BIOL111 Membranes and membrane tranport 2024 PDF
Document Details
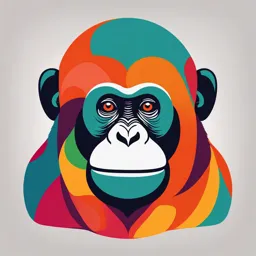
Uploaded by FearlessCello
Canterbury
2024
null
Tags
Summary
This document discusses membrane proteins, transport mechanisms, and functions in cells. It also includes information about cell adhesions and cancer metastasis by focusing on mechanical properties, adhesion, and the role of proteins like E-cadherin, N-cadherin, and β-catenin in CAF (Cancer-Associated Fibroblast)-mediated cancer cell invasion. The document presents a summary about recent research on cell biology and cancer.
Full Transcript
Membrane Proteins and Membrane Transport Chapter 5 Biology 2e pp 135 – 146 Chapter 35 Biology 2e pp 984 - 989 1 Membrane Proteins and Membrane Transport Membranes contain proteins that fulfil many different functions Me...
Membrane Proteins and Membrane Transport Chapter 5 Biology 2e pp 135 – 146 Chapter 35 Biology 2e pp 984 - 989 1 Membrane Proteins and Membrane Transport Membranes contain proteins that fulfil many different functions Membrane transport (form of work) occurs by 3 mechanisms: passive, active or secondary active Proteins are involved in these transport processes Ion channels function in nerve impulses Active transport pumps generate ion gradients that power secondary active transport Water can also move across membranes and through water channels 2 1 Membrane Proteins and Membrane Transport 3 Integral Membrane Proteins a helices span the membrane with hydrophilic residues facing the inside of the protein, and hydrophobic residues facing the interior of the lipid bilayer 4 2 Functions of membrane proteins 5 Functions of membrane proteins 6 3 Cell adhesions and cancer metastasis Europe PMC Funders Group Author Manuscript Nat Cell Biol. Author manuscript; available in PMC 2018 March 01. Published in final edited form as: Nat Cell Biol. 2017 March ; 19(3): 224–237. doi:10.1038/ncb3478. Europe PMC Funders Author Manuscripts A mechanically active heterotypic E-cadherin/N-cadherin adhesion enables fibroblasts to drive cancer cell invasion Anna Labernadie1, Takuya Kato2, Agustí Brugués1, Xavier Serra-Picamal1,3, Stefanie Derzsi2, Esther Arwert2, Anne Weston2, Victor González-Tarragó1, Alberto Elosegui- Artola1, Lorenzo Albertazzi1, Jordi Alcaraz3, Pere Roca-Cusachs1,3, Erik Sahai2,*, and Xavier Trepat1,3,4,5,* 1Institute for Bioengineering of Catalonia, Barcelona 08028, Spain 2The Francis Crick Institute, 1 Midland Road, London, NW1 1AT, UK 3Unitatde Biofísica i Bioenginyeria, Facultat de Medicina, Universitat de Barcelona, Barcelona 08036, Spain 4Institució Catalana de Recerca i Estudis Avançats (ICREA), Barcelona 08010, Spain 5Centro 7 de Investigación Biomédica en Red en Bioingeniería, Biomateriales y Nanomedicina, Barcelona 08028, Spain Abstract Cancer Associated Fibroblasts (CAFs) promote tumor invasion and metastasis. We show that CAFs exert a physical force on cancer cells that enables their collective invasion. Force Europe PMC Funders Author Manuscripts transmission is mediated by a heterophilic adhesion involving N-cadherin at the CAF membrane and E-cadherin at the cancer cell membrane. This adhesion is mechanically active; when subjected to force it triggers β-catenin recruitment and adhesion reinforcement dependent on α-catenin/ vinculin interaction. Impairment of E-cadherin/N-cadherin adhesion abrogates the ability of CAFs to guide collective cell migration and blocks cancer cell invasion. N-cadherin also mediates repolarization of the CAFs away from the cancer cells. In parallel, nectins and afadin are recruited to the cancer cell/CAF interface and CAF repolarization is afadin dependent. Heterotypic junctions between CAFs and cancer cells are observed in patient-derived material. Together, our * Corresponding authors: Xavier Trepat, PhD, ICREA Research Professor, Institute for Bioengineering of Catalonia, 08028, Barcelona, Spain, Tel: (+34) 934 020 265, [email protected]; Erik Sahai, PhD, The Francis Crick Institute, 44 Lincoln’s Inn Fields, London WC2A 3LY, United Kingdom, [email protected]. Author contributions AL, ES, and XT conceived the study and designed experiments, with additional input from TK. AL performed most experiments and data analysis. TK performed and analyzed spheroid invasion experiments and generated A431 KO cell lines. AL, AE-A, VG and PR-C designed, performed and analyzed magnetic cytometry assays. AB and XS-P developed software for image analysis and force measurements. SD performed QRT-PCR experiments. AL and LA performed STORM imaging. JA contributed CAFs from patients with non-small lung cell carcinoma. EA performed the intravital imaging and assisted the patient sample analysis, AW performed electron microscopy. AL, ES, XT wrote the manuscript with input from all authors. Code availability Computer code used in this study can be made available upon request to the corresponding authors. Data availability Data supporting the findings of the study are available from the authors on reasonable request. 8 4 9 10 5 Membrane transport: Permeability through the lipid bilayer Some things can pass freely through the lipid bilayer However many biologically important molecules cannot pass through the lipid bilayer at rates that cells require Proteins have evolved that allow cells to move these molecules/ions in a fast and controlled manner 11 Membrane Transport – basic principles 3 Mechanisms to move things across membranes Passive transport Active transport Secondary active transport 12 6 Membrane Transport – basic principles Passive transport – Molecules move down their concentration gradient (think of as moving something “downhill”) – Spontaneous – No energy input 13 Membrane Transport – basic principles Active Transport - Ions move against their concentration/charge gradients - Non spontaneous - Energy input 14 7 Membrane Transport – basic principles Secondary active transport An ion gradient (typically Na+ or H+) set up by an active transport protein is used to energise the transport of another ion/solute Similar to energy coupling 15 Passive transport Two types: – Simple diffusion Molecules move through bilayer itself – Facilitated diffusion Molecules move through transport proteins (channel/carriers) 16 8 Passive transport - osmosis Not just the solute, can be the solvent i.e. water! 17 Passive transport - osmosis High water concentration 18 9 Passive transport - osmosis Low water concentration 19 Passive transport - osmosis Passive transport – water Moves down its concentration gradient – Simple diffusion Through bilayer itself – Facilitated diffusion Through transport proteins (channels – aquaporins) 20 10 Water movements - osmoregulation Water movement – many types of cell adjust their internal solute content so they remain isotonic (i.e. have the same solute concentration) with the external media to prevent gaining or losing too much water. Osmoregulation 21 Water movements - osmoregulation In the absence of * osmoregulation – Lower solute concentration outside cell compared to inside (outside solution is hypotonic) – water flows into cell. – Cell expands 22 11 Water movements - osmoregulation In the absence of * osmoregulation – Higher solute concentration outside cell compared to inside (outside solution is hypertonic) – water flows out of cell. – Cell shrivels 23 Water movements – cells with a wall Plant/fungal/algal cells have a cell wall They are typically hypertonic to the external media Wall prevents cell from bursting Plant/fungal cells are turgid Non turgid cells – plant wilts 24 12 Facilitated diffusion – ion channels Ion channels Typically specific – only let one type of ion through. K+ channel selects K+ over Na+ at a ratio of 1000:1. Controlled (gated) e.g. voltage High turnover rates 109 ions s-1 25 Na and K ion channels – responsible for nerve impulses 26 13 Na and K ion channels – responsible for nerve impulses Opening and closing of Na and K channels leads to changes in the charge gradient across the cell membrane This is called the membrane potential 27 These changes in membrane potential constitute an action potential 28 14 The nerve signal can be passed onto other nerve cells/muscles via synaptic transmission 29 These changes in membrane potential constitute an action potential 30 15 Cystic fibrosis – a defective Cl channel Caused by a mutation to a chloride ion channel (called CFTR) 1 in 25 of us carry a mutant copy of the gene for the channel 1 in 2,500 of us have the disease Defective Cl- transport affects the airways – increased infections and gradual breakdown of lungs 31 Facilitated diffusion - carriers Carriers Typically specific Bind solute Change shape such that solute-binding site faces other side of membrane Lower turnover 103-6 ions s-1 GLUT4 32 16 Facilitated diffusion - carriers Carriers GLUT 4 moves glucose into cells. Its presence in the membrane is controlled by insulin Diabetes when no insulin produced (Type 1) or insulin receptor not active (Type 2) Thus diabetics are unable to move glucose into their cells GLUT4 33 Active transport Ions move against their concentration/charge gradients Pumps – Na+/K+ ATPase – H+ ATPase – Ca2+ ATPase Non spontaneous Energy input – ATP 34 17 Active transport generates ion gradients across membranes In an animal cell Per ATP Na+/K+ Na+ + ATPase + + Outside – 3 Na+ out plasma membrane – 2 K+ in Inside + + + K 35 Active transport generates ion gradients across membranes 2 components to the ion gradient Na+ (electrochemical + + gradient) + + + + Outside – Concentration gradient plasma membrane High Na+ outside High K+ inside + Inside – Charge gradient + More + outside + ++ K -60 mV (membrane potential) 36 18 Ion gradients drive secondary active transport glucose Na+ coupled transport Na+ proteins + + Secondary active + + + Outside + transport + Na /glucose symporter plasma membrane For example Na+ glucose symporter Inside 37 Ion gradients drive secondary active transport Na+ coupled transport proteins Secondary active + transport + + + Outside For example Na+ glucose symporter plasma membrane In your kidney this can + + move glucose against a Inside 30,000 fold concentration gradient!! 38 19 Ion gradients drive secondary active transport Na+ glucose symporter + + + Outside Symport means the ion + and the glucose move in plasma membrane the same direction + + Some secondary active Inside transport proteins act as antiporters. 39 In plants and fungi Active transporter: H+ ATPase Moves 1H+ per ATP H+ Generates + Outside – Concentration gradient + + + High H+ outside plasma membrane – Charge gradient More + outside Inside -250 mV Electrochemical gradient again used to drive secondary active transport 40 20 That’s all Folks J Thanks for your attention through these lectures I hope that they have stimulated your interest in cell biology and biochemistry Any questions don’t hesitate to email me Vanessa Morris will be here on Monday to take the final block of lectures on cell biology 41 21