARcPSD|40737841 Daria Harris Agnatha – Jawless fishes +Agnatha (jawless) / not mouthless! cyclostomes (circle mouth); Jawless doesn’t mean mouthless jaw supports the mouth and allows chewing Class Myxini (hagfishes) – Craniate – No vertebrae Class Petromyzontida (lampreys craniate image) – Craniate – Precursors of vertebrae (therefore nearly a vertebrate?!) now grey out last section and move onto proper vertebrates Vertebrate form & function Same basic body plan but variation os constant theme Some variation in function of body parts both in form and function For example Gnathostomes – Jawed fishes Class Chondrichthyes – Cartilaginous fish – No true bone (lost) – Teeth (denticles) not fused to jaw (actually associated with skin) Class Actinopterygii – Ray-finned fish – Bony skeleton – “True” teeth Downloaded by Harshdeep Kaur ([email protected]) lOMoARcPSD|40737841 Daria Harris Sacropterygii – fleshy finned fishes Coelocanths & Lungfishes Lobe (fleshy)-finned fish Mostly fossils but ‘living fossil’ species, discovered by Miss Marjorie Latimer Lungfish closely linked to tetrapods -in dotted line- (4 limbs) – Pelvic girdle – Skull bones – Heart structure – Embryology – DNA Tetrapods Four limbs with separate digits Downloaded by Harshdeep Kaur ([email protected]) lOMoARcPSD|40737841 Daria Harris Why aren’t the mammals at the top right of the tree? Think of phylogenies as flexible, like a hanging mobile The arms can pivot, but the relationships between groups is fixed Amphibia Includes; Frogs and toads Salamanders, mud puppies & newts Caecilians Most require water to reproduce Indirect development (tadpole larva) Most larvae fully aquatic with gills All adults show some reliance on skin for oxygen input and waste removal (permeable skin) Amphibians in Australia ~250 species of frogs in Australia, ~80 in WA Live in all environments No caecilians, salamanders, newts or toads (until cane toads...) ** in two classes orange and blue Amniotes Sauropsids and mammals are amniotes 4 Extra-embryonic membranes; 3 new – Yolk sac (yolk energy store ) – Chorion (under egg shell) – Amnion (around embryo) – Allantois (waste sac) Direct development Water-resistant skin Downloaded by Harshdeep Kaur ([email protected]) lOMoARcPSD|40737841 Daria Harris Suited for terrestriality Amniote skulls Turtle is oldest skull thought as only eye sockets Sauropsids Sauropsids: turtles, crocodiles, birds, snakes, lizards & geckoes: but not as we’d expect... Traditional: Revised: just don’t know where turtles branched off – where they belong Mammals Relatively few species – but large biomass Characterised by hair & mammary glands Evolved from mammal-like reptiles – therapsids Two sub-classes show progression in reproductive traits Protheria (Monotremes): lay eggs Theria: bear live young, embryos nourished from wall of uterus (through placenta) Downloaded by Harshdeep Kaur ([email protected]) lOMoARcPSD|40737841 Daria Harris – Metatheria (Marsupials): yolk-sac ‘placenta’, young born less developed early ‘fetal’ stage – Eutheria (Placentals): true chorio-allantoic placenta, young born more developed stage Mammary glands A key mammal character Nutrition, antibodies Endothermy (capacity to regulate body temp) A key mammal character What does it mean? Mammals are endothermic (so are birds) – they precisely regulate their body temperature using metabolic heat production – other vertebrates are ectotherms Were dinosaurs endothermic? Lots of debate many dinos had feathes Thermoregulation versus thermoconforming A thermoconforming organism, by contrast, simply adopts the surrounding temperature as its own body temperature, thus avoiding the need for internal thermoregulation. Why are vertebrates important? Diversity of form: Vertebrates show all feeding strategies: Suspension feeders Deposit feeders Detritrivores Grazers Gatherers Predators Parasites Vertebrates provide ecosystem services Food source Pollination Seed dispersal Ecosystem engineers Apex predators – Top down control Ecosystem engineers Facilitate other species through their presence or activities Lecture 4.2 Metabolism, Energetics and Movement Cell metabolism is the use of energy & catabolism/anabolism of organic molecules Animal metabolism is the overall direction of biochemical reactions; it defines animal ‘life’; it produces heat ~The fire of life ~ BUT still must obey laws of physics Downloaded by Harshdeep Kaur ([email protected]) lOMoARcPSD|40737841 Daria Harris Physical laws dictate energy exchange & transformation in all reactions (including biological ones), so important for biologists to understand the physical laws of energy & heat exchange Metabolic rate of animals depends on a number of factors, including presence of: – oxygen – activity level – body mass – cellular complexity, phylogeny – body/ambient temperature ATP & energy Adenosine triphosphate (ATP) ATP is a high-energy molecule used by cells as energy source by losing phosphate groups ATP → 22 kJ useful energy / mol Also used for DNA & RNA coenzyme Anaerobic (Glycolytic) Metabolism Glucose → 2 lactate + 2 ATP + 45 kJ/mole useful energy + 72 kJ/mole heat energy = 117 kJ/mole Aerobic (O2 ) Metabolism Glucose + 6 O2 → 6 CO2 + 6 H2O + 32 ATP + 1161 kJ/mole useful energy + 1693 kJ/mole heat energy = 2870 kJ/mole ITS BETTER Where did O2 come from? Atmosphere contained no O2 at first First photosynthetic bacteria were anaerobic - metabolism did not use O2 ; trapped sunlight energy used to synthesise organic molecules from CO2 From 2 BYBP plants produced O2 as a by-product, accumulated to current level of 21% atmosphere Animal metabolism Animal metabolism is sum total of all biochemical reactions occurring in the body of an animal – Or subsets thereof, eg for glucose metabolism If the metabolic rate of an animal is a measure of its overall cellular metabolism, then how is it measured? Metabolic rate measurement Glucose + 6 O2 → 6 CO2 + 6 H2O + 2870 kJ/mole Downloaded by Harshdeep Kaur ([email protected]) lOMoARcPSD|40737841 Daria Harris There are a variety of possible ways to measure metabolic rate: 1. heat production 2. oxygen consumption 3. carbon dioxide production 4. energy balance (IN = OUT) 5. substrate utilisation 6. metabolic water production Heat production Best measure of metabolic rate? Antoine Lavoisier (1743-1794) Named oxygen and hydrogen Helped create metric system, first law thermodynamics Measured direct heat production using a simple but ingenious method 2. Oxygen consumption 3. Carbon dioxide production 4. IN-OUT energy balance It is possible to determine the metabolic rate of an animal by its overall energy balance Food Drink Faeces Urine Growth Reproduction Unaccounted energy = metabolic energy Determinants of metabolic rate 1. Activity Level 2. Body Mass 3. Taxonomy 4. Temperature Activity Level Metabolic rate varies from Minimal / basal level to Downloaded by Harshdeep Kaur ([email protected]) lOMoARcPSD|40737841 Daria Harris Maximal / summit level Body Mass Relationship between body mass & metabolic rate Does a bigger animal have a higher or lower metabolic rate than a smaller animal? Some books suggest smaller animals have a lower metabolic rate Relationship is a curve The curve can be converted to a straight line using a logarithmic scale for both axes The slope of the log-log relationship is 0.75 Why? Unknown mystery! Possible reasons include 0.75 is a 'compromise’ 1.0 = metabolism proportional to mass 0.67 = metabolism proportional to surface area Theoretical slope in 4-dimensional space (length, breadth, depth, time) is 0.75 the slope is 0.67 in three dimensional space (length, breadth, depth). Fractal scaling, or patterns of shape. etc…… Unfortunately, none of these is convincing! Don’t get confused metabolic rate and mass mass is per gram of mass that’s why smaller animals can look like they have higher rate Many physiological processes proportional to metabolic rate, not body mass ‘Metabolic mass’ is the physiologically-related “mass” It changes less rapidly than actual body mass Downloaded by Harshdeep Kaur ([email protected]) lOMoARcPSD|40737841 Daria Harris Cellular Grade Same effect of mass on metabolism for almost all animals slope = 0.75 However, metabolic rate varies dramatically for three groups of animals: Temperature Chemical reactions are (exponentially) faster at higher temperatures Q10 = proportional increase in reaction rate (K) over 10°C range For physical reactions, like diffusion, Q(under)10 ≈ 1.1 For biochemical reactions & biological processes, Q10 ≈ 2 - 3. Activity Locomotion has a major metabolic cost Aquatic = swimming Terrestrial = running Aerial = flying Locomotion Animal locomotion Aquatic (swimming) Terrestrial (slithering, walking) Aerial (gliding and flying) Downloaded by Harshdeep Kaur ([email protected]) lOMoARcPSD|40737841 Daria Harris Terrestrial locomotion is based on lever mechanics (usually) Aquatic & Aerial locomotion have similar fluid-dynamics (surprisingly?) similar concept swimming (water) is based on drag flying (air) is based on lift Animal locomotion – swimming Animals can swim by a variety of means, including Jet propulsion – squeezing water out of a chambre Drag from limbs, body or fin undulations ‘Flying' using lift Jet propulsion Jellyfish (Cnidaria) Circular muscles around bell Cephalopods (Mollusca) Octopus, squid, cuttlefish Circular muscles around mantle, siphon for direction Bivalves (Mollusca) Scallops Adductor muscles pull shells together, forces water from the shells Drag from Limbs, Body, Fins Drag in water counteracted by thrust Like a rowing oar, limbs, fins & bodies push back on water; drag provides forward force Flying underwater Less common Uses hydrofoil (≈ ‘wing’) to create lift for propulsion ‘Flying’ only works for relatively big aquatic animals Aerial - gliding and flying Parachuting A 'primitive' form of flight Drag slows fall of object Parachuting similar 'rowing‘ (drag) Can be turned into gliding (+ lift) Various animals glide (SE Asia!): Frogs, Snakes, Lizards, Birds, also plant seeds Downloaded by Harshdeep Kaur ([email protected]) lOMoARcPSD|40737841 Daria Harris Gliding uses part of the lift force to overcome drag So the animal maintains speed But insufficient lift to balance weight So animal moves forward horizontally & descends Gliding uses less energy than powered flight Birbs glide ass well… still capable of powered flight though Called soaring instead of gliding Powered flight Flying animals provide extra energy through their wing movements (muscle contraction) to maintain their horizontal position (lift = body weight) and forward velocity Evolution of Powered Flight – 2 ways Terrestrial - slithering, walking, hopping Running / walking Limbs (‘levers’) support / lift body off ground & provide forward movement Lift pattern can confer stability: centre of mass held in tripod formed by three limbs contacting ground Metabolic cost of walking/running is typically linear with speed (especially by appropriate gait selection) The net cost of transport (slope of line) is independent of speed. Hopping Hopping animals, eg kangaroos, have wide range of speeds with no effect on the metabolic cost Tendons act like springs; store elastic energy for re-bound Hopping at high speed is very economical Downloaded by Harshdeep Kaur ([email protected]) lOMoARcPSD|40737841 Daria Harris Animal locomotion – metabolic cost Transport costs vary with body mass, speed & locomotion type More economical per gram for large animals Most economical for swimmers, least economical for runners Lecture 5.1 Gas Exchange Gas Exchange “Respiration” Gas exchange / respiration / external respiration is the supply of oxygen to, and removal of carbon dioxide from all cells of the body Gas exchange occurs over living cells – so wet surfaces +In aquatic habitats, O2 dissolved in water, so no issue with water +In terrestrial habitats, O2 in air, air with less than 100% RH results in loss of water by evaporation during respiration… So respiratory gas exchange often requires mechanisms to limit water vapour loss. Gases in Dry Air At sea level 1 atmosphere = 101.3 kiloPascals = 1013 hectaPascals also = 760 mm Hg = 760 torr Downloaded by Harshdeep Kaur ([email protected]) lOMoARcPSD|40737841 Daria Harris Partial Pressures of Gases Partial pressure = fraction of total atmospheric pressure pO2 = 101.3 kPa (total atmospheric pressure) x 21/100 (percentage of O) = 21 kPa Partial pressure is the physiologically relevant measure not %, concentration, mole fraction or other… Total atmospheric pressure at top of Mt Everest = 33.6 kPa pO2 = 33.6 kPa x 21/100 = 7 kPa = unconsciousness! Principles of Gas Exchange: 1. Diffusion (PASSIVE) Diffusion is the exchange of gases (O2 , CO2 , N2 ) by the random thermal* motion of molecules in air (or water) * Temperature dependent Passive physical process NOT active transport The movement of O2 from lungs into the blood is example of diffusion Rate of exchange = ∆ Quantity / ∆ time (where ∆ = change) Diffusion depends on: partial pressure difference (∆ pO2) area (A) for exchange distance (L) over which diffusion occurs Fick's Law of Diffusion Adolf Fick, 1855 Relates three important variables with a physical constant Rate of exchange = ∆Q / ∆ t = D. A. ∆ pO2 / L where D is the diffusion coefficient Downloaded by Harshdeep Kaur ([email protected]) lOMoARcPSD|40737841 Daria Harris physical constant for air or water *****DON’T NEED TO REMEMBER EQUATIONS) 2. Convection (ACTIVE) Convection is the exchange of gases / fluids (O2 , CO2 , N2 ) by movement Most animals have a convection mechanism to move water or air over their respiratory surface Will minimise depletion of O2 and build up of CO2 near gas exchange surface This movement is called respiratory ventilation Fick’s Principle Adolf Fick, 1870 Relates fluid flow (F; ml/min) variable with a physical constant Rate of exchange = ∆Q / ∆ t = a. ∆ pO2. F where a is the solubility coefficient physical constant for air or water Oxygen cascade – diffusion O2 delivery from external environment (air or water) to mitochondria inside cells follows the ‘O2 cascade’ Two diffusion steps in the O2 cascade are: 1. Across respiratory surface 2. Diffusion from blood to cell / mitochondria Oxygen cascade – convection Two convection steps in the O2 cascade are: 1. Respiratory ventilation 2. Blood circulation Allometry (how dies size effect surface area and v) Increasing size is complicated. Consider body length: Body length related to o Strength & speed – limb & muscle length But surface area related to body length squared o SA = gas & nutrient exchange And volume and weight related to body length cubed o Vol = body mass of cells to maintain Downloaded by Harshdeep Kaur ([email protected]) lOMoARcPSD|40737841 Daria Harris SA/Vol is important ratio = SA per unit mass Relying on diffusion for gases & nutrients quickly becomes impossible Diffusion is fine when small but not when big Body Surface as Respiratory Structure Small animals, flat animals few cells / less than 1 mm thick Can rely on gas exchange by diffusion across body surface Sponges can be large (over 1 m), but rely on diffusion Entire body always a few cells thick +++ Many aquatic phyla and even a few terrestrial animals (eg, small, moistskinned amphibians - salamanders) can rely on gas exchange across their skin Cutaneous gas exchange is limited for large animals by low surface-to-volume ratio gas-impermeable surfaces (shells, skin) Diffusion across respiratory surfaces All examples are diffusion, regardless of type of animal Downloaded by Harshdeep Kaur ([email protected]) lOMoARcPSD|40737841 Daria Harris Surface Respiratory Structures in Animals Air-breathing insects / others Internal TRACHEA Rigid internal branching tubes Along which O2 and CO2 diffuse Aquatic animals Evaginated GILL Often protected by a rigid covering Water keeps gill surfaces separated Air-breathing animals Invaginated LUNG Air pressure wet lung surfaces apart Opposing surface tension Surface Respiratory Structures – insects etc Most successful air-breathing invertebrates = insects, spiders & scorpions Tracheal gas exchange system Spiracles = openings Internal tubes = trachea – rigid, branching tubes to all cells Diffusive gas exchange Tracheal respiratory system works well for small insects, but not big insects Largest extant insects? Downloaded by Harshdeep Kaur ([email protected]) lOMoARcPSD|40737841 Daria Harris Goliath beetles, ca. 11 cm Some abdominal pumping (convection) to large air sac, but trachea all diffusion Largest insect ever? Giant Permian dragonflies, 70 cm air was 29% O2 Surface Respiratory Structures – Gills Many aquatic invertebrates have complex gills External water flow across gill filaments is in opposite direction to internal blood flow counter-current exchange Crustaceans Note the carapace = rigid covering protection for gills Bony fishes have complex gills Water is drawn into mouth passes through gill arches exits via operculum* *rigid covering protection for gills Gill arches have numerous plate-like extensions Gill filaments Gill filaments are covered by small gill lamellae Lamellae = site of gas exchange between water and blood External water flow across lamellae in opposite direction to internal blood flow counter-current exchange Counter-current exchange Counter-current exchange is an important concept in physiology Maximises exchange Theoretically to nearly 100% Usually less in biological systems Water and blood flow in opposite directions to maximise exchange Gases (O2 & CO2 ) Salts Other metabolites Heat (to maintain internal body temperature) Concurrent exchange (THE OPPOSITE) concurrent / co-current flow Downloaded by Harshdeep Kaur ([email protected]) lOMoARcPSD|40737841 Daria Harris Water and blood flow in the same direction Exchange leads to equalised levels Counter-current exchange is more efficient Surface Respiratory Structures – Lungs Some air-breathing invertebrate animals, have a simple diffusion lung EG pulmonate snails & slugs Air-breathing Fishes Early fishes evolved paired lungs for air-breathing gulp air using buccal (mouth) pumping Two ways to Breathe = ventilation Some Fishes have converted the lung to a swim bladder, for buoyancy regulation Some bony fishes have modified the swim-bladder back to a lung other air breathing A few fishes can also breathe air, using other structures, including modified gills, their skin or even their gut The Oriental Weatherloach swallows air, exchanges gas in hind gut, in addition to its gills other ‘gut’ breathing Sea cucumbers are unusual in lots of ways… Respiratory tree (aquatic ‘lung’) off rectum Water is sucked into & expelled – convention! Surface Respiratory Structures – Amphibians Two stroke ‘buccal pump’ Throat muscles suck air into buccal cavity = inspiration I. − Nostrils open, glottis closed Throat muscles pump air into lungs = inspiration II. − Nostrils closed, glottis open Rely on water pressure to push air out of lungs = expiration Downloaded by Harshdeep Kaur ([email protected]) lOMoARcPSD|40737841 Daria Harris Four stroke ‘buccal pump’ 1. Buccal pump to ventilate buccal cavity 2. Buccal pump to fill buccal cavity 3. Elastic recoil of lungs to expire lung air into buccal cavity; mix lung with fresh air 4. Buccal pump to push mixed air into lungs 5. Buccal pump to empty the buccal cavity Surface Respiratory Structures – Reptiles Reptiles have more advanced respiratory system than amphibians The lung is sometimes a simple sac But usually extensive folding of lung surface = high surface area Typically, rib-cage muscles expand and collapse lungs A few species (e.g. goannas) can also use the buccal pump to force air into lungs Crocodiles have more complicated system for lung ventilation They have a rib cage and… Lungs are connected to liver by the diaphragmaticus muscles, which contract and expand lungs by pulling on the liver Surface Respiratory Structures – Mammals Mammals have a very complex lung Lung surface is divided into numerous small sacs, called alveoli Alveoli increase enormously the surface area for gas exchange (50- 75 m2 ) – same as a tennis court But breathing is still ‘tidal’ Air entering & exiting lungs mixed Surface Respiratory Structures – birds Birds have the most complicated lung structure of all vertebrates The lung is rigid & connected to numerous, extensive air sacs Provides unidirectional / one-way airflow through lung tissue Air entering does not mix with air exiting lung Inspiration expands air sacs, sucks Downloaded by Harshdeep Kaur ([email protected]) lOMoARcPSD|40737841 Daria Harris fresh air into posterior air sacs & old air from lungs into anterior air sacs Expiration collapses air sacs, pushes fresh air from posterior air sacs to lungs, & old air from anterior air sacs outside Blood flow is more or less perpendicular to air flow = cross-current flow Although not counter-current flow, bird lung is more efficient than mammal / other vertebrate lungs Thus is birds can breathe at much higher altitudes than mammals Mount Everest = 8848 m One third of oxygen at sea level Humans lose consciousness in minutes Kerosene cannot burn Most helicopters cannot fly there Bar-headed geese can fly over Mt Everest The highest-flying bird ever recorded was a Ruppell's griffon vulture (wingspan 3 m) sucked into jet engine 12,200 m above the Ivory Coast (29 November 1975) 4 km higher than Mount Everest! Changes with altitude Downloaded by Harshdeep Kaur ([email protected]) lOMoARcPSD|40737841 Daria Harris Mammals & the ‘bends’ With depth comes pressure Gases dissolve into body fluids When surfacing, pressure is reduced dissolved gases bubble out of solution bubbles in small blood vessels causes pain = the bends Whales avoid this by collapsing their lungs when they dive no gas exchange from lungs Lecture 5.2 Water, Solutes and their Circulation Water and Solutes Water Solvent for life on earth Essential constituent of plant and animal cells. Solutes Salts (e.g. NaCl) Organic components (e.g. sugars, fatty acids, amino acids) Waste products (e.g. CO2 , ammonia, urea) Body water content and solute levels must be maintained within a tolerable range for normal cellular functions. Solutions =solvent plus solute Biological solutions are water containing salts, sugars, fatty acids, amino acids etc Water molecules in a solution have lower energy level (i.e. water potential, ) than water molecules alone (i.e. pure water) Water is a small molecule (H2O) So moves freely across cell membranes from high to low water potential (i.e. from solutions with high dissoved solutes to those with low dissolved solutes) Solution concentration Solute concentration (C): number of moles of a solute per litre of solution e.g. 1 mole = 58.5 g of NaCl to a final volume of 1000 ml is a one molar NaCl solution Osmotic concentration (P): total number of moles of all solutes per litre of solution e.g. 1 mole = 58.5 g of NaCl to a final volume of 1000 ml is a two osmolar solution (because a mole of NaCl dissociates into a mole of Na+ and a mole of Cl-) Downloaded by Harshdeep Kaur ([email protected]) lOMoARcPSD|40737841 Daria Harris A solution with high solute concentration has low water potential Osmosis Osmosis is ‘diffusion’ of water Water molecules will move from a dilute solution to a concentrated solution by osmosis - But only if the solute is impermeable. e.g. water will move across a sugar-impermeable membrane separating dilute and concentrated sugar solutions At equilibrium, osmotic force = hydrostatic force = pressure loading A 1 osmolar solution has an equivalent water potential to a water column about 224 m high h = 224 m for freshwater-to-seawater! The osmotic effect occurs if solute is NOT permeable across the membrane separating the solutions (e.g. haemoglobin in RBCs) +Cells will expand or contract if there is an osmotic imbalance to allow water movement out of or into a cell Environments Unicelluar protozoans experience two environments Multicellular animals there are three environments Intracellular environment & external environments -Animals evolved in seawater -Their intracellular environments often have similar Osmotic Concentration OC as seawater BUT has different concentration of ions and organic solutes Downloaded by Harshdeep Kaur ([email protected]) lOMoARcPSD|40737841 Daria Harris Extracellular environment & external environments The extracellular environment is similar to seawater for some marine animals (crabs, hagfish) BUT can very different to seawater for other marine animals (e.g. bony fish) The Osmotic Concentration OC (osmotic pressure) is always the same for both extracellular and intracellular environments of multicellular animals even though their solute compositions are very different this is NOT true for plant cells and microorganisms Conform or regulate? Osmo-conforming Solute composition of extracellular fluid is same as ambient medium, even if it changes (e.g. ghost shrimp) Osmo-regulating Solute composition of extracellular fluid is different from ambient medium, especially if it changes (e.g. brine shrimp) osmo- regulating is energetically expensive (ion pumps) Iono-conforming (especially marine species): Ionic composition of extracellular fluid is virtually the same as the ambient medium, even if the composition of the medium changes But note high ion concentrations interfere with proteins Iono-regulating (especially freshwater species): Ionic composition of extracellular fluid is di dfferent from the ambient medium, especially if the composition of the medium changes There are THREE combinations of osmoconform/regulate and ionoconform/regulate among aquatic animals: Downloaded by Harshdeep Kaur ([email protected]) lOMoARcPSD|40737841 Daria Harris Osmo-conform/Iono-conform Most marine invertebrates osmo-conform and iono-conform to seawater e.g As do hagfishes, the most basal / ‘primitive’ vertebrates They essentially do not gain or lose water or ions - they are in equilibrium. Osmo-conform/Iono-regulate Sharks & relatives, coelacanths, and one species of frog osmo-conform to seawater, but iono-regulate (at lower concentrations) Urea (plus Trimethylamine N-oxide TMAO, other solutes) fill the 'osmotic gap’ Ureo-osmo-conforming animals are : in water balance with seawater gain ions, lose urea by diffusion Why? Salt impacts cell function Osmo-regulate / Iono-regulate – seawater Many vertebrates osmo-regulate and iono-regulate in seawater lampreys and bony fishes Passive processes Lose water by osmosis through gills Gain ions by diffusion Active regulation Gain water (drinking) Lose ions (ion pumps in gills, kidneys Osmo-regulate / Iono-regulate – freshwater Animals must osmo-regulate and iono-regulate in freshwater no animal can survive with body fluids as dilute as freshwater Passive processes Gain water by osmosis through gills, Lose ions by diffusion Active regulation Lose excess water as copious urine Gain ions by active ion uptake in gills, kidneys Hyper osmo-regulate / Iono-regulate In hypersaline (salty) environments No animal can survive with very concentrated body fluids Animals must osmo-regulate and iono-regulate (remove salts) e.g. brine shrimp have salt glands on legs, in gut Terrestrial Animals Downloaded by Harshdeep Kaur ([email protected]) lOMoARcPSD|40737841 Daria Harris All terrestrial animals osmo-regulate and iono-regulate Must, due to fluctuations in available water and solutes Water gain Drinking Water in food Metabolic water (aerobic respiration) Water loss Evaporation (lungs and skin), Urine Faeces Solutes are not exchanged across body surface Water Budget: In and Out e.g. The thorny devil is an interesting Australian lizard Arid zone specialist Ant-eater ‘Blotting paper’ skin absorbs water, then transfers it by capillarity to the mouth for drinking Water is not absorbed across skin Circulatory Systems Water and solutes enter body in one location eg mouth (drinking), gills (diffusion) etc Must be transported from entry location to all organs in the animal Diffusion transport water and solutes slowly and over short distances (a mm or so) Convection required to transport of water and solutes rapidly over longer distances Bulk flow (convection) in animals is usually accomplished by a circuit-loop (unidirectional, not tidal) circulatory system NO Circulatory Systems? NO circulatory systems – similar for no respiratory systems Small animals, flat animals few cells / less than 1 mm thick Can rely on gas exchange by diffusion across body surface Downloaded by Harshdeep Kaur ([email protected]) lOMoARcPSD|40737841 Daria Harris Circulatory Systems In larger animals, the circulatory system has many roles: Transport – Gas (O2 and CO2 ) – Nutrient and waste – Hormones, etc – Immune cells and components – Heat Blood pressure – Hydrostatic skeleton (incl. erectile tissues) – Locomotion (spider legs) Clotting components Heart(s): pumps blood through the blood vessels Blood Vessels: conduits for blood flow arteries = away from heart, to organs through capillaries (if closed system), to veins = towards heart Circulatory Systems – closed systems Closed circulatory systems have a complete vessel network Vessels from the heart, capillaries into organs, back to the heart Blood cells remain within vessels / circulatory system eg Annelid worms have a closed circulatory system Circulatory Systems – open systems Open circulatory systems have an incomplete vessel network Vessels from the heart, NO capillaries, vessels back to the heart Blood cells leave vessels, blood ‘percolates’ through organ/tissue spaces, returns to vessels to enter heart Arthropods have an open circulatory system Vertebrate Circulatory Systems The most basal vertebrates (e.g. hagfishes) have a slightly open circulatory system All other vertebrates have a closed circulatory system Increasing efficiency: Fishes have single pump, single circuit Tetrapods have single pump, double circuits Mammals & birds have double pump, double circuits Fish Circulatory Systems Fishes have simple circulation: single pump, single circuit Heart pumps blood to gills, blood is oxygenated Then blood flows to body to deliver oxygen to the organs Downloaded by Harshdeep Kaur ([email protected]) lOMoARcPSD|40737841 Daria Harris The heart is 2-chambered thin-walled atrium receives blood thick-walled ventricle pumps blood to gills, then to body - most of the pulsatile blood pressure Amphibian Circulatory Systems Frogs etc have more complex circulation; single pump, double circuit system Heart pumps blood to lungs (larvae = gills) and skin, blood is oxygenated & returns to heart – first circuit Heart pumps blood to body to deliver oxygen to the organs – second circuit Heart has three chambers: Right atrium → ventricle to lungs/skin Left atrium → ventricle to body Ventricle structure & spiral valve keep oxygenated & deoxygenated blood partly separated Reptile Circulatory Systems Reptiles (NOT crocodiles) simpler than amphibians; single pump, double circuit system Heart pumps blood to lungs (only), blood is oxygenated & returns to heart – first circuit Heart pumps blood to body to deliver oxygen to the organs – second circuit Heart has three chambers: Right atrium → ventricle to lungs Left atrium → ventricle to body Ventricle has three chambers, keeps oxygenated & deoxygenated blood well separated (but evolutionary dead end) (Crocodiles) more complex; double pump, double circuit system Heart pumps blood to lungs, blood is oxygenated & returns to heart – first circuit Heart pumps blood to body to deliver oxygen to the organs – second circuit Heart has four chambers: Right atrium → Right ventricle to lungs Left atrium → Left ventricle to body Oxygenated & deoxygenated blood well separated Retain extra-cardiac connection between R & L ventricles (foramen of Panizza) to bypass lungs when diving Mammal & bird Circulatory Systems Mammal & bird most complex; double pump, double circuit system Heart pumps blood to lungs, blood is oxygenated & returns to heart – first circuit Heart pumps blood to body to deliver oxygen to the organs – second circuit Heart has four chambers: Downloaded by Harshdeep Kaur ([email protected]) lOMoARcPSD|40737841 Daria Harris Right atrium → Right ventricle to lungs Left atrium → Left ventricle to body Oxygenated & deoxygenated blood well separated Unable to bypass lungs (except embryonic circulatory system) Birds opposite to mammals Blood Pressure The heart(s) generates blood pressure to push blood through the vessels High pressure in arteries Low pressure in veins Due to high resistance in capillaries Double pump = higher blood pressure (as well as separation of oxy/deoxy blood) Also important for large animals… Blood Pressure & gravity Arterial blood pressure pushes blood against gravity to brain Small animals: trivial Humans need 50 mm Hg blood pressure (of 100 mm) Giraffes need 200 mm Hg Sauropod dinosaurs would need >500 mm Hg Lecture 6.1 Digestion Downloaded by Harshdeep Kaur ([email protected]) lOMoARcPSD|40737841 Daria Harris Organic matter & energy Animals require other organic matter & energy to survive Energy can be acquired by animals in three different ways: Chemotrophs Autotrophs Heterotrophs Chemotrophs Real chemotrophs are bacteria synthesise organic materials from simple chemicals (H2 , H2S, NH3 or ferrous iron) Various invertebrate animals (e.g. annelid worms, bivalve molluscs, decapod crabs) form symbiotic relationship with bacteria Animal host gains some/all of their energy & nutrients from symbiont; often through simple compounds (e.g. acetic acid) Pogonophorans (‘giant tube worms’, a kind of annelid worm) at deep sea-vents; lack feeding anatomy, gut, adapted for hosting bacteria (Photo)Autotrophs Real autotrophs are cyanobacteria, singlecelled ‘algae’ protists, algae etc synthesise organic materials from photosynthesis Various invertebrate animals (e.g. corals, bivalve molluscs) form symbiotic relationship with photoautotrophs Animal host gains some/all of their energy & nutrients from symbiont; often through simple compounds (e.g. acetic acid) Giant clams (a kind of bivalve) in shallow waters; also feed using filter feeding Heterotrophs All animals are heterotrophs Gain their energy by consuming organic material Herbivores – plant material Carnivores – animal material – Insectivores are carnivores specialised on insects Omnivores – plant & animal material Other specialisations Saprozoic animals –obtain organic chemicals by absorption across the body surface e.g. this gutless worm Carnivorous plants A few plants are also carnivorous They digest invertebrates (usually insects) to gain nitrogen and minerals Downloaded by Harshdeep Kaur ([email protected]) lOMoARcPSD|40737841 Daria Harris They typically live in nutrientdeficient freshwater wetlands; bogs and marshes Animal body composition Water Water is essential for life Liquid water is ‘universal solvent” for life A few organisms survive outside of standard physico-chemical state of water Cryptobiosis = ‘hidden life’ Anhydrobiosis = absence of water Osmobiosis = high solute concentration Cryobiosis = frozen water Anoxybiosis = lack of oxygen All specialised, highly-adapted to their extreme habitats Organic matter Organic matter provides nutrients in four macromolecules Carbohydrates Lipids Proteins Nucleic acids Vitamins Downloaded by Harshdeep Kaur ([email protected]) lOMoARcPSD|40737841 Daria Harris Vitamins are organic compounds Essential for physiological function Not made by animal (so vary) Needed in trace amounts The first vitamin discovered was thiamine (B1), the deficiency of which causes beri-beri (nervous system) Human unable to synthesise Vitamin C deficiency causes scurvy Other mammals (e.g. rats) do synthesise Some animals obtain all of their vitamins from symbiotic microorganisms Minerals Many minerals are required Major minerals: Downloaded by Harshdeep Kaur ([email protected]) lOMoARcPSD|40737841 Daria Harris calcium and phosphorus (bone), potassium, sodium and chlorine (body fluid ions) & magnesium (enzymes) Minor minerals (aka trace elements; 50 kg) use large intestine Gut morphology & absorption Carnivore Herbivore Pre-peptic fermenter Downloaded by Harshdeep Kaur ([email protected]) lOMoARcPSD|40737841 Daria Harris Lecture 6.2 Temperature, Thermoregulation and Stressful Environments Temperature Temperature is one of the most important abiotic factors for animals Temperature effects state of water In general, increasing temperature increases chemical reaction rates But biological chemical reactions have narrow range, typically 0 to 50°C Biophysics of Temperature Temperature is the average kinetic (movement) of a molecule Heat is the total kinetic energy content of all molecules Temperature ≠ Heat consider steel vs plastic at 60°C Q10 Quantifying temperature effect Chemical reactions occur exponentially faster at higher temperatures Q10 is the proportional increase in reaction rate (K) over a 10°C change in temperature i.e. Q10 = K(t+10) / K(t) Q10 for physical reactions (diffusion) about 1.1 Q10 for biological reactions (with enzymes) is between 2 and 3 Biochemical & biological reactions, mediated by enzymes, have lower and upper temperature limits Upper limit due to enzymes lower limit die to water Temperature Biological chemical reactions have narrow range, typically 0 to 50°C Thermophiles 30 to 50°C Mesophiles 10 to 30°C Downloaded by Harshdeep Kaur ([email protected]) lOMoARcPSD|40737841 Daria Harris Psychrophiles 0 to 10° C Some organisms (bacteria, basal plants, animals) tolerate up to 100° C Few bacteria can tolerate over 100° C if ambient pressure is high enough to prevent boiling! Some organisms tolerate 0°C (freshwater) or -1.86°C (seawater) by either avoiding freezing, or tolerating freezing of their extracellular fluids. Few organisms can tolerate freezing at extremely low temperatures Temperature & proteins Thermophiles at high temperatures keep protein structure stable with less hydrogen (weak) bonds more hydrophobic (strong) bonds Increases rigidity of proteins Psychrophiles at low temperatures keep protein structure stable with more hydrogen (weak) bonds less hydrophobic (strong) bonds Increases functional “flexibility” of proteins Heat Exchange Mechanisms for heat exchange Conduction Convection Radiation Evaporation (Condensation) Metabolic Heat Production (MHP) Body Temperature Terminology Downloaded by Harshdeep Kaur ([email protected]) lOMoARcPSD|40737841 Daria Harris Temperature regulation is an important concept In general, ectotherms are thermally passive; they thermoconform to the environmental temperature However, some ectotherms, are able to achieve a particular ‘preferred’ body temperature; they use thermal variation within the environment (e.g. solar radiation) to thermoregulate In general, endotherms are thermally active; they use physiology (e.g. metabolic heat) to thermoregulate and thus maintain a constant temperature, independent of environmental temperature Ectotherms Ectotherms are often the same temperature as their environment, because of their low heat production (low metabolic rate) Body temperature increases linearly with ambient temperature Metabolic rate increases exponentially with temperature Q10 ~2.5 effect) AQUATIC ENVIRONMENT Aquatic ectotherms have the same temperature as their environment due to: low heat production high thermal conductivity of water Downloaded by Harshdeep Kaur ([email protected]) lOMoARcPSD|40737841 Daria Harris But, aquatic ectotherms may thermoregulate behaviourally by entering/exiting water at different temperatures Requires water with different temperatures Thermoclines in still water Terrestrial Environments Terrestrial ectotherms often have different temperature as their environment; they thermoregulate behaviourally more, due to: lower thermal conductivity of air more variation in environmental temperatures solar radiation & differential heating Heliotherms bask in sun Thigmotherms press body to warm rocks or soil Arctic midges perch in parabolic-shaped flowers, sunlight focused on body (heliothermy) Dragonflies changes body posture to maximise or minimise heat gain (heliothermy) Snakes bask in sunlight, hood/body flattened, in early morning (heliothermy), avoids light in middle of day, lies of rocks in afternoon (thigmothermy); body temperature remains about 37°C Endotherms Endotherms use internal heat production to regulate a high and constant body temperature; generally 36 to 42°C Independent evolution: Mammals Birds Possibly pterosaurs and some dinosaurs Basic pattern is body temperature regulated high (36 to 42°C) & constant, over wide range of ambient environmental temperatures Metabolic rate (= heat production): Increases at low temperatures to match heat loss Constant in thermoneutral zone Increases at high temperatures due to cost of heat loss HEAT CONSERVATION First step in endothermy is heat conservation Fur and feathers are insulation to prevent metabolic heat loss DIANASAURS Some small dinosaurs (ancestors of birds) would have been true endotherms They had feathers for insulation e.g. velociraptors Gigantotherms (dino) Many large reptiles were ‘gigantotherms’ Large animals have a low surface area : volume ratio So reduced heat loss Large reptiles have higher body temperatures (e.g ≈ 2°C for sea turtles) from internal heat production Downloaded by Harshdeep Kaur ([email protected]) lOMoARcPSD|40737841 Daria Harris Very large dinosaurs would have had high body temperatures Indeed, they might have had problems losing excess internal heat! HIBERNATION Hibernating mammals; e.g. bears, squirrels, hedgehogs Drop internal temperature to save metabolic energy Driven by low food availability INSECTS Dung beetles are endothermic while they are walking e.g. rolling their dung balls Many insects are Endothermic when active Ectothermic when resting Night-flying moths: Eupsilia is endothermic, a ‘hot’ flyer with a ‘furry’ thorax (contains flight muscles) >30°C; even at freezing air temperatures Operophtera is ectothermic, a ‘cold’ flyer, with adaptations to fly (low wing load) at the same low temperatures (ligher males only) REPTILES Brooding female pythons Incubate their eggs Shiver to produce metabolic heat Adult tegu lizards during breeding season Raise metabolic heat Constrict peripheral blood vessels Active at night, protect territories FISHES Regional Heterothermy Mako sharks, tuna and swordfish Partially endothermic Use metabolic heat to warm part of their body Usually warm muscles, but also brain and eyes Counter current blood flow retains heat Large animals with a low surface areato-volume ratio reduce heat loss Environmental Challenges Metabolic depression in extremes Seasonal acclimatisation Cold (peripheral hypothermia) Cold (torpor, hibernation) Freezing cold Heat (avoid, evaporation) Hypoxia (burrowing, altitude, diving) Climate Change Metabolic depression in extremes Downloaded by Harshdeep Kaur ([email protected]) lOMoARcPSD|40737841 Daria Harris Seasonal acclimatisation Cold Peripheral heterothermy, counter-current heat exchange Downloaded by Harshdeep Kaur ([email protected]) lOMoARcPSD|40737841 Daria Harris Torpor, hibernation Diurnal, seasonal Freezing Cold Supercool: Pure water freeze at 0°C Body fluids freeze at -0.7°C Seawater freeze at -1.86°C BUT polar fishes supercool to -2°C Hibernating Arctic ground squirrels supercool to -3°C Many insects supercool to -20°C (lower!) Tissue Freezing Extracellular fluids can freeze Intracellular fluids must not freeze Wood frog Inter-tidal molluscs Heat Avoid Get off the ground surface! Burrow underground Move above ground Minimise contact Evaporation Water has very high specific heat Salivate Pant Sweat Gular flutter Climate change +getting hotter everywhere +rainfall: Wetter (tropics) Drier (arid zones) Predictions for ectotherms with climate change Downloaded by Harshdeep Kaur ([email protected]) lOMoARcPSD|40737841 Daria Harris Lecture 7.1 Photosynthesis, leaf structure and function What are the main functions of a leaf? Photosynthesis = capture light and assimilate carbon dioxide (CO2) Requires light-absorbing pigments and several enzymes to allow light capture and CO2 assimilation Also requires a system that allows diffusion of CO2 from the atmosphere to chloroplasts (the sites of CO2 fixation) Visualise being a CO2 molecule – Downloaded by Harshdeep Kaur ([email protected]) lOMoARcPSD|40737841 Daria Harris passing through the stomata through to the chloroplast J Go to: https://www.calacademy.or g/educators/travel-deepinside-a-leaf Overview of reactions of photosynthesis 6 CO2 + 12 H2O + photons → C6H12O6 + 6 O2 RUBISCO (RuBP carboxylase) is the enzyme that ‘fixes’ CO2 +Catalyses a reaction: 5C compound + CO2 à 2 x 3C compound +Additional reactions then use the 3C compound to make sugar (hexose = 6C) Downloaded by Harshdeep Kaur ([email protected]) lOMoARcPSD|40737841 Daria Harris overview of (eudicot) leaf anatomy 1. CO2 fixation results in a concentration gradient from air-tochloroplasts 2. Diffusion of CO2 into leaf: boundary layer resistance stomatal conductance (or resistance) 3. Once in the leaf, CO2 diffuses through: intercellular gas-filled spaces mesophyll cell wall and cytoplasm chloroplast envelope Plants leaves are covered by a ‘ waxy ’ cuticle that reduces evaporative water loss other than via stomata Cuticle on the epidermal cells of a leaf (Tabernaemontana pachysiphon) Cuticular covering of the plant body and a protective layer around the reproductive organs (e.g., seed coats) reduce water loss and the risk of desiccation. These traits are the first characteristics important in the transition of plants from water to land Downloaded by Harshdeep Kaur ([email protected]) lOMoARcPSD|40737841 Daria Harris Leaves need a system that allows the diffusion of CO2 from the atmosphere to the chloroplast Stoma form pores that can open and close at the leaf’s surface The system also needs to be able to control water loss waxy cuticle restricts water loss and the stoma close when water availability is low Stomatal pores allow entry of CO2 Water loss is inevitable during u